The sedimentary fill of the MHB
Overview
Knowledge limits
The large thickness of the MHB strata (Fig.3), the repetitive stacking of gravity deposits and uncertainties about their lateral and vertical correlation across the basin bring about uncertainties on the definition of large-scale lithological units of stratigraphic significance (Fig. 6). Moreover, the chronostratigraphy of MHB Formations is still not very precise, mostly because of the scarcity of fossils or due to their reworking in gravity dominated facies.
Figure 6. Schematic stratigraphic logs in the central (Grevena) and southern (Meteora-Rizoma) MHB, compared to the northern one (North MHB).
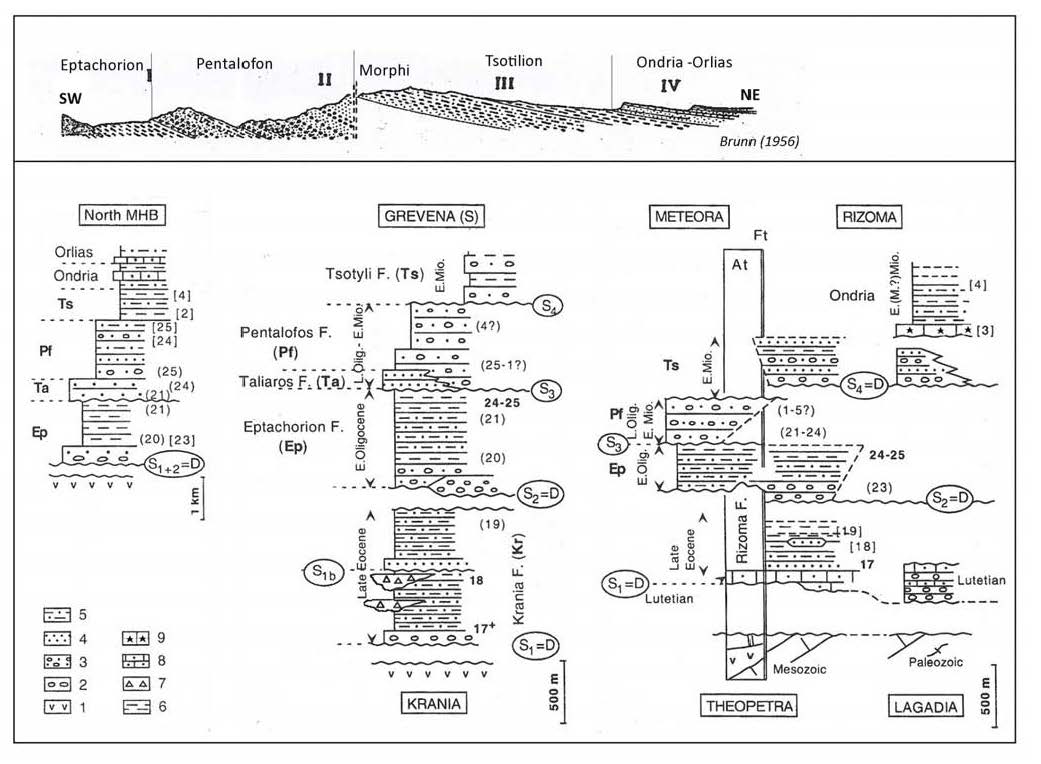
Schematic stratigraphic logs in the central (Grevena) and southern (Meteora-Rizoma) MHB, compared to the northern one (North MHB) (modified after Ferriere et al., 2004). 1: Pelagonian basement: ophiolites (v) with upper Cretaceous (limestones) on Mesozoic marbles and Paleozoic gneisses; 2: Basal conglomerates (unconformity); 3: conglomerates and sandstones; 4: Sandstones (mainly turbiditic); 5: Sandstones and shales (mainly turbiditic); 6: Shales and marls (partly hemipelagic); 7: major olistoliths (Krania); 8: Eocene detrital limestones with Nummulites; 9: Miocene Echinid-rich limestones.
Nannofossils Biozones after: Zygojiannis and Müller [1982]; Kontopoulos et al. [1994] and Zelilidis et al. [2002]; thick numbers: our own results.
Abbr: D: major angular unconformities, S: other significant Surfaces (main lithological changes), Pz: Paleozoic, TJ: Triassic-Jurassic, UK: upper Cretaceous, Eo: Eocene, Olig: Oligocene, Mio:Miocene, E: Early, M: Middle, L: Late.
Upper Cross-section: Stratotypes of the MHB Formations as defined by Brunn [1956].
The only available ages are from marls (pelagic foraminifera, nannofossils) or from a few carbonate shelf intervals (benthic foraminifera, invertebrates). Moreover, published ages are significantly divergent, even for the same faunal associations as nanofossils [Zygojiannis and Müller, 1982; Kontopoulos et al., 1999; Zelilidis et al., 2002; Ferriere et al., 2004] (Fig. 7).
The thickest, deepest and more extended Oligo-Miocene marine formations crop out in the northern part of the MHB basin. Middle-upper Eocene deposits are exposed in restricted area of the center of the basin (near Krania village; Fig. 1B and Fig. 3). These formations have their chronostratigraphical equivalents to the south of the basin, but there the facies point to shallower water depths (ex. Pentalofon Formation in the Meteora area) and therefore hiatuses and lacunas are frequent.
Figure 7. Compared ages of MHB deposits from Foraminifera (D) or nannoflora biozones.
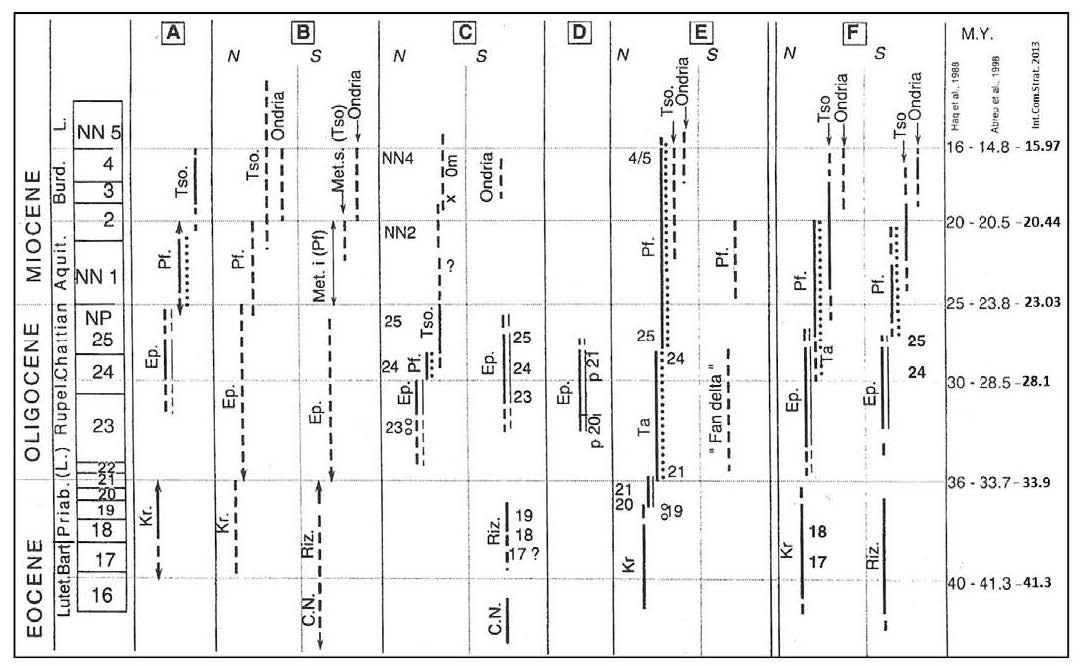
Compared ages of MHB deposits from Foraminifera (D) or nannoflora biozones (modified after Ferriere et al. 2004). A: Brunn [1956], B: Geological Maps of MHB areas, IGME, Greece, at 1:50,000 scale (cf References) and Bizon et al. 1968, C: Zygojiannis and Müller [1982], D: Barbieri [1992], E: Doutsos et al. [1994], Zelilidis et al. [1997, 2002] and Kontopoulos et al. [1999], F: this study.
Abbr. CN: nummulitic limestones, Kr.: Krania, Riz.: Rizoma, Ep.: Eptachorion, Ta.: Taliaros, Pf.: Pentalofon s.l. (Pf and Ta) or s.s. (Pf), Tso.: Tsotyli, l. and u. Met.: lower (Pf) and upper (Ts) Meteora. Single and double lines : different formations ; broken thick lines : uncertainties; nannoflora biozones 16 to 25 = NP 16 to NP 25, 17+ minimum age (biozone 17 or younger), 1 to 5 = NN1 to NN5 ; Foraminifera biozones: P20 and P21 (D). Ages of the stratigraphic stages from Haq et al [1987], Abreu et al [1998] and the International Commission on Stratigraphy [2013].
The main lithological formations and their boundaries
The Oligocene to Miocene siliciclastic deposits were first described as six main lithostratigraphic units by Brunn (1956, 1960) from the northern part of the MHB with the addition of a late Eocene Formation (Krania Fm). These Formations are (Fig.3, 4 and 6):
- i) Eptachorion Formation (about 1000m, mainly Oligocene) dominated by silty marls in the upper part of the Formation with decimetric thick very fine sandstone beds often resting on thick conglomerates in the lower part.
- ii) Taliaros (or Tsarnos) and (iii) Pentalofos Formations (2500m, latest Oligocene and early Miocene): sandstone beds coarsening upwards to conglomeratic beds; mainly conglomeratic beds in the south [Ori and Roveri, 1987; Ferriere et al., 2011].
- iv) Tsotyli Formation (600m, early-mid ? Miocene): marls interbedded with sandstones in the northern MHB; gneissic pebbles rich conglomeratic beds in the south [Savoyat et al., 1971a; 1971b; 1972a; 1972b; Zygojiannis and Muller, 1982; Ferriere et al., 2004].
- v) Ondria and (vi) Orlias Formations (350m or more, early-mid Miocene): sandstones and marls with fossiliferous limestone beds.
Most of these formations have been adopted by later workers, although minor modifications have been made. These consist namely in additional lithologic members: i) within Pentalofon Fm: Tsarnos and Kalloni members were distinguished, passing southward to Kalabaka and Anthrakia members [Zelilidis et al., 2002] and ii) in the upper part of the MHB fill, a few more formations have been added within the Ondria Fm: X Fm, Omorphoklissia Fm and Zevgostasi Fm (see geologic maps to 1:50 000, ie: Nestorion and Argos Orestikon sheets, Savoyat, 1971a, b).
The formation boundaries are either major angular unconformities or/and abrupt changes in lithology. Angular unconformity-bounded formations record major tectonic deformations. Amongst those, one may cite, in chronological order, the lower boundaries of: Krania and Rizoma Fms (Upper Eocene) ; Eptachorion Fm (Oligocene) ; Tsotyli Fm (Miocene). In the southern part of Fms the MHB, the lower Tstotyli bounding unconformity reflects a major change in depocenter location, shifting to the Rizoma area where Miocene deposits rest above Upper Eocene, Mesozoic or Paleozoic strata (Fig. 3 and 4).
Some formation boundaries are associated with major lithologic changes without basal angular unconformity (ex. Eptachorion-Taliaros-Pentalofon Fms and Ondria-Orlias Fms). In this case, the control could be an eustatic change only. This is argued by Zelilidis et al., [2002] to explain, for instance, the Eptachorion-Pentalofon Fm boundary. However, Ferrière et al., [2011] shows that even in that case the tectonic component has to be taken into account.
Facies map of the MHB
After general maps of lithological formations [Brunn, 1956; Desprairies, 1977] and detailed geological maps to 1:50 000 (maps of IGME, from 1969 to1998), lithofacies maps have been published by Kontopoulos et al., [1999] and Zelilidis et al., [2002]. These authors distinguish the marls, conglomerates, and various facies of sandstones which they relate to submarine fans and associated turbidites (Fig. 8).
Figure 8. Map of the central part of the MHB showing different sedimentary facies.
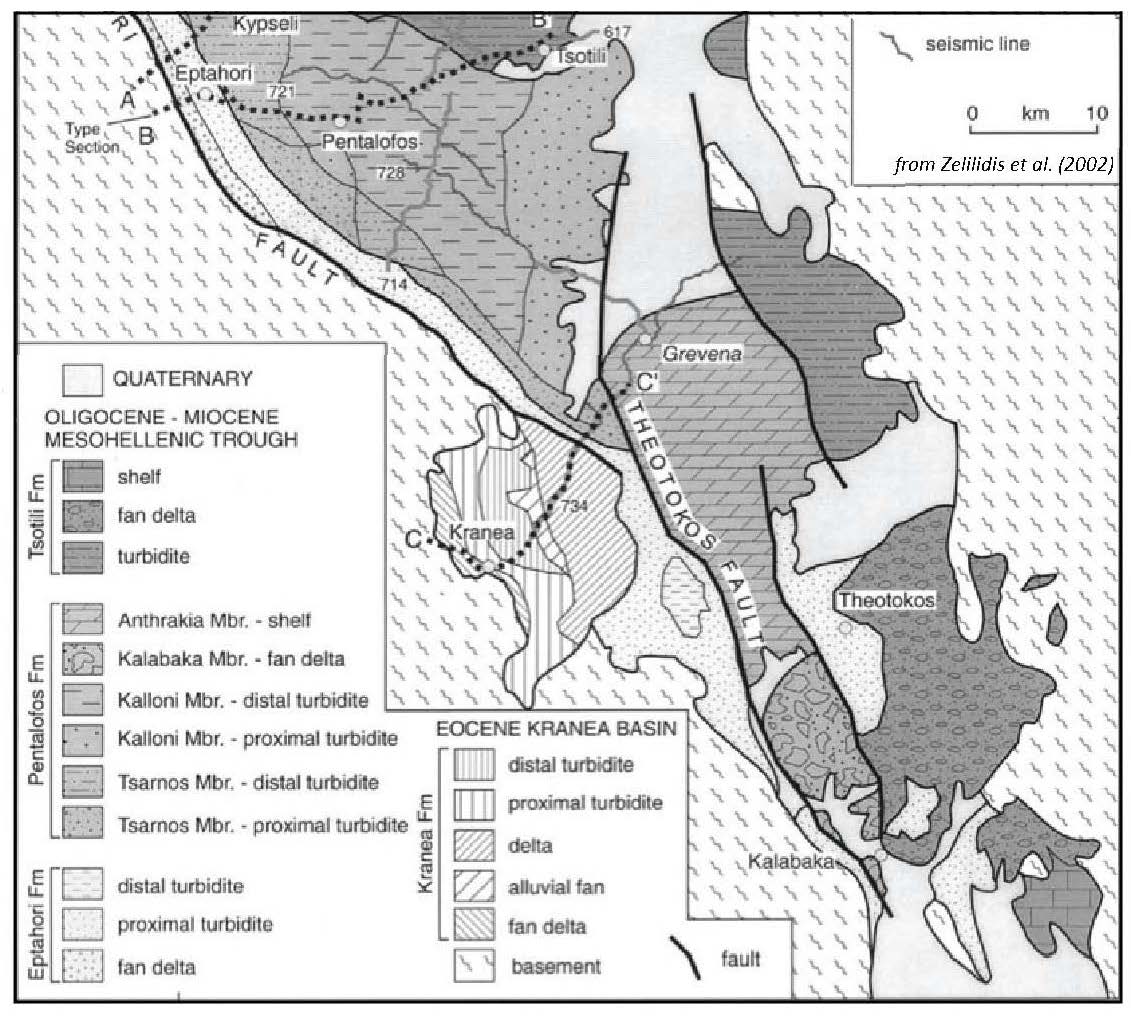
Map of the central part of the MHB showing different sedimentary facies, especially distal and proximal turbidites, as described by Zelilidis et al. [2002].
Zelilidis et al. ([2002] related these facies to subsurface depositional seismic facies and geometries. However, the likely complexity of the submarine basin topography and the lack of preservation of a complete depositional profile at the outcrop (especially for the Oligocene) make these interpretations somewhat speculative.
Besides, Ferrière et al., [2004; 2011] privileged the detailed facies mapping of selected areas in the southern half of the MHB, where unconformities are better expressed, thus providing specific arguments of the tectonic control of the depositional systems (Fig.3 ) [Ferriere et al., 2004, 2011]. The formation descriptions presented herein mostly rest above these observations (including the Krania area), but we also have added information compiled from other available sources as regarding to the area more to the north.
The upper Lutetian-upper Eocene Formations
Rizoma Fm
This formation, which rests unconformably above the Pelagonian basement, crops out to the SE of the MHB only (Fig.3 and Fig.6). It is dominated by shales with massive sandstone interbeds, locally overlying conglomerates and limestones hosting a benthic macrofossil fauna (Pl. I-B) [Savoyat et al., 1969; Zygojiannis and Muller, 1982]. The shales and sandstones have been interpreted as a fluvial-dominated shelf delta system [Ferriere et al., 1998; 2004]. This formation is covered by Oligocene basal conglomerates or Miocene conglomeratic Tsotyli beds (Fig.3).
The Rizoma Fm comprises three lithological units, from base to top (Fig.6):
- i) Well-rounded basal conglomerates. These conglomerates are exposed in two areas, to the south of Vassiliki and more to the east near Lagadia (Fig.3 and Fig.6). They deliver numerous clasts of Cretaceous limestone, as well as radiolarites, ophiolites and triassic-jurassic marbles, all derived from the internal zones which crop out in vicinity to the east.
- ii) Nummulitic-rich limestones, pointing to a carbonate shelf setting (Pl.I-B). They also contain algae and Echinids, and are attributed to the Upper Lutetian [Savoyat et al., 1969; 1972a; Ardaens, 1978; Ferriere 1982].
- iii) The “Rizoma marls”, a thick shale succession (more than 200m) made up of distal turbiditic sequences, locally with metric sandstone beds interpreted as fluvial dominated deltaic mouth bar systems (wood fragments, floating mud pebbles, water escape features, current ripples, Skolithos traces and various burrows). This deposit is supplied by the pre-ophiolitic basement comprising schists and gneisses. Globigerinids [Bizon et al., 1968; Savoyat et al., 1969; 1972a] and calcareous nannofossils [Zygojiannis and Muller, 1982; Ferriere et al., 2004] from the Rizoma calcareous marls yielded an Upper Eocene age (biozones 17 to 19) (Fig.6 and Fig.7).
Krania Fm
The Krania Formation is well developed and preserved only inside a syn-sedimentary syncline. This formation is exposed in the western part of the MHB, to the SW of Grevena (Fig.3). It forms a flysch-like unit 1500 m thick, bounded at the base by ophiolitic conglomerates and overlying mostly the ophiolitic basement of the basin. It is bounded to the top by the major intrabasinal unconformity of the MHB. A minor unconformity has been described inside the formation [Koumantakis and Matarangas, 1980; Wilson, 1993; Ferriere et al., 2004]. The Krania Fm provided a foraminifera and nannofossil assemblage of the upper Lutetian-Upper Eocene (Fig.6 and Fig.7).
The Krania Formation exhibits a set of two sequences of deposits (Fig.6):
Lower Krania sequence
West of Krania, the deposits rest onto roughly bedded, polygenic clast-supported conglomerate beds, interpreted as alluvial fan deposits, onlapping a sole of ophiolitic epiclastites. Above these basal beds in the Krania-Microlivadon areas, the lower sequence is composed of fine-grained fining upwards and homogeneous sandstone beds interpreted as deep water and ophiolitic rich turbidites (Pl. I-C).
On the northern side of the syncline, this basal succession passes laterally to highly bioturbated, fine grained marly sandstones with thin channel bodies of sandstones showing mud pebbles at the base, interpreted as bay-fill deposition and to roughly bedded conglomerates interpreted as alluvial fans (Trikomo-Parorio area, cf Fig.14). Nannofossils in these facies yielded an Upper Eocene age (Biozones 17 and 18, Fig.6 and Fig.7) [Ferriere et al., 2004].
These deposits may be highly disrupted by slumps and locally by a large scale olistostrome (Trikomo-Monachiti area, Pl. I-D) especially at the end of deposition of the lower sequence. This olistostrome, mainly composed of Cretaceous limestones olistolites collapsed from the northern basin margin, feeds channels and gullies down to the southern basin floor (Krania and Microlivadon area ) [Wilson, 1993; Papanikolaou et al., 1988; Ferriere et al., 2004]. It is coeval to a paroxysm of slumping in the deep turbiditic basin. Middle to Upper Eocene Nummulitids are found in the matrix of the olistostromes ([Ferriere et al., 1998; 2004].
Near Mylia (6 km SW of Krania, Fig.4B), flysch-like deposits deliver clasts and blocks of lavas or Lutetian and upper Eocene limestones. For some authors, these deposits belong to the top of the Pindos flysch units, which would be exposed as a tectonic window beneath the ophiolitic basement of the MHB [Desprairies, 1979]. We rather suggest they form the lowermost deposits of the MHB, preserved in small grabens at the top of the ophiolites (Fig.4B) [Ferriere et al., 2004]. This is of importance for determining the age of the main stage of deformation in the Pindos zone.
Upper Krania sequence (shales and sandy turbidites)
This unit unconformably rests above the lower sequence. The unconformity is well exposed on the northern side of the Krania syncline (Trikomo-Monachiti area), while it is less prominent southward, in the center of the syncline (cf Fig. 14). The upper Krania sequence is made up of the same turbiditic sandstones as the lower one, but exhibits at the base a sharp-based hectometric succession of thicker beds with locally abundant burrows (Skolithos), plant fragments, water escape structures and intraclastic breccias, interpreted as part of a basin floor fan (south of Monachiti) (Pl. I-D).
The Krania basin deposits are topped by the Oligocene major unconformity locally made up of reddish conglomerates and paleosoils truncating the turbidites in the middle of this basin (Fig. 3 and cf Fig.14).
Interpretation: transgression above a post-tectonic, irregular paleotopography
The late-Eocene formations of the MHB (Rizoma and Krania) record the transgression which takes place after the main Cenozoic tectonic phase of the internal zones of the Hellenides. The paleotopography was still scarped and locally active, which explains the development of olistolites (submarine slope mass wasting) and conglomerates. The first transgressive deposits are rapidly buried beneath sandy, deep-water, flysch-like turbidites to the west (Krania), while a thin calcareous shelf persists to the east (Rizoma), finally drowned by a siliciclastic shelf delta. This reflects the irregular topography on which transgression takes place, perhaps the stronger subsidence to the west, closer to the foredeep of the Hellenic chain, and likely the influence in the Rizoma area of the uplifted Pelagonian Indentor (cf infra, PI, Fig.1 and cf Fig.13) [Ferriere et al., 2004].
The Oligocene Eptachorion Formation
Overview
By contrast to Eocene formations, the Eptachorion Fm is ubiquitous throughout the MHB. It forms the lower part of the main Oligo-Miocene, NW-SE trending “Albano-Thessalian” basin. It is exposed mostly to the western border of the basin, while it is buried beneath Miocene formations to the east (Fig.3). To the south, the Theotokos-Theopetra anticline (TTS, Fig.3 and Fig.4) allows part of this formation to be exposed in the center of the MHB.
The facies are mostly marine siliciclastics but limestones are locally present at the base of the formation. The age of the formation is based on datation of benthic foraminifera (at the base), and calcareous nannofossils and planktic foraminifera (at the top). An Oligocene age is largely admitted but the time range varies from one author to another (Fig.7 and infra).
Facies of the base
Limestones
Where the Oligocene transgression comes onto hard substrates, namely Mesozoic limestones, shallow-water carbonate reefs develop, and they might there replace the basal conglomerates of the Eptachorion formation. This is what can be observed to the north of Alatopetra (Fig.3), where the reefs were formed by scleractinian corals associated to benthic foraminifera (Lepidocyclins).
These facies are in turn rapidly buried by fine-grained turbidites. This is also the case to the southernmost part of the MHB: (i) near Mitropoli (western border), where Oligocene limestones overlie the Mesozoic limestones of the Koziakas range [Savoyat et al., 1969b; Lekkas, 1988; Ferriere et al., 2011]; (ii) near Farkadon (eastern border) where the limestones are not followed by a siliciclastic series. At least for the first instance, the limestones are dated from the Uppermost Oligocene [Lekkas, 1988; Ditbanjong, 2013].
Conglomerates
Elsewhere (and in most places), the lower part of the Oligocene series is commonly composed of conglomerates, which record the major tectonic phase of the Eocene/Oligocene boundary. These conglomerates may reach 1,5 km in thickness, as interpreted from seismic profiles [Kontopoulos et al., 1999; Zelilidis et al., 2002]. However, they have not been studied in detail at the outcrop.
The clast lithology of these conglomerates reflect the bedrock lithology of the basement highs exposed in the vicinity of the outcrops. Desprairies [1979] shows that they consist of mostly ophiolitic clasts derived from the Pindos area (to the west of the basin), but this may be biased by the fact that most of his studied samples come from the western border of the MHB. In Avra (to the east of Kalambaka, Fig.4D), the conglomerate clasts are mostly composed of gneisses derived from the Pelagonian zone (to the east of the basin).
From turbidites to shelf marls
The facies of the base are overlain by sandstones and shales interpreted as turbidites (areas of Zouzouli, Alatopetra or SE Krania). These deposits largely overlap the facies of the base and also overlie the Eocene formations (near Parorio-Trikomo) or even the basin basement (Cretaceous limestones to the north of Theopetra) [Ferriere et al., 1998; 2004].
These dominantly silicic clastic deposits show an upward increase in carbonates, finally passing to marls, which is the most typical facies of the Eptachorion formation throughout the MHB. These marls contain locally pelagic foraminifera, small bivalves and floated wood fragments (Pl. I-F and I-G).
Near the Meteora, the Eptachorion deposits beneath the Lower Meteora Conglomerates are a little bit different. The marls are underlain by decametre-thick successions of alternating silty marls and cross-stratified sandstone beds, with locally preserved HCS, many trace fossils (planolites) and mud clasts (Pl. I-E). The marls contain large benthic foraminifera [Savoyat et al., 1972a] molluscs (gastropods, pectinids, oysters) and plant remnants. The marls and sandstone deposits point to a shallow water coastal or bay setting.
The Oligocene age of the Eptachorion marls was early determined owing to mollusks, large benthic (Lepidocyclins) or pelagic (Globigerinids) foraminifera, or calcareous nannofossils [Brunn, 1956; Savoyat et al., 1972b; Zygojiannis and Muller, 1982; Barbieri, 1992; Kontopoulos et al., 1999; Ferriere et al., 2004]. However, while ages based on Globigerinids (biozones 20-21) might be well established [Barbieri, 1992], those based on nannofossils vary from one author to another [Zygojiannis and Muller, 1982; Kontopoulos et al., 1999; Zelilidis et al., 2002; Ferriere et al., 2004] (see Fig.7).
Interpretation : rapid and widespread subsidence of the MHB
The Oligocene Eptachorion Formation corresponds to a transgressive sequence. The Eptachorion marls point to an offshore setting, at distance from terrigenous supply, by contrast to the underlying conglomerates and turbidites. But this facies shift might also be (partly) due to a progressive levelling of the post-tectonic landscapes surrounding the basin.
There are also significant lateral variations of the water depth associated to the upper, finer- grained part of the formation. The Eptachorion marls are interpreted as outer shelf to bathyal deposits based on their microfaunal content, mainly nanofossils and Globigerinids, which might indicate a water depth up to 600 m [Barbieri, 1992]. To the south, near the Meteora area, the interbedded marls and sandstones indicate a more proximal, lower shoreface to upper offshore/bay setting sporadically supplied by sediments derived from the coast.
Whatever, considering the shallow water of the shelf limestones of the base of the Oligocene series, eustatic changes cannot be the only cause for the recorded transgression (a rise up to 600m). It implies a strong subsidence, the causes of which are not determined. The nature of the crust beneath the MHB, which is locally loaded by thick ophiolitic nappes (Vourinos to North- Pindos section), may control subsidence variations based on density and rheology contrasts. Also, processes related to the underthrusting of external zones during the Oligocene could enhance these inherited heterogeneities (i.e. tectonic erosion, for instance; cf. infra, geodynamical setting).
The Upper Oligocene (?) - Lowermost Miocene Tsarnos - Pentalofon Formation
Overview
The Pentalofon Formation is attributed to the lowermost Miocene and possibly to the uppermost Oligocene (cf. infra). It is composed of marine conglomerates and sandstones and records an increase in energy of the sedimentary processes as compared to the Oligocene. This is the response to the uplift of the eastern border of the MHB.
One major characteristics of this formation is a sediment source fully localized in the internal zones (Pelagonian basement), to the east of the basin, which supply mostly Paleozoic gneisses and Triassic marbles to the deposit. This eastern feeder was already active in the Oligocene (cf. Avra, Fig. 4 and Fig. 16B), but subdued by comparison to the Pindos zone (to the west), which was then probably higher and supplied a more weatherable and erodible material (ophiolites and flysch).
The lower boundary of the Pentalofon Formation is a sharp lithologic contact well expressed to the south of the MHB, where the Lower Meteora conglomerates overlie the Eptachorion marls (Fig. 6). To the north, the Pentalofon Fm is finer grained. Its lower part consists of the Taliaros Fm or Tsarnos Fm of Brunn [1960], Kontopoulos et al., [1999] and Zelilidis et al.. [2002]. Based on seismic profiles, Zelilidis et al., [2002] evidence within the Pentalofon Fm a lower member (Tasrnos) and an upper member (Kalloni). These members would correspond, to the south of the MHB, to the Kalabaka and Anthrakia members respectively (Fig.8).
The lower part: Taliaros or Tsarnos formation
This formation does not exist to the south of the MHB (Fig. 3). It is composed of sandstones, marls and scaphopod - and ahermatypical coral-rich, gravelly limestones [Brunn, Pentalofon map, 1960]. The sandstones are well bedded (to the north of Alatopetra for instance). They are interpreted as submarine inner and outer fan deposits [Kontopoulos et al., 1999] or distal to proximal turbidites based on their sandstone-shale ratio [Zelilidis et al., 2002]. Near Eptachorion village, mass-wasting features are preserved in these facies (slumps) (Pl. I-G).
The main part: Pentalofon Formation
Overview
The main Pentalofon Fm is coarser-grained and consists of materials derived from the Pelagonian basement mainly [Brunn, 1956]. It records facies variations from north to south [Desprairies, 1979; Zelilidis et al., 2002; Ferriere et al., 2004 and 2011].
To the north, it forms the main reliefs of the MHB landscapes. There, it is 2-4 km thick (Kalloni Fm, as defined from seismic profiles by Zelilidis et al. [2002]).
The mainly terrigenous deposits of the Pentalofon Fm, range from well bedded conglomerates to marly shales. These facies commonly form thickening and coarsening upward successions, first interpreted as shelf deltas [Desprairies, 1979], and then associated to deep-sea fan turbidites [Kontopoulos et al., 1999; Zelilidis et al., 2002] (Pl. I-H). Such regular bedded alternating sandstones and siltstones also exist on the southernmost western border of the MHB, near Mitropoli (Fig.1B and Pl. IIA) where they cover thick conglomerates.
In its southeastern part the Pentalofon Fm is coarser grained and has been interpreted as fan- and shelf deltas [Kontopoulos et al., 1999; Zelilidis et al., 2002], namely the famous Gilbert deltas of the Meteora area [Ori and Roveri, 1987].
The Lower Meteora Conglomerates (LMC)
The LMC rest by a locally channelized erosional contact above dominantly sandy-silty marine deposits of the Oligocene Eptachorion Formation (Fig.6). The LMC, emplaced at the narrowest part of the MHB, have been described in detail by Ori and Roveri [1987]. It is composed of homogeneous, matrix to clast-supported well rounded conglomerates (Pl. II-B and II-C). The clast lithology is dominated by Paleozoic gneisses and Triassic marbles that point to a source located NE of the Meteora area, in the Pelagonian domain (Pl. II-D and II-E). The ophiolitic pebbles are generally not abundant. It is worth noting the absence within the LMC of clasts of the lithologies originated from the Koziakas ranges, which form the basin basement on the western side of the LMC (Fig.3).
Figure Plate II. Facies of the upper part of the MHB fill
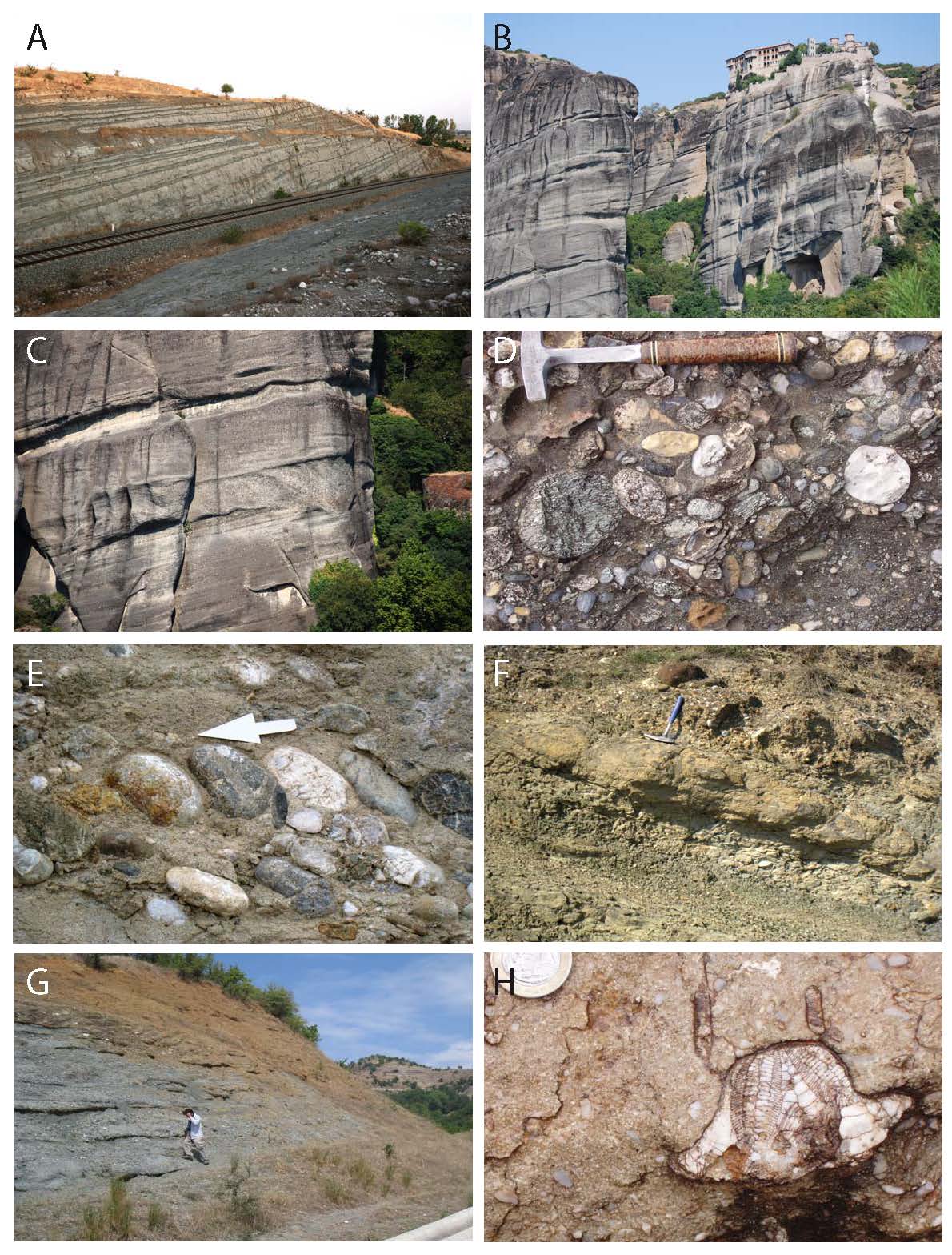
A) Early Miocene (Pentalofon Fm) to the north of Mitropoli (Southern MHB). Alternating upward-fining sandstone and siltstones. B) Pentalofon Fm: view of the Meteora Gilbert deltas (southern MHB), showing the typical westward dipping progradational wedges. C) Pentalofon Fm, Meteora Gilbert deltas (south MHB). Cliff view showing grain-size variations. The channel is of decametric scale. D) Pentalofon Fm. Conglomerate facies. The pebbles are composed of Triassic and Jurassic white marbles and Pelagonian Paleozoic gneisses, with locally minor ophiolitic clasts. E) Pentalofon Fm: imbricated pebbles showing a paleocurrent to the SW in the conglomeratic deposits. This direction is about the same for all the conglomerates of the Pentalofon Fm in the southern MHB. F) Tsotyli Fm. A few km to the north of Meteora, near Asproklissia, sandstones and silstones with interbedded conglomerate lenses. These facies occur between thick conglomerate successions not seen on the photography. G) Tsotyli Fm (early Miocene): sandstones and conglomerates with numerous schist and gneiss clasts (east of Asproklissia). H) Ondria Fm (early-mid Miocene): Clypeaster-rich limestones
The LMC are basically formed by the stacking of wedge-like units composed of clinoforms over 10° dip, which were interpreted as Gilbert-type, fan deltas prograding toward the basin axis in about 30 m water depth (Fig. 9). The feet of the deltas are overscoured by up to 30 m deep channels indicating strong currents constricted in the axis of the basin. The basin might have been overfilled by these deltas, as the same conglomerates are preserved on its other side near Mitropoli and Koziakas mountains. The wedges would correspond to highstand deposits, while the channels would be formed and backfilled during subsequent lowstand and rise of sea level. The maximum thickness of the wedge stack is about 300 m at the outcrop. The stacking pattern shows an upward decrease of the slope dip of the topsets. This points to a tectonic tilt and uplift of the hinterland, the western border of the Pelagonian Indentor (cf. infra) (Ferriere et al., 2011).
Figure 9. Meteora Gilbert delta
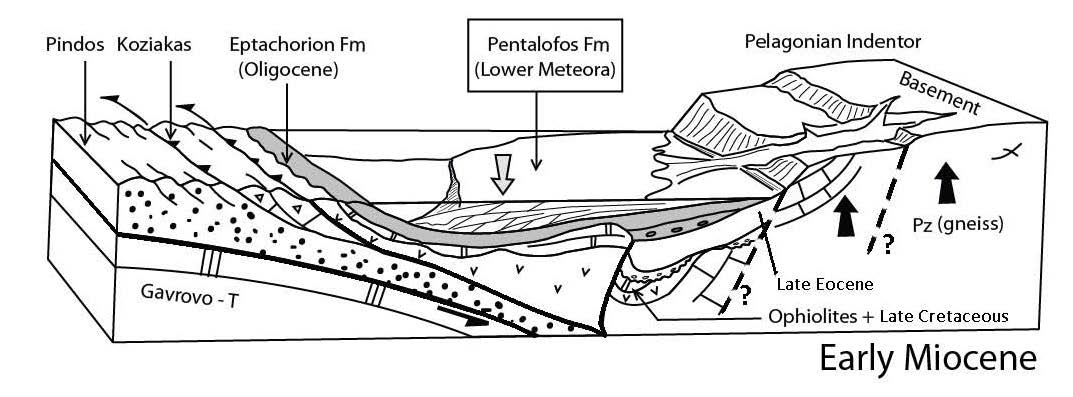
Meteora Gilbert delta (early Miocene): Schematic environment showing uplift in the eastern area (modified after Ferriere et al., 2011). Normal faults are hypothetical faults as they have not been proven in the field.
Age of the Pentalofon Formation
Where it is dominated by conglomerates, the Pentalofon Fm could not be dated by biostratigraphy. Elsewhere, there is a range in the published ages, depending on the area and sub-formation considered (Fig.7).
Brunn [1956] observed scleractinian corals within the Tsarnos-Taliaros Fm which he considers Upper Oligocene in age, as well as Miogypsina complanata SCHLUMB in the Pentalofon s.s. Fm which he attributes to the Aquitanian. Later datations by nannofossils confirmed this age [Zygojiannis and Muller, 1982 ; Zelilidis et al., 2002 ; Ferriere et al., 2004]. However, Late Rupelian - Chattian biozones were locally identified: NP 24 in Tsarnos member, and NP 25 in Kalloni member [Zelilidis et al., 2002]. This somewhat older age of the Pentalofon Fm was also adopted by Kontopoulos et al. [1999]. Nevertheless, the large time span of this formation raises issues as regarding to its correlation 3rd order cycles of eustatic charts.
Interpretation: raise of Pelagonian supply and rapid infilling
The deposits of the Pentalofon Fm are mainly coarse-grained detrital sediments (sandstones and conglomerates) deeper in the northern part of the MHB than in its southern one. While the main source of the Oligocene Eptachorion Fm came from the western side of the MHB, the detrital sediments of the Pentalofon Fm are feeded from the uplifted eastern side of the MHB (cf Pl. II-E). The different fan-deltas, notably the Gilbert delta outcropping in the Meteora area, fill the MHB and give rise to a regressive event.
The Lower Miocene (p.p.) Tsotyli Formation
Overview
This formation is heterogeneous, ranging from continental, coarse-grained deposits to the south, to fine-grained marine facies to the north, where it was first defined as the ‘Tsotyli marls’ by Brunn [1956], who aged it, based on nannofossils, to the late Aquitanian-Burdigalian (Fig.7).
To the south, in the Meteora area, it consists of mostly very coarse conglomerates alternating with poorly bedded sandstones and was there mapped as the “Upper Meteora Conglomerates” (UMC) [Savoyat et al., Kalabaka sheet, 1972a]. There, the relative amount of clasts derived from the older Pelagonian basement (mostly pre-Triassic gneisses) increases as compared to the underlying Pentalofon Fm.
These various facies are gathered into a unique formation defined as: (i) a separate, distinct depocenter on the eastern side of the MHB ; (ii) a set of deposits bounded at the base by the same, major unconformity which is well expressed in the Meteora area (angular unconformity of about 20° between the LMC and the UMC).
This depocenter has the shape of a syncline parallel to the strike of the MHB (Fig.3). The Tsotyli strata inside this syncline show an eastward offlaping, which indicates the progressive eastward displacement of accommodation and, therefore, subsidence (Fig.4). To the south of the MHB, the western side of this syncline is an old structural high, the "Theopetra-Theotokos Structure" (TTS, cf. infra and Fig.4). On the eastern flank of the syncline, the upper units of the UMC are overlying Rizoma Eocene deposits or the basement of the MHB (Fig.3 and Fig.4).
The Tsotyli deposits
The Tsotyli marls, the thickness of which is up to 1 km to the north, thin southward where they are interfingered with more sandstones and conglomerates.
To the north, the Tsotyli deposits have been first interpreted as shelf marls with some interfingering sandstones interpreted as fluvial-dominated shelf deltas [Brunn ,1956; Desprairies,1979]. More recently, they have been reinterpreted as turbidites [Kontopoulos et al., 1999] passing upward to fan-deltas [Zelilidis et al., 2002].
To the south, from Aliakmon river to the Meteora area (Asproklissia area, Fig.3), the Tsotyli deposits form a transgressive-regressive succession comprising from base to top [Ferrière et al., 2004]: (i) conglomerates and channelized fluvial sandstones with paleocurrents to the WSW (Upper Meteora Conglomerates, UMC); (ii) fine-grained and locally carbonate-rich shelf sandstones and siltstones (Pl. II-F); (iii) conglomeratic fans-deltas similar to the LMC; (iv) grey or reddish conglomerates (mostly preserved on the eastern basin border) (Pl. II-G).
Further southward, to the SE of the Meteora area, pebbles of pre-Triassic Pelagonian gneisses constitute the whole deposit.
The clast lithology within the Tsotyli Fm reflects the space and time evolution of the feeders which supplied sediments to the eastern border of the MHB: (i) in space, one notes the predominance of ophiolitic clasts to the north, which is related to the vicinity of the Vourinos mounts, while marbles and gneisses are more abundant toward the south where exists the Pelagonian Indentor made up of these dominant lithologies ; (ii) in time, there is an overall increase in the amount of gneisses and marbles, as the sediment source was shifted eastward into the Pelagonian hinterland, where the rejuvenating relief supplied progressively deeper rocks from the basement to the basin.
Interpretation: shift of subsidence to the east
The deposits of the Tsotyli Formation show that the MHB was deeper in its northern part as for the Pentalofon ones. The main difference between these two Formations is the major eastward migration of the depocenter and of the uplifts at the origin of the detrital material. In the southern part of the MHB (Meteora area) the Tsotyli basin is separated from the Pentalofon one by a high structural element (the TTS structure, Fig.4).
The Lower to Middle (p.p) Miocene Ondria and Orlias formations
Overview
In the Greek part of the MHB, the youngest deposits are preserved as two distinct formations at the two ends of the basin. Their lithology is not homogeneous but both have a significantly higher amount of carbonates compared to the previous ones. These two formations were initially defined in the northern part of the MHB by Brunn [1956]: The Ondria Fm is composed of alternating sandstones, limestones and marls, while the uppermost Orlias Fm, which cover a more restricted area, is composed of sandstones and bioclastic carbonates. To the south of the MHB, only the time equivalent of the Ondria Fm is exposed.
The Ondria Formation
To the north of the MHB, the Ondria Fm is well exposed. The fauna delivered by the marls provided a Burdigalian age (Fig. 7).
On the 1 :50,000 geological maps (e.g. Nestorion sheet [Savoyat et al., 1971a]), the Ondria Fm comprises several formations of higher order based on lithology: the X Fm (mostly marls and limestones, among which carbonate buildups); the Omorfoklissia Fm (sands and sandstones with interbedded Globigerinid-rich marls); and Zevgostation Fm (also alternating sands and sandstones with marly intervals).
It typically starts with marls or limestones resting above an unconformity which is more obvious to the south of the basin. Thus, near Ellinokasto-Lagadia (ca 11km east of Vassiliki, Fig.3), Echinid (Clypeaster)-rich limestones are onlapping toward the east above the basin basement. In this area, the limestones are covered by sandy turbidites which pass upward to shelf marls (cf. Fig.16B ; Pl. II-H and Pl. III-A).
The Orlias Formation
This formation occupies a restricted area. It is attributed to the Helvetian (Langhian-Serravalian p. p. ; cf. Fig.7), and comprises sandy marls, sandstones and bioclastic carbonates, mostly composed of green algae, echinids and mollusks (Ostrea cf Crassissima notamment).
Owing to the fact that they were emplaced in very shallow water depths (green algae), these deposits might correspond to the final infilling of the last marine area within the MHB.
Interpretation: final marine stage
The Ondria Fm is interpreted as a transgressive-regressive sequence. The transgressive, lower part is typically dominated by shallow-water carbonates, implying a minimum input of siliciclastics (maximum retreat of littoral sources), while the regressive, upper part of the formation records the influence of the return of these sources.
The two small areas where these formations are preserved are thought to correspond to the last marine areas of the MHB at the end of its infilling. This interpretation is supported by the fact that the strata in these formations are not much deformed (nearly horizontal) and that the facies indicate a very shallow water depth (final infilling of accommodation space).
The absence of either an erosional unconformity or conformable continental regressive strata above these formations indicate that the surrounding reliefs were eroded at the final marine stage. This is indicated by the progressive replacement of siliciclastic sediments by carbonates.
Synthesis of stratigraphic data
Overview
The sedimentary fill of the MHB, up to 4.5 km thick (Fig.3), is almost composed of siliciclastic deposits. The gravity processes dominate, as indicated by the abundance of turbidites, olistostromes, and Gilbert deltas. This depositional regime indicates an important submarine relief at the basin margins and a significant erosion in the catchments areas. It ceases for a long time only during the emplacement of the Eptachorion marls (upper Oligocene) and at the final stage of basin infilling when it is replaced by shallow-water carbonate systems (middle Miocene Ondria and Orlias Fms). The only other places where carbonates are preserved are the basin borders during major transgressions (lower Oligocene at Alatopetra, upper Oligocene at Mitropoli; Figs. 1 and 3).
The formations are organized in 5 major transgressive-regressive stratigraphic sequences, which are bounded by angular unconformities or major and abrupt facies changes (Fig.6). In most instances, even where the angular unconformity cannot be easily evidenced, the large amplitude of relative sea-level change across the boundary exceeds that of sea-level cycles as reported on eustatic charts. Therefore, the major stratigraphic sequences are controlled by tectonic deformation.
Overall evolution of water depth
The submarine, gravity flow-related facies are finer-grained moving toward the north, which is interpreted as the result of a deepening of the basin. This applies to all periods of the infilling. The deepest facies of each major stratigraphic sequence correspond to the fine-grained deposits. The Krania “flysch”sub-basin might be the deepest one, at the onset of the MHB (over 1500 m?). Water depths about 600 m are proposed by Barbieri [1982] for the Eptachorion marls. No specific water depth was determined for the Miocene sub-basins, but the submarine fan terminology used by Zelilidis et al. [2002] relates to water depths in excess of those prevailing for the shelf-to-slope range (i.e. in excess of 200 m). Thus, water depth overall decreases in the course of the basin infilling, independently from the tectonic changes which drive the major stratigraphic sequences.
This probably reflects the larger time- and space scale of formation of the Hellenic accretionary prism.
Evolution of depositional slopes
Comparison between the depositional settings of the most distal facies recorded in each major stratigraphic sequence helps to reconstruct the overall evolution of the submarine topography and infilling of the basin.
The maximum slope of the depositional system is recorded in Eocene sandstones of Krania, which also correspond to the deepest setting (common occurrence of Zoophycos traces).
The Oligocene Eptachorion marls progressively point to a lesser slope or even the distal part of a shallower basin.
The return of a steep slope, with high energy gravity deposits, is marked in the Late Oligocene Pentalofon slope fans and deltas, which recedes again within the Lower Miocene Tsotyli marls in the northern MHB. The deepest parts of the ancient formations are not at the same place than that of the youngest ones as the depocenters migrate to the east and the origin of the main part of the detrital sediments change from the W to the E of the MHB.
The basin profile achieved during the Ondria and Orlias Fms is almost flat, allowing the development of carbonate shelves even covered by terrigenous beds as in the south.
Throughout the entire basin evolution (at the exception of the latest carbonates), nevertheless, even the most distal and finest grained deposits still exhibit hints of turbidites, which suggests a likely connection at the coast with canyons or deltas. Also, the basin borders might host mostly conglomerates (alluvial fans or fan-deltas), which testifies the occurrence of neighboring steep reliefs (above sea level). A modern setting analogue could be the Gulf of Corinth, although the tectonic context is not the same.
Eastward migration of sediment sources
The clast petrography and mineralogy of the MHB deposits designate the proximal borders of the basin as the sources for sediment supply.
The Eocene Krania deposits have a high ophiolitic content. Ophiolites constitute the bulk of the basin basement and also most of the mountains that bordered the former Krania basin to the west. To the east, by contrast, Late Eocene Rizoma quartz and mica-rich deposits are not resting on ophiolites but on Mesozoic marbles and Paleozoic gneisses (due to the interplay of the basement “Pelagonian Indentor”, see below).
As this lithological contrast is reflected in the clast composition of the deposits above, authors conclude that most of the basin fill was sourced in the west before the Late Oligocene (Krania and Eptachorion F.) and in the east after that time (i.e. Pentalofos and Tsotyli [Desprairies, 1979]).
That is not necessarily true for Oligocene deposits, most of which are covered by Miocene ones in the eastern part of the MHB. For instance, the Oligocene conglomerates outcropping in the eastern Kalambaka area (Avra, Fig.4D and cf Fig.16A ) are not rich in ophiolitic elements but yet they are rich in gneiss pebbles coming from the pelagonian domain located on the eastern side of the MHB.
Miocene transport paths may locally be more complex, especially because of the northward deepening of the depositional setting but paleocurrent analysis based on many clasts imbrication, show that most of the basin fill was sourced in the east since the Early Miocene (i.e. Pentalofon and Tsotyli; [Desprairies, 1979 ; Ferriere et al., 2011]), even on the westernmost part of the MHB, as in the Mitropoli area (Pl. II-E).
Eastward migration of subsidence
Starting in the Oligocene, a generalized subsidence of the MHB is recorded by the basin depocenter of each major stratigraphic sequence. Subsidence progressively shift towards the east (Figs. 10 and 11).
Concerning the amount of subsidence and its behaviour in 3D, the lack of available boreholes, drillholes, and seismic profiles, as well as local erosion within the series and the fact that turbiditic slope depositional systems are poor bathymetric indicators, bring about uncertainties.
Figure 10. Paleogeographic synthesis of possible basin and sub-basins extension at different stages of MHB evolution
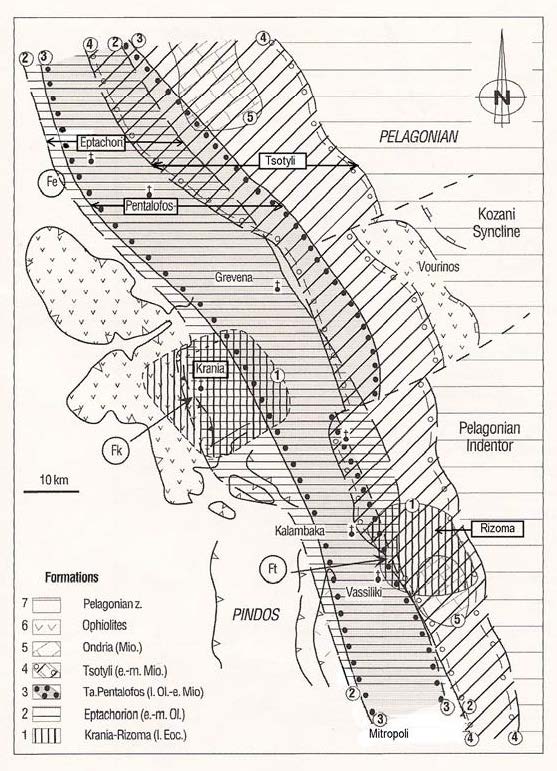
Paleogeographic synthesis of possible basin and sub-basins extension at different stages of MHB evolution (modified after Ferriere et al., 2004). The limits here minimise the depositional areas (e.g. we could not exclude a possible connection of the sea between Krania and Rizoma in the upper Eocene, especially along the tectonic structures bounding the Pelagonian Indentor). Abbreviations: Eoc: Eocene, Ol: Oligocene, Mio: Miocene, e: early, m: middle, l: late. 1 to 5: different MHB Formations, 6:Ophiolites and 7: infra-ophioltic Pelagonian basement.
However, some curves based on extrapolated vertical sedimentary records, from outcrops, map- derived cross-sections and published seismic profiles have been proposed [Kontopoulos et al., 1999 ; Ferriere et al., 2004] (Fig.11).
The general pattern of the eastward migration of MHB depocentres and associated subsidence is synthesized by the map in Fig. 10 (see also schematic curves Fig.11). The forcing mechanisms are discussed below (see Fig. 19).
Figure 11. Subsidence characteristics
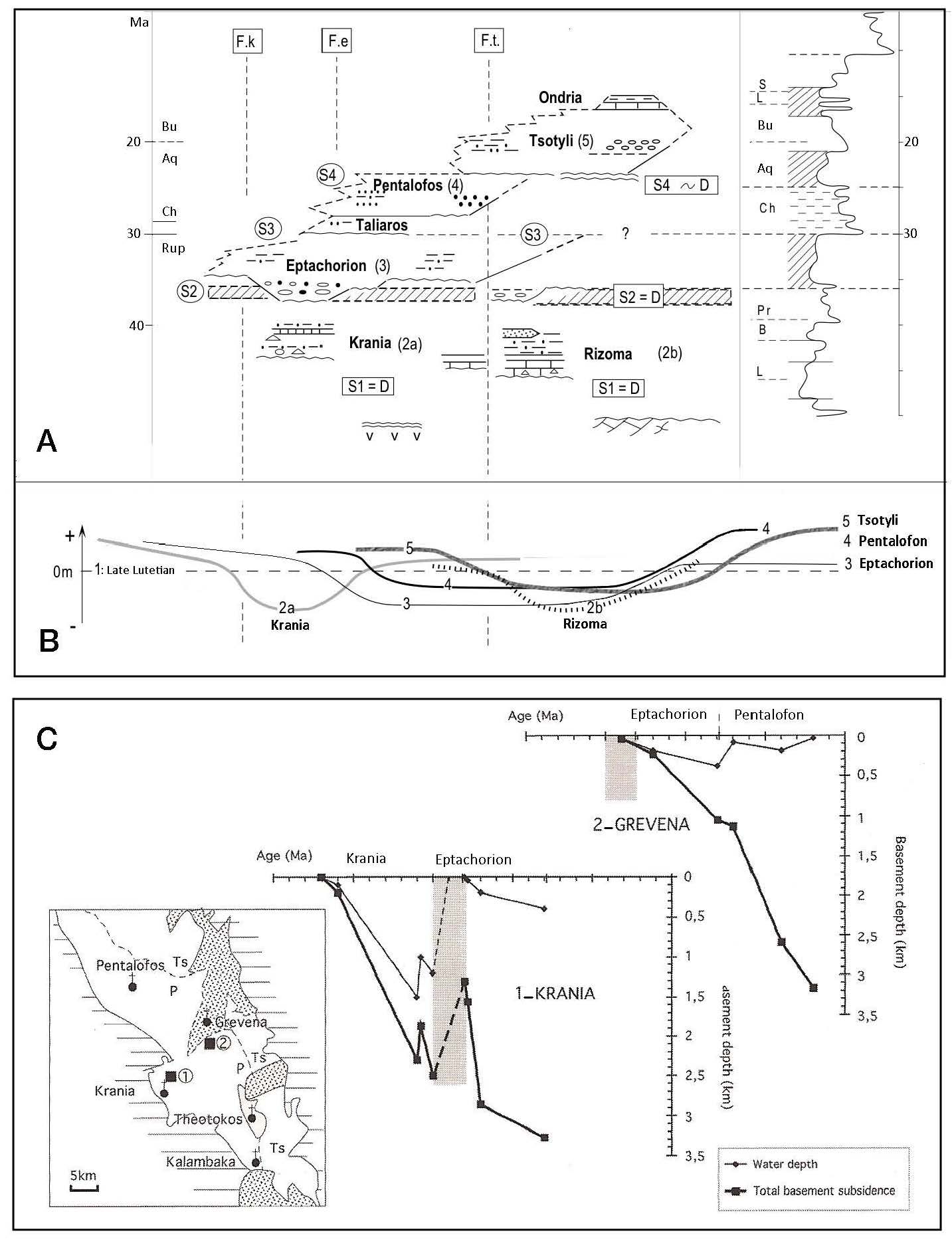
A and B: Schematic drawings showing subsidence migration toward the east (A: lithologic Formations ; B: schematic curves ). Abbreviations: L (Lutetian) to S (Serravalian) : Eocene to Miocene stratigraphic stages ; Fe, Fk, and Ft: faulted-flexures of Eptachorion (Fe), Krania (Fk) and Theopetra-Theotokos (Ft) ; S: significative surfaces ; D : Unconformities.
C: Subsidence curves concerning the central part of the MHB (1:Krania and 2: Grevena series) from our own field data and from seismic published data [Kontopoulos et al., 1999 and Zelilidis et al. 2002]. Approximations on the subsidence calculations are related to some age (see Fig.7) or Formation thicknesses uncertainties, and mainly to paleobathymetric data, particularly for deep water facies (i.e. turbidites). Backstripping has been computed with SUBSILOG (Dubois et al., 2000), using the standard parameters defined by Sclater & Christie (1980). Grey area (ca 35-33 Ma) corresponds to the main compressive tectonic event at the Eocene-Oligocene boundary. Modified after Ferriere et al. [2004].
The two subsidence curves proposed by Kontopoulos et al. [1999] evidence an uplift stage during Pentalofon sedimentation, from 21 to 16 Ma, but they are only representative of the axis of the present MHB (areas of maximum residual thicknesses).
Eustatic versus tectonic controls
The paleogeographical sketch (Fig.10) is based on subsidence but also lateral and vertical facies variations. The frequent absence of shoreline deposits and possible erosion bring(s) about uncertainties concerning the true extension of the basin limits through time. Therefore, the proposed limits minimise the marine depositional area extension: i.e., the Rizoma and Krania sub-basins probably respectively extended to the north and to the east along the tectonic structures of the Pelagonian Indentor, but they were certainly partly eroded at the Eocene - Oligocene boundary.
Locally, the limits of drastic facies changes follow tectonic structures (as at the Krania sub-basin northern limit, Fig.3 and cf. fig.14). However, even if the major geographic gap between Pentalofon and Tsotyli Formations follows the Theopetra-Theotokos structure (Fig.4), no major facies change occurs at the boundary between these two conglomeratic Formations in this area.
By contrast, the main lithologic changes from Eptachorion marls to Pentalofon conglomerates are not associated to major changes in basin limits. This would mean that, if the lithologic change is triggered by tectonics, the tectonic hinge line was located near the paleocoast, as was the case in the Meteora area [Ori and Roveri, 1987; Ferriere et al., 2004].
Alternatively, this suggests other kinds of controls as climate or eustatic changes. Zelilidis et al. [2002] argue that all the stratigraphic occurrences of lowstand facies compare closely with published eustatic sea-level curves (Fig. 12) . However, this apparent correlation remains more than questionnable, because (i) there is no accurate biostratigraphic control and (ii) the required tectonic calendar is not accurately established.
Figure 12. Eustatic sea level variations compared to the MHB lithologic formations ages
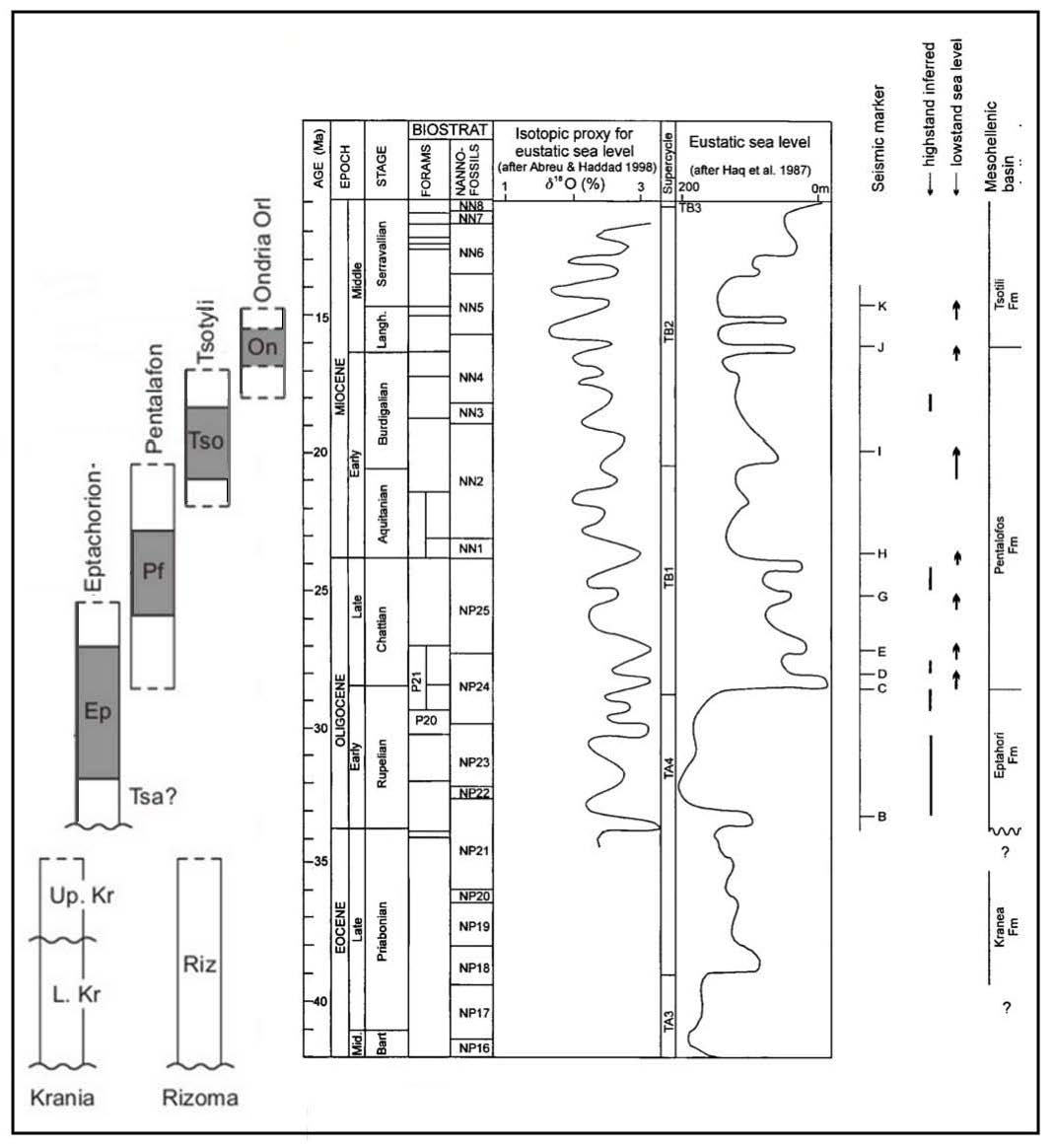
Eustatic sea level variations compared to the MHB lithologic formations ages showing the uncertainties concerning the eustatic control of the MHB evolution. Right part of Fig.11 from Zelilidis et al. [2002]; left part from our own data.
Zelilidis et al. [2002] suggest that the major Oligocene sea-level drop would be responsible for the abrupt change from Eptachorion marls to the Lower Meteora Conglomerate (Fig.12). However, Ori and Roveri [1987] suggested a tectonic control at origin and development of the Meteora deposits, which was evidenced by Ferriere et al. [2011].
If eustatism changes have, of course, some control on the marine deposits of the MHB, in the next section, we summary the data showing that tectonic is the most important control on the paleogeographic evolution of the MHB (cf. infra).