Geological outline
The Campi Flegrei volcanic field and the Somma-Vesuvius complex are two still active volcanic districts belonging to the so-called Plio-Pleistocene “Italian Potassic Magmatism” (e.g., Appleton, 1972; Beccaluva et al. 1991; Conticelli et al., 2002, 2004; Peccerillo, 2005). Volcanic activity was related to a very complex geodynamic scenario, dominated by the opening of the Tyrrhenian basin and to the build-up of the Apennine-Maghrebides chain (e.g., Peccerillo e Manetti, 1985; Di Girolamo et al., 1988; Di Girolamo and Morra, 1988; Beccaluva et al., 1991; Doglioni et al., 1997; Gueguen et al., 1998; Lustrino, 2000; Faccenna et al., 2004; Garzanti and Malusà, 2008). Several discussions have been very active in the past regarding the “anorogenic” versus “orogenic” nature of this magmatism, while at the present its “orogenic” character seems to be widely accepted (e.g., Savelli, 2000, 2002; Conticelli et al., 2002; 2004; Mattei et al., 2004, Peccerillo 2005; Peccerillo and Lustrino, 2005).
Italian magmatism developed in several volcanic districts, mainly dislocated in a very narrow area in central-southern Italy. Magmatism was mainly effusive, with products showing a serial character ranging from calcalkaline to potassic alkaline to ultrapotassic (e.g., Conticelli et al., 2002, 2004; Peccerillo, 2005). Campanian volcanism is only a small part of the wider Roman Province (including all the volcanic provinces of Latium and Campania; Conticelli et al., 2004). It is to note, however, that some authors have argued that geochemical and isotopic evidences seem to indicate that Campanian volcanites are more akin to the products of the potassic series of Stromboli (Aeolian arc), rather than to the products of the other districts of the Roman Province (e.g., Peccerillo, 2001, 2005). Campanian volcanic products essentially show two different serial affinities, namely a potassium (shoshonitic) series and a high potassium series (respectively, KS and HKS), with products of the two series being very recurrently found together in the same volcanic district (e.g., at the Roccamonfina and the Somma-Vesuvius volcanic complexes; Conticelli et al., 2002, 2004). Rocks belonging to the KS are represented by saturated to slightly undersaturated products, with K2O/Na2O approaching unity. Compositions range from shoshonitic basalt, through shoshonite and latite to trachyte. The HKS is made of ultrapotassic, mildly to strongly Si-undersaturated leucite-bearing products, whose composition range from leucitite and basanite through leucite tephrite, leucite tephriphonolite to phonolite (e.g., Conticelli et al., 2002, 2004; Peccerillo, 2005).
Several studies have been published on the nature of the mantle sources of the whole Italian potassic province (e.g., Vollmer, 1977; Hawkesworth and Vollmer, 1979; Beccaluva et al., 1991; Conticelli et al., 2002, 2004; Peccerillo, 2005), as well as on the sources of the rocks of the Campanian region (e.g., D’Antonio and Di Girolamo, 1994; D’Antonio et al., 1996, 1999a, 2007; Piochi et al., 2004). Many of them evidenced the existence of a great variability of K2O and incompatible element contents, coupled with a similar variability of Sr, Nd and Pb isotope ratios, usually considered to be related to crustal assimilation and source contamination processes. The most recent models (e.g., Conticelli et al., 2002, 2004; Peccerillo, 2005) argue for a very complex source for the Campanian rocks, made of several different components, including subducted continental crust, depleted MORB-mantle and HIMU components. The various degree of Si-undersaturation of the products is probably reflecting a different residual character of the involved sources (lherzolitic for the more undersaturated and harzburgitic for the more saturated ones), coupled with variable metasomatization and partecipation of the metasomatic phases in the melting processes.
The Campi Flegrei
The Campi Flegrei is a volcanic field located immediately west of the city of Naples (Fig. 1). Its volcanic history was characterized by a great number of eruptions, giving birth to mainly monogenetic edifices and emplacing huge volumes of pyroclastic rocks and very sporadic small-scale lava flows. Phlegrean volcanism includes not only the activity developed on the continent (the Campi Flegrei strictu sensu) but also the activity developed on the islands of Ischia and Procida, which share many geological, volcanological, petrochemical and petrological similarities with Campi Flegrei s.s. (Di Girolamo et al., 1984, 1995; De Astis et al., 2004).
Figure 2. Geochronology of Campi Flegrei eruptions.
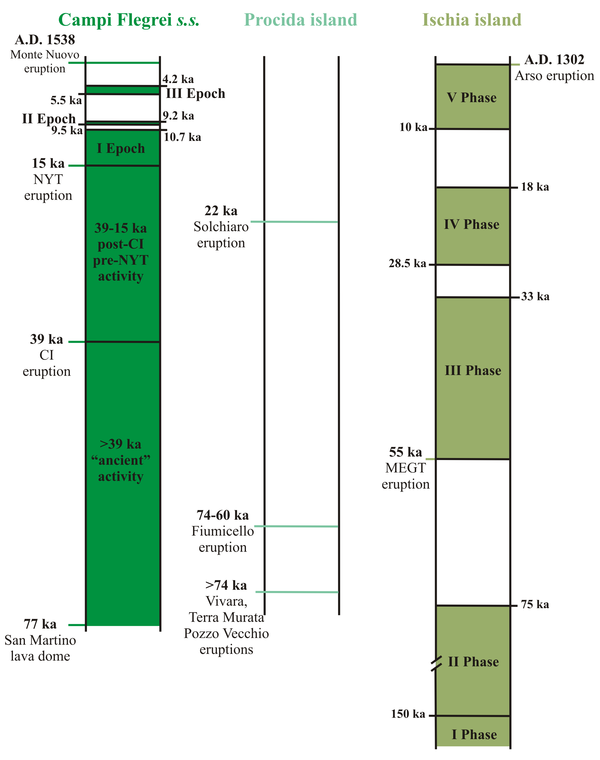
Chronological sketches summarizing the periods of active volcanism in the continental (i.e., Campi Flegrei strictu sensu) and insular (Procida and Ischia islands) portions of the Campi Flegrei district. White areas represent known periods of volcanic quiescence, while coloured areas represent periods of active volcanism (with coloured thick marks corresponding to particularly significative volcanic events). See text for a more detailed treatment.
The beginning of Phlegrean volcanism is not yet well constrained. Di Girolamo et al. (1976, 1996) and Barbieri et al. (1979) reported the occurrence in some exploratory wells drilled in the Campanian Plain of calcalkaline volcanics (lavas and volcanoclastites) dating back to ~2.0 Ma and tentatively related them to the very first manifestations of magmatism in the Campanian region related to build-up of the Apennine chain. De Vivo et al. (2001) suggested that Campi Flegrei volcanism began at least ~315 ka, on the basis of geochronological data on previously unrecognized pyroclastic deposits outcropping in the peripheral portions of the Campanian Plain. Not taking into account these poorly studied and constrained deposits, it should be noted that insular volcanism has been historically retained to largely predate continental one. More specifically, the oldest outcropping products of the entire Phlegrean area were commonly thought to be those marking the base of the volcanic sequence at Ischia, dating back to more than 150 ka (Poli et al., 1987; Vezzoli, 1988).
The volcanic history of the Ischia island is generally subdivided into five phases (Vezzoli, 1988; Fig. 2):
• 1st phase (>150 ka). The pyroclastic deposits of this phase belong to an ancient volcanic complex, probably covering a wider area with respect to the present island and now almost completely destroyed. Volcanic activity was mainly represented by very energetic pyroclastic eruptions. The observation of two well-developed humified horizons suggests the occurrence of at least two long repose periods (Poli et al., 1987).
• 2nd phase (150-75 ka). Lava eruptions are the main feature of this phase. The distribution of lava domes indicates a sub-circular structure along the south-western and south-eastern sides, possibly suggesting the presence of a caldera rim with a large depression at the center of the island.
• 3rd phase (55-33 ka). After ~20 kyrs of quiescence, a new phase of activity occurred, starting with the Monte Epomeo Green Tuff (MEGT, ~55 ka, K/Ar; Vezzoli, 1988) eruption, the most powerful volcanic event on the island. The deposits of this event show the typical ignimbritic welded facies (with abundant pumices, lithics and biotite and K-feldspar loose crystals) only in correspondence of the Monte Epomeo volcano-tectonic horst. The characteristic greenish colour is due to sea-water alteration of the vitric ignimbrite matrix in hydrothermal conditions. Other facies include non-welded ash flow and pumice flow deposits and alternances of pyroclastic flow deposits with an explosive breccia at the bottom (Vezzoli, 1988).
• 4th phase (28.5-18 ka). Volcanism is concentrated in the south-western sector of the island, probably related to the reactivation of the NE-SW fault system corresponding to the volcanic alignment of Ischia, Procida and continental Campi Flegrei.
• 5th phase (10 ka-A.D. 1302). After a new period of quiescence (~10 kyrs), a new reprise of volcanism occurred, mainly involving the eastern sector of the island, corresponding to the Ischia Graben area (Vezzoli, 1988) and possibly related to the resurgence of the Monte Epomeo block (Orsi et al., 1991). This last phase was almost exclusively characterized by volcanic eruptions coming from monogenetic vents, and is closed by the A.D. 1302 historical eruption of Arso (Gasparini and Adams, 1969; Gillot et al, 1982; Vezzoli, 1988; Civetta et al., 1991c).
The onset of volcanism on Procida island is generally thought to post-date Ischia's oldest activity (i.e., the first two phases). The oldest products occurring on the island are represented by the pyroclastic deposits which built the Pozzo Vecchio, Vivara and Terra Murata volcanoes (Rosi et al., 1988; De Astis et al., 2004; Fedele et al., in press), which must be collocated somewhere before 74 ka, given their occurrence in lower stratigraphic position with respect to the deposits of the Pignatiello formation of Ischia (dated between 55 and 74 ka; Vezzoli, 1988). The pyroclastic products of the youngest edifices of Fiumicello (60-74 ka; Fedele et al., in press) and Solchiaro (~17-19 ka, 14C ages; Alessio et al., 1976; Lirer et al., 1991; calibrated to 22 ka by Fedele et al., in press) are the only other deposits clearly ascribable to Procida volcanism. A single effusive event is recorded on the island, represented by emission of the Punta Ottimo lava dome (Di Girolamo and Stanzione, 1973; Di Girolamo et al., 1984; De Astis et al., 2004; Fedele et al., in press).
As regards the continental portion of the Campi Flegrei, the first volcanic manifestations seem to be even younger with respect to Procida oldest activity. The products of the Punta Marmolite and Cuma lava domes (respectively ~47 and ~37 ka, K/Ar ages; Cassignol and Gillot, 1982) and of the Tufi di Torre Franco formation (~42 ka, 14C; Alessio et al., 1973) have been historically considered to represent the oldest products of Campi Flegrei s.s. activity. Recent datings have brought the lower age limit of continental activity progressively backwards, firstly to 58 ka (40Ar/39Ar; Pappalardo et al., 1999) and then up to ~77 ka (40Ar/39Ar of the San Martino lava dome, Fig. 3a; Fedele et al., in press).
Volcanism at Campi Flegrei s.s. has been variously subdivided into distinct periods of activity (e.g., De Lorenzo, 1904, Rittmann, 1950; Di Girolamo et al., 1984; Rosi and Sbrana, 1987). Notwithstanding the differences between them, these schematizations are fundamentally based on the identification of two major volcanic events, whose deposits have always been used as efficient stratigraphic markers.
Figure 3. Petrography of Campi Flegrei rocks.
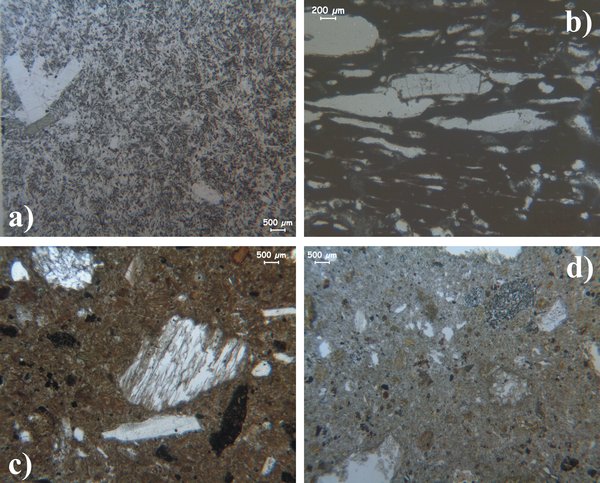
Microphotographs of some representative Campi Flegrei products: a) plane polarized view of a trachytic lava from the San Martino lava dome showing a weakly porphyritic texture with phenocrysts of sanidine and clinopyroxene (left side) set into a groundmass predominantly made by sanidine microcrysts; b) plane polarized view of the trachytic welded tuff from the Piperno unit showing the typical eutaxitic texture with iso-oriented collapsed scoriae (fiammae) set into a cineritic matrix (in the center of the picture a sanidine phenocryst is also visible); c) plane polarized view of a trachytic tuff of the CI formation [belonging to the WGI unit of Cappelletti et al. (2003)] showing pumices, lava lithic fragments, sanidine, clinopyroxene and biotite (upper side of the picture) loose crystals set into abundant ashy matrix; d) plane polarized view of a trachytic tuff of the NYT formation showing pumices and lava lithics plus minor sanidine and clinopyroxene loose crystals set into an ashy matrix.
The first is the Campanian Ignimbrite (hereafter CI) eruption, the most powerful event ever occurred in the Mediterranean area in the last 200 ka (Barberi et al., 1978). The eruption, occurred ~39 ka (40Ar/39Ar; Deino et al., 1994; De Vivo et al., 2001; Ricci, 2000), was probably accompanied by an extensive caldera collapse, which gave birth to the Campi Flegrei caldera (e.g., Rittmann, 1950; Rosi et al., 1983; Rosi and Sbrana, 1987; Orsi et al., 1996; Perrotta et al., 2006). The CI eruption emplaced a widespread pyroclastic fall and flow sequence, basically of trachytic composition (plus rarer phonolites), covering an area from the Campi Flegrei district to as far away as the eastern Mediterranean Sea and Russia (Barberi et al. 1978; Thunnel et al., 1979; Sparks and Huang, 1980; Cornell et al., 1983; Rosi and Sbrana, 1987; Scandone et al., 1991; Fedele et al., 2002; Wulf et al., 2004; Perrotta et al., 2006; Pyle et al., 2006). Proximal deposits, preserved in limited outcrops along the Campi Flegrei caldera rim, constitute a complex sequence of six units (the so-called “Breccia Museo”) consisting mainly of coarse, lithic-breccia and welded horizons (Rosi and Sbrana, 1987; Perrotta and Scarpati, 1994; Melluso et al., 1995; Rosi et al., 1996; Perrotta et al., 2006; Fedele et al., 2008). One of the most particular volcanic units of the proximal sequence is represented by the so-called “Piperno” (e.g., De Lorenzo, 1904; Rittmann, 1950; Perrotta and Scarpati, 1994; Rosi et al., 1996; Perrotta et al., 2006; Fedele et al., 2008), a deposit made of alternating beds of welded ash with flattened scoriae (fiammae), resulting in the typical eutaxitic texture (Fig. 3b), and a monolithologic coarse breccia made up of grey lava fragments, exposed exclusively in the eastern sector of Campi Flegrei and in the city of Naples and widely used as a building stone (see following sections).
Deposits of the CI found in medial exposures (i.e., the Campanian Plain and the Apennine chain, up to 80 km from the vent area) consist mainly of a stratified plinian pumice deposit overlain by a grey welded tuff [the WGI unit of Cappelletti et al. (2003); Fig. 3c] that grades upwards into a yellow one [the LYT unit of Cappelletti et al. (2003); see also Fisher et al. (1993), Rosi et al. (1999), Perrotta and Scarpati (2003)]. The type-sequence is completed by a basal stratified, incoherent ash to sandy deposit and a topmost incoherent coarse pumice deposit with an ashy matrix [respectively, USAF and CPF, Cappelletti et al. (2003)]. Finally, in distal areas CI deposits have been correlated to a stratified ash layer identified in eastern Europe (Sparks and Huang, 1980; Cornell et al., 1983). The volume of the ignimbrite is not well constrained, and proposed estimates range from 80 to 500 km3 (e.g., Barberi et al., 1978; Thunnel et al., 1979; Fisher et al., 1993).
The second most explosive event of Campi Flegrei history is the Neapolitan Yellow Tuff (NYT) eruption, for which 40Ar/39Ar datings indicate an age of ~15 ka (40Ar/39Ar; Deino et al., 2004; Insinga et al., 2004). The eruption, accompanied by a second caldera collapse episode (Di Girolamo et al., 1984; Orsi et al., 1992a, 1996; Scarpati et al., 1993), emplaced a deposit mainly consisting of two distinctive members: member A, a succession of cineritic and pumiceous lapilli layers, and member B, a deposit made of cineritic layers with dispersed rounded pumices (Scarpati et al., 1993). The deposits of the latter member, constituting the great part of the entire erupted sequence, occur as a yellowish massive lithified tuff (the so-called “Tufo”; Fig. 3d) in the proximal areas, as an unlithified light grey pumiceous cinerite (“Pozzolana”) in the more distal exposures (e.g., Di Girolamo et al., 1984; Rosi and Sbrana, 1987; Orsi et al., 1991, 1992a; Cole and Scarpati, 1993; Scarpati et al., 1993; Wholetz et al., 1995; Valentini et al., 2008). The emplaced products, ranging in composition from latite to trachyte, cover an estimated area of ~1000 km2 (Wholetz et al., 1995), reaching a total dense rock equivalent volume of ~50 km3 (Scarpati et al., 1993).
Campi Flegrei volcanic activity postdating NYT eruption, generally referred to as the “recent” phase, is concentrated well within the boundary of the NYT caldera. Such phase of volcanism has been very deeply studied in the last 20-25 years, allowing to depict a very detailed reconstruction of the chronology of the numerous events occurred. The reason for this is twofold. On one hand, a detailed knowledge of the recent behaviour of the Campi Flegrei is necessary for a correct evaluation of the volcanic hazard (and, consequently, of the volcanic risk) related to the district. Moreover, the two caldera collapses created favourable outcropping conditions for the youngest deposits (while, on the contrary, much of the deposits emplaced before one or both the collapses are presently very hard to be recovered due to their sinking and their burial beneath the products of the recent activity). According to Di Vito et al. (1999a) and Orsi et al. (2004), Campi Flegrei recent volcanism can be subdivided in three epochs of activity, identificated on the basis of the recognition of three periods of volcanic quiescence. The first epoch goes from 15 ka (i.e., age of the NYT eruption) to 9.5 ka [14C age, recalibrated to ~10.7 ka by Fedele et al. (2009a)] and is characterized by at least 37 explosive events (with magmatic to phreatomagmatic eruptive style), the most energetic of which is the Pomici Principali eruption (Astroni volcano; Rittmann, 1950; Di Girolamo et al., 1984; Rosi and Sbrana, 1987; Lirer et al., 1987a; Di Vito et al., 1999a), for which Di Vito et al. (1999a) obtained a 14C age of ~10.3 ka [recalibrated at ~12.2 ka by Fedele et al. (2009a)]. This epoch is followed by a period of quiescence, testified by the development of a thick paleosoil horizon extensively recognized by the authors throughout the Phlegrean district. The second epoch goes from 8.6 to 8.2 ka [14C ages of Di Vito et al. (1999a), recalibrated respectively to ~9.5 and ~9.2 ka by Fedele et al. (2009a)] and is characterized by only 6 episodes of low volcanic magnitude which mainly concentrated in the north-eastern sector of the district. After a new period of prolonged volcanic quiescence, a new epoch of activity began, spanning the time interval between 4.8 and 3.8 ka [14C ages of Di Vito et al. (1999a), recalibrated respectively to ~5.5 and ~4.2 ka by Fedele et al. (2009a)]. This phase was characterized by 20 explosive and 3 effusive events (generally concentrated along fault zones running along the north-western border of the NYT caldera; Orsi et al., 1996), the most powerful of which was the Agnano-Monte Spina eruption (4.1 ka, 40Ar/39Ar age; de Vita et al., 1999). The end of the third epoch is marked by a new phase of quiescence, interrupted by the A.D. 1538 Monte Nuovo historical eruption (Di Vito et al., 1987; Lirer et al., 1987b; D’Oriano et al., 2005; Piochi et al., 2005). It is worth of note that the above schematization of Campi Flegrei recent activity, although very detailed and based on a notable wealth of data, seems to need further reworking in the light of recent absolute datings (e.g., Insinga et al., 2006; Fedele et al., 2009a), which collocate some eruptive events into periods formerly retained of volcanic quiescence.
Figure 4. Classification of Campi Flegrei and Somma-Vesuvius rocks.
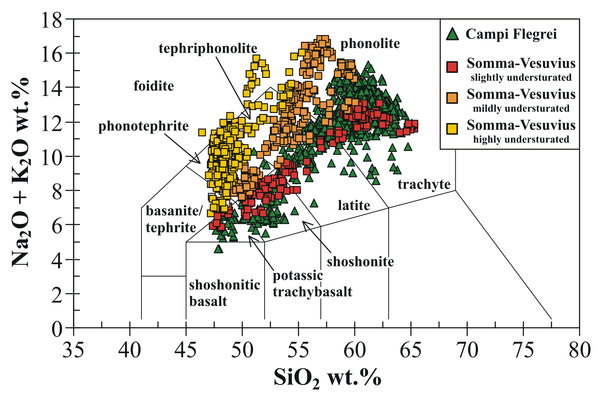
Total-Alkali vs. Silica classification diagram (Le Bas et al., 1986) for Campi Flegrei and Somma-Vesuvius rocks from the existing literature data [Campi Flegrei: Albini et al. (1977), Armienti et al. (1983), Barberi et al. (1978), Beccaluva et al. (1991), Bohrson et al. (2006), Carbone et al. (1984), Civetta et al. (1988, 1991a, 1997), Crisci et al. (1989), D’Antonio and Di Girolamo (1994), D’Antonio et al. (1996, 1999a, 1999b, 2007), De Astis et al. (2004), de Vita et al. (1999), Di Girolamo (1968, 1970), Di Girolamo et al. (1973, 1984, 1995), Di Girolamo and Stanzione (1973), Di Girolamo and Rolandi (1979), D’Oriano et al. (2005), Fedele (2006), Fedele et al. (2006, 2008), Fowler et al. (2007), Ghiara (1989-1990), Ghiara et al. (1977, 1979), Insinga (2004), Lirer et al. (1987a), Lustrino et al. (2002), Melluso et al. (1995), Orsi et al. (1992a, 1992b, 1995), Pappalardo et al. (1999, 2002a, 2002b), Piochi et al. (1999), Poli et al. (1987), Rosi and Sbrana (1987), Scarpati et al. (1993), Signorelli et al. (1999a), Vezzoli (1988), Villemant (1988); Somma-Vesuvius: Aulinas et al., 2007; Ayuso et al. (1998), Barberi et al. (1981), Black et al. (1998), Brocchini et al. (2001), Cioni et al. (1992, 1995, 1998, 2003), Civetta and Santacroce (1992), Civetta et al. (1991b, 2004). Cortini and Hermes (1981), Cortini et al. (2004), Di Renzo et al. (2007), Di Vito et al. (1999b), Fulignati et al. (2000), Landi et al. (1999), Marianelli et al. (1999), Piochi et al. (2006), Rolandi et al. (2004b), Rosi and Santacroce (1983), Santacroce (1987), Santacroce et al. (1993), Savelli (1967a), Signorelli et al. (1999b)].
At present, Campi Flegrei activity is mainly fumarolic and hydrothermal, with sporadic bradiseismic episodes, like those of the 1969-1972 and 1982-1984 crises, during which a total round uplift of 3.5 m was recorded near the town of Pozzuoli (Barberi et al., 1991).
Campi Flegrei rocks range in composition from potassic alkaline basalt (“shoshonitic basalt”) to trachyte and phonolite, defining a typical potassium alkaline “shoshonitic” series. The most differentiated products, and particularly the trachytes, are by far the dominant lithotypes (Fig. 4), whereas the less evolved shoshonitic basalts and shoshonites are sensitively rarer, being almost exclusively represented by the products of Procida island. Rock textures vary from totally aphyric to slightly porphyritic (up to ~30% of phenocrysts). The most abundant mineral phases are represented by clinopyroxene, plagioclase, sanidine, biotite and magnetite. Olivine is confined to the least evolved lithotypes (from shoshonitic basalts to latites). Common accessory phases are apatite and zircon, while brown amphibole, titanite (particularly in Ischia products) and feldspathoids (usually nepheline) have been found only in the most differentiated products. Sodalite, aegirine and aegirine-augite have been sporadically observed in the most evolved trachytes of Ischia (Crisci et al., 1989).
Chemical composition of the main mineral phases can be briefly summarized as follows.
Olivine. Olivine crystals, typically confined to the very few least differentiated rocks, show very narrow compositional ranges, spanning from Fo88 to Fo80. A sensitively wider spectrum has been reported for olivine crystals from Ischia products, ranging from Fo89 to Fo72 (Crisci et al., 1989; Di Girolamo et al., 1995; Piochi et al., 1999). Phenocrysts are usually altered to iddingsite (Armienti et al., 1983) and contain Mg-Cr spinel inclusions (D’Antonio and Di Girolamo, 1994).
Clinopyroxene. Clinopyroxene is the dominant phenocryst in the least differentiated rocks, while its abundance in the more differentiated products is inversely proportional to the degree of evolution. Clinopyroxene from the least differentiated volcanics is pale green to greenish, ranging from Mg-rich diopside to Fe-rich diopside, commonly showing both normal and reverse zoning (Beccaluva et al., 1990; D’Antonio and Di Girolamo, 1994). In latites and trachytes two clinopyroxenes typically occur: a greenish salitic one and a colourless diopsidic, with the latter being generally less abundant. Chemical compositions are fairly homogeneous (from Ca47Mg40Fe13 to Ca47Mg35Fe18 for salite and from Ca47Mg49Fe4 to Ca47Mg45Fe8 for diopside; Vezzoli, 1988; Melluso et al., 1995; Piochi et al., 1999; Ricci, 2000; Fedele et al., 2008, 2009b; Fig. 5). However, zoned crystals also occur, usually showing a diopsidic core rimmed by a salitic border (Civetta et al., 1997; Ricci, 2000; Fedele et al., 2008). Clinopyroxene crystals from Ischia trachytes and phonolites are usually characterized by high aegirine contents (Na2O up to ~9 wt.%; Poli et al., 1987; Crisci et al., 1989).
Figure 5. Classification of Campi Flegrei and Somma-Vesuvius clinopyroxenes.
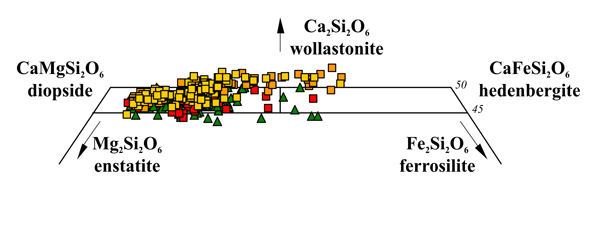
Composition of representative Campi Flegrei and Somma-Vesuvius clinopyroxene crystals from the existing literature data [Campi Flegrei: Armienti et al. (1983), Civetta et al. (1997), de Vita et al. (1999), Di Girolamo et al. (1995); Fedele et al. (2006, 2008), Fulignati et al. (2004), Ghiara et al. (1979), Melluso et al. (1995), Orsi et al. (1995), Pappalardo et al. (2002a), Piochi et al. (1999), Poli et al. (1987), Signorelli et al. (1999a); Somma-Vesuvius: Barberi et al. (1981), Cioni et al. (1995, 1998), Fulignati et al. (2000), Marianelli et al. (1999), Santacroce (1987), Santacroce et al. (1993), Savelli (1967a)] Symbols as in Fig. 4.
Plagioclase. Plagioclase is a very common phase in the least differentiated rocks, being progressively replaced by K-feldspar in the most evolved products. In trachybasalts plagioclase compositions span a very large spectrum, from An95 to An56 (Armienti et al., 1983; D’Antonio and Di Girolamo, 1994). With the progressive transition to latites and trachytes, Na2O and K2O contents tend to constantly increase, leading to andesinic-oligoclasic compositions (from An32 to An25; Crisci et al., 1989; Piochi e al., 1999; Fedele et al., 2008; Fig. 6).
K-Feldspar. Sanidine occurs in trachybasalts only as small individuals dispersed in the groundmass, whereas in latites is also present as plagioclase outer rims and in trachytes it represents the absolutely dominant phenocryst phase, accounting for ~90% of the total phenocryst content (Armienti et al., 1983; Civetta et al., 1997). Orthoclase molecula content goes from ~Or73 of the least differentiated rocks to ~Or87 of trachytes (Fedele et al., 2008) and then decreases to more sodic and calcic compositions up to Or63 in the most differentiated phonolites (Armienti et al., 1983; Fig. 6). Very Na2O-rich varieties have been reported for Ischia trachytes (up to Or34; Crisci et al., 1989; Piochi et al., 1999).
Figure 6. Classification of Campi Flegrei and Somma-Vesuvius feldspars.
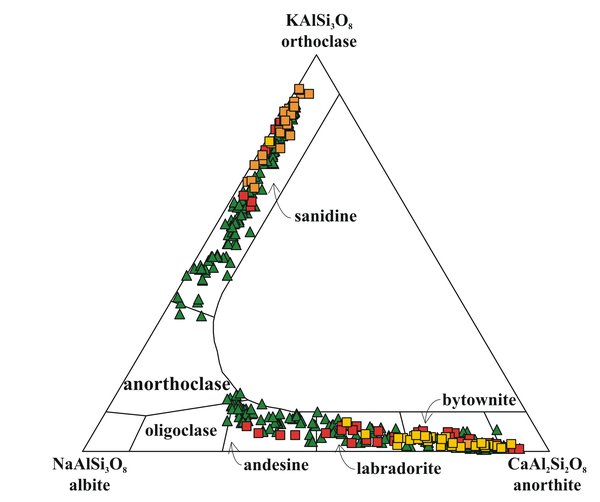
Composition of representative Campi Flegrei and Somma-Vesuvius feldspar crystals from the existing literature data [Campi Flegrei: Armienti et al. (1983), Civetta et al. (1997), de Vita et al. (1999), Di Girolamo et al. (1995); Fedele et al. (2006, 2008), Fulignati et al. (2004), Ghiara et al. (1979), Melluso et al. (1995), Orsi et al. (1995), Pappalardo et al. (2002a), Piochi et al. (1999), Poli et al. (1987), Signorelli et al. (1999a); Somma-Vesuvius: Barberi et al. (1981), Cioni et al. (1995), Landi et al. (1999), Santacroce (1987), Santacroce et al. (1993),] Symbols as in Fig. 4.
Biotite. Biotite is quite recurrent in Campi Flegrei products, occurring as phenocrysts or microphenocrysts from latites to trachytes and as small groundmass individuals in trachybasalts (Armienti et al., 1983). Crystals are generally unzoned Mg-biotites [according to the nomenclature proposed by Rock, (1982)], usually containing apatite inclusions (Pappalardo et al., 2002a) and showing moderate TiO2 contents (4.7-4.8 wt.%; Melluso et al., 1995; Fedele et al., 2008). Its occurrence in trachytic products is a clear evidence of very high H2O.
Opaque oxides. A Ti-magnetite phase is present in Phlegrean rocks as phenocrysts and microphenocrysts, particularly abundant in latites (Armienti et al., 1983). The ulvöspinel content constantly decreases from trachybasalts and latites (~25-28%) to trachytes (~16-20%; Armienti et al., 1983; Melluso et al., 1995; Civetta et al., 1997; Fedele et al., 2008).
Figure 7. Major elements of Campi Flegrei and Somma-Vesuvius rocks
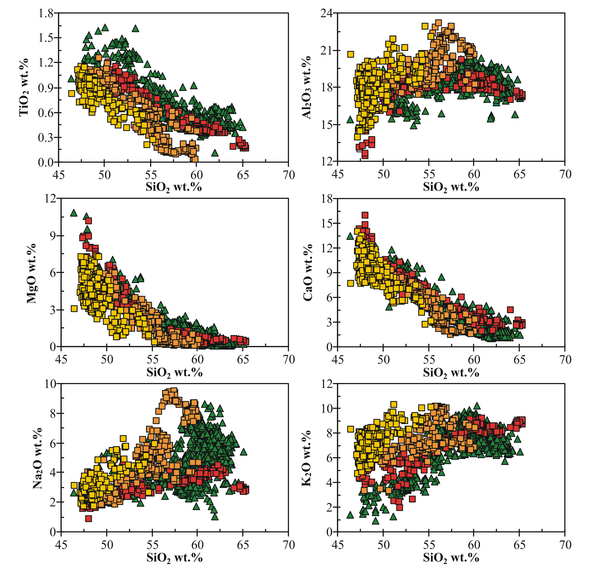
Selected major element binary diagrams for Campi Flegrei and Somma-Vesuvius rocks from the existing literature data (see Fig. 3 for references). Symbols as in Fig. 4.
Phlegrean volcanites are characterized by low TiO2, Nb, and Ta, high K2O and Al2O3 and high LILE/HFSE (Large Ion Litophile Elements; High Field Strength Elements) and LREE/HREE ratios (Light Rare Earth Elements; Heavy Rare Earth Elements). With increasing rock differentiation, TiO2, Fe2O3, MgO, CaO and P2O5 decrease, SiO2 and Na2O increase (Fig. 7). K2O firstly slightly increases, then, when magmas approach trachytic compositions, it rapidly falls. Among trace elements, LREE, Y, Zr, Nb, Th, Rb and Ta are all strongly incompatible, whereas V, Sc, Ba and Sr show a compatible character (Fig. 8). These variation patterns suggest crystal fractionation of the observed mineral phases, mainly clinopyroxene (CaO, MgO, Fe2O3, V and Sc decrease), plagioclase (CaO, Ba and Sr), Fe-Ti oxides (TiO2, Fe2O3, Sc and V) and apatite (P2O5). The “bell-shaped” differentiation pattern of K2O is clearly related to the scarce or even absent sanidine crystallization during the first phases of differentiation (dominated by clinopyroxene and plagioclase), followed by its massive fractionation when magmas reach trachytic compositions and sanidine becomes the main fractionating phase.
Figure 8. Trace elements of Campi Flegrei and Somma-Vesuvius rocks
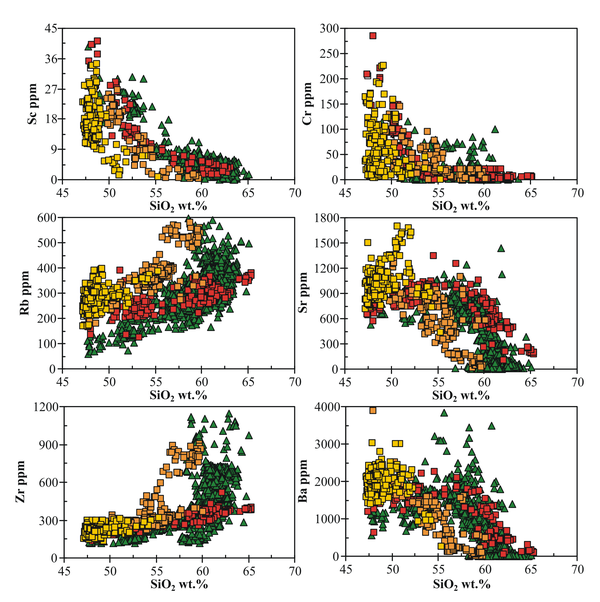
Selected trace element binary diagrams for Campi Flegrei and Somma-Vesuvius rocks from the existing literatuer data (see Fig. 3 for references). Symbols as in Fig. 4.
Even though overall linear fractionation trends seem to suggest for Campi Flegrei rocks an evolution basically dominated by closed-system fractional crystallization, many authors have reported several lines of petrographic, geochemical and isotopic evidences in favour of a possible role (though limited) of open-system processes, such as mixing and/or mingling of different magma batches, magma contamination by hydrothermal fluids, wall-rock assimilation (e.g., Cortini and Hermes, 1981; Vollmer et al., 1981; Villemant et al., 1988; Di Girolamo et al., 1984; Beccaluva et al., 1990; Ghiara et al., 1989-1990; Civetta et al., 1991a, 1997; D’Antonio et al., 1999b; Pappalardo et al., 1999, 2002b; De Astis et al., 2004; Bohrson et al., 2006; Fowler et al., 2007). Chemical zoning of Phlegrean magmatic reservoirs seems to be another common process, as it is evident from the recognition of a mirrored chemical zoning of the erupted products (e.g., Di Girolamo, 1970; Armienti et a., 1983; Melluso et al., 1995; Civetta et al., 1997; de Vita et al., 1999; Pappalardo et al., 2002a; Fedele, 2006; Fedele et al., 2006, 2008, in press).
Finally, restricted to rocks of Ischia island, some authors (e.g., Vezzoli, 1988; Poli et al., 1989; Civetta et al., 1991c) have shown that the products of the five periods of activity are separated by very evident compositional discontinuities. The first of these basically corresponds to the 55 ka MEGT eruption (i.e., the 3rd phase of activity), possibly testifying for a renewal of the magmatic system of the island. Successively, an alkali increase can be observed, followed, at 28.5 ka (4th phase), by a new alkali decrease. A new alkali increase can be detected at 18 ka, followed by a progressive decrease until 10 ka (5th phase), when most of the basic volcanics of the island (predominantly latites and shoshonites) were emplaced.
The Somma-Vesuvius
The Somma-Vesuvius (Fig. 1) is a central volcanic complex, sited not far from the south-eastern part of the city of Naples within the Piana Campana semi-graben, at the intersection of conjugate NW-SE and NE-SW fractures (e.g., Principe et al., 1987; Rolandi et al., 2004a). It is made up of an ancient stratovolcano (Mt. Somma) with a summit caldera, in which the more famous cone of the Vesuvius volcano developed. Andronico et al. (1995), Cioni et al. (1999) and Rolandi et al. (2004a) argued for a multicyclic nature of the Somma summit caldera, formed as a consequence of various caldera-forming events. The primitive Somma stratovolcano vent was probably located about 500 m north of the present crater, at the height of ~1600-1900 m (Cioni et al., 1999) or even higher, up to 2000 m if an original crater width of 0.5 km is considered (Rolandi et al., 2004a). The caldera probably developed as a consequence of a multicyclic process related to repeated plinian eruptions (Andronico et al., 1995; Cioni et al., 1999; Rolandi et al., 2004a). Rolandi et al. (2004a) proposed a compound model involving an “explosive coring”, followed by a repeated “flank failure” caused by phreatomagmatic activity during Avellino and Pompei plinian eruptions. The Vesuvius cone grew up within Mt. Somma caldera after the A.D. 79 eruption (Cioni et al., 1999), mainly due to the interplinian activity occurred between 472 and 1139 and between 1631 and 1944 (Rolandi et al., 1998, 2004a).
The eruptions which characterized the volcanological evolution of the Somma-Vesuvius can be basically ascribed to three different types (Santacroce, 1983, 1987; Civetta et al., 1991b): 1) small volume eruptions (i.e., strombolian and effusive), with the emission of about 0.01 km3 of magma; 2) medium volume eruptions (subplinian; ~0.1 km3); 3) big volume eruptions (plinian; >1 km3). Plinian and subplinian eruptions generally follow a precise eruptive sequence (Civetta et al., 1991b). This seems particularly true for plinian eruptions (mainly because they are much better characterized with respect to subplinian ones), which generally start with the emission of fallout pumice, scoria and ash deposits (the plinian s.s. phase) related to the development of a substained column. This magmatic phase can be interrupted by partial column collapses, with associated emplacement of pyroclastic flow deposits, followed by the final collapse of the eruptive column, marked by pyroclastic flow and lahr deposits (e.g., Sheridan et al., 1981; Sigurdsson et al., 1985). The eruption ends with a phreatomagmatic phase (characterized by the emission of “wet” pyroclastic deposits), related to the interaction of the magmas with ground water, due to the emptying of the magmatic reservoirs (Sheridan et al., 1981; Barberi et al., 1989, 1990; Civetta et al., 1991c).
A similar repetitive behaviour can be recognized also in the overall dynamics of Somma-Vesuvius volcanism, which seem to have followed a very simple cyclic mechanism in which periods of quiescence (from hundreds to thousands of years), characterized by obstructed conduit conditions, were interrupted by plinian or subplinan eruptions. Interplinian activity was characterized by open conduit conditions, with small volume semipersistent strombolian-vulcanian activity plus frequent lava effusions and phreatomagmatic eruptions (Civetta and Santacroce, 1992; Rolandi et al., 1998). In addition, Civetta and Santacroce (1992) recognized a positive correlation between erupted volumes, degree of magma differentiation and length of repose periods. The same authors also related the obstruction of the conduit to the formation of large magma chambers, whose depth controlled the styles of eruptions. Reservoir structures developed within the Mesozoic carbonatic basement are associated with very long periods of quiescence (i.e., several centuries), followed by powerful plinian events. Conversely, magma chambers formed above the basement are related to shorter periods of repose (i.e., few centuries) and less voluminous subplinian eruptions. Open conduit activity is probably related to smaller (<0.5 km3) and shallower (1-2 km depth) reservoirs, constantly refilled by new magma batches (Civetta and Santacroce, 1992; Marianelli et al., 1995).
Figure 9. Geochronology of Somma-Vesuvius eruptions
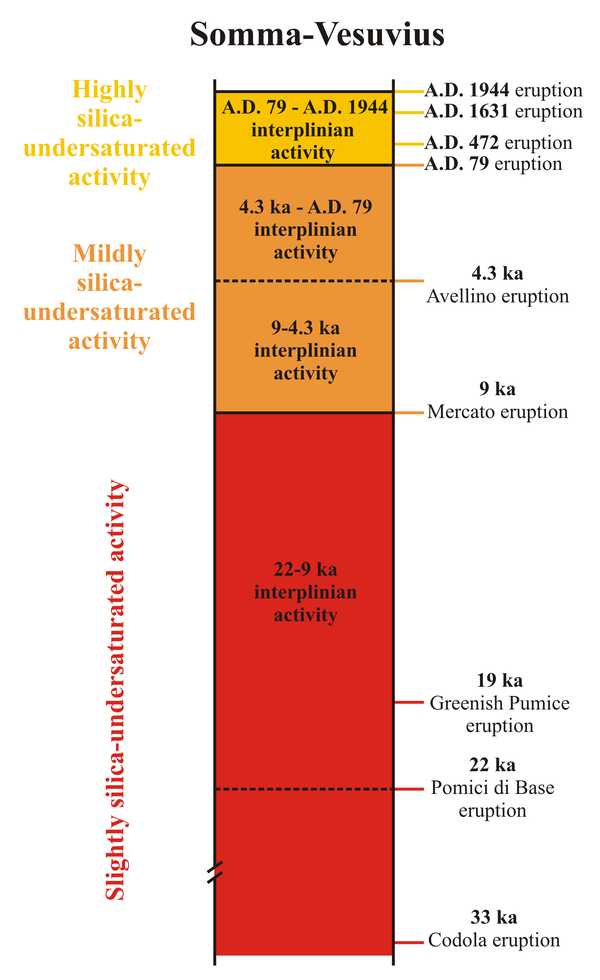
Chronological sketch summarizing the post-39 ka volcanism of the Somma-Vesuvius complex (from Santacroce et al., 2008), whith different colours indicating different magma compositions. Thick marks refer to significative eruptions. See text for a more detailed treatment.
Very little is known about the ancient Mt. Somma activity. The few available data are mainly restricted to samples recovered in the “Trecase 1” geothermal well, which indicate that Somma-Vesuvius volcanism probably started around 300-400 ka (Bernasconi et al., 1981; Principe et al., 1987; Brocchini et al., 2001) with the emission of tephritic-phonolitic and shoshonitic lavas. This very early phase of activity was probably followed by a long period of quiescence, which ended only after the CI eruption (i.e., ~39 ka), whose deposits represent a widespread marker horizon in the Somma-Vesuvius area. Most of the Mt. Somma products known, represented by the lava flows, spatter and scoria deposits presently observable along the inner walls of the caldera (Santacroce and Sbrana, 2003; Santacroce et al., 2008) and by pyroclastic deposits recovered in the Camaldoli della Torre borehole (Di Renzo et al., 2007), were emplaced after this event.
Several models have been so far proposed for the post-CI volcanological evolution of the Somma-Vesuvius (e.g., Johnston-Lavis, 1884; Rittmann and Ippolito, 1962; Lirer et al., 1973; Delibrias et al., 1979; Arnò et al., 1987; Civetta et al., 1991b; Civetta and Santacroce, 1992; Ayuso et al., 1998; Somma et al., 2001; Piochi et al., 2006; Santacroce et al., 2008). These schematizations differ from each other in many aspects, from the number of the recognized phases of activity, to the dating of the various eruptions, to the classification of each explosive event (e.g., as a plinian or a subplinian eruption), up to the name of a specific eruption. However, an overall concordance can be envisaged as regards the most important points, and therefore it is here chosen to refer to the most recent of these models, the one of Santacroce et al. (2008), reported in Figure 9.
Five major plinian eruptions characterized the Somma-Vesuvius activity (Santacroce et al., 2008): Codola [dated at ~25 B.P. by Alessio et al. (1974), calibrated at 33 ka by Giaccio et al. (2008)], Pomici di Base (or “Sarno”, ~22 ka; Capaldi et al., 1985; Andronico et al., 1995), Mercato (or “Ottaviano”, ~8-8.9 ka; Aulinas et al., 2007; Santacroce et al., 2008), Avellino (~4.3 ka; Santacroce et al., 2008) and the A.D. 79 [or “Pompeii”; e.g., Sheridan et al. (1981), Sigurdsson et al. (1985), Civetta et al. (1991b), Cioni et al. (1992), Lanphere et al. (2007)]. Except for the Codola eruption, for which the very few available data do not allow a thorough characterization, all these events have been widely studied and accurately described in all their volcanological and petrochemical aspects.
The Pomici di Base eruption is the oldest among the plinian caldera-forming events of the Somma-Vesuvius (e.g., Delibrias et al., 1979; Capaldi et al., 1985; Arnò et al., 1987; Andronico et al., 1995; Bertagnini et al., 1998; Landi et al., 1999). The eruption (~4.4 km3 of erupted volume) progressed basically following the above mentioned sequence, starting with a magmatic stage, which emplaced ash, pumice and scoria fallout deposits, and ending with a phreatomagmatic phase, during which lithic-rich pyroclastic fall deposits and flow deposits accompanied the caldera collapse. Interplinian activity following the Pomici di Base eruption was notably variable, alternating low magnitude eccentric flank eruptions, quiescent phases and subplinian events (e.g., the ~19 ka Greenish Pumice eruption; Delibrias et al., 1979; Ayuso et al., 1998; Santacroce and Sbrana, 2003; Santacroce et al., 2008).
The following plinian event was the Mercato eruption (e.g., Walker, 1977; Delibrias et al., 1979; Rolandi et al., 1993a; Cioni et al., 1999; Aulinas et al., 2007), again characterized by the usual progression from a magmatic fallout stage (the main phase, accounting for some ~90% of the total erupted material, on a total estimated volume of ~2-3 km3 of pyroclasts) to a phreatomagmatic pyroclastic flow stage. However, the Mercato eruption has shown two features which make it unique among the Somma-Vesuvius plinian eruptions, namely the homogeneous chemical composition of the products (whereas the other plinian eruptions all show a marked compositional zoning) and the poorly developed phreatomagmatic phase.
After the Mercato eruption, the Somma-Vesuvius entered a long quiescent period with no interplinian activity, interrupted only by the Avellino plinian eruption (e.g., Lirer et al., 1973; Arnò et al., 1987; Civetta et al., 1991b; Rolandi et al., 1993b; Cioni et al., 2000). The main particularity of this event (~1-2 km3 of erupted material), whose eruptive sequence still followed the common scheme of Somma-Vesuvius plinian eruptions, is represented by the sharp colour change of the pyroclastic fall deposits, changing upwards from white to grey. This has been interpreted as a clear evidence of a change in magma composition (e.g., Barberi et al., 1981; Civetta et al., 1991b; Santacroce et al., 2008). Interplinian activity following the Avellino eruption was characterized by a very complex sequence of events which are still a matter of very controversial interpretations (e.g., Rolandi et al., 1998; Somma et al., 2001; Andronico and Cioni, 2002).
The A.D. 79 eruption is the last and the best known of the Somma-Vesuvius plinian eruptions (e.g., Di Girolamo, 1963; Sheridan et al., 1981, Sigurdsson et al. 1982, 1985, Civetta et al., 1991b; Cioni et al., 1992, 1999; Lirer et al., 1995; Luongo et al., 2003a,b; Lanphere et al., 2007). The eruption is once more characterized by a transition between magmatic and phreatomagmatic phases, in which the plinian s.s. stage accounts for most of the erupted tephra (~2-4 km3 in volume). Like the Avellino eruption, a sharp transition can be observed between basal white pumices and apical grey pumices, suggesting magma stratification in the magma chamber.
The most recent period of activity of Somma-Vesuvius, which contributed to the edification of Vesuvius cone, is characterized by a complex alternation of periods of activity (with various explosive character) and quiescent phases [e.g., Santacroce (1987), Andronico et al. (1995), Somma et al. (2001), Santacroce et al. (2008)]. The period immediately after the A.D. 79 eruption was probably characterized by open conduit conditions, which resulted in very low-energy events mainly consisting in ash emissions (Andronico et al., 1995), suddenly interrupted by the A.D. 472 eruption [“Pollena”; Delibrias et al. (1979); Rosi and Santacroce (1983); Civetta et al. (1991b); Rolandi et al. (2004b)], the largest Somma-Vesuvius subplinian eruption known. After this event, a period of very variable “medieval” activity begun, alternating lava effusions, moderately explosive eruptions and repose periods (e.g., Santacroce, 1987; Rolandi et al., 1998; Principe et al., 2004), before a new sudden subplinian eruption occurred in 1631 [e.g., Rosi et al. (1993), Bertagnini et al. (2006)]. After this event, the volcano entered a new open-conduit phase (“modern” activity) characterized by semipersistent mild activity, minor lava effusions and short quiescent periods. Each of this periods of repose was preceded by relatively powerful explosive-effusive polyphased eruptions (e.g., Santacroce, 1987; Arrighi et al., 2001) like the 1944 event, the last eruption of the Somma-Vesuvius complex.
The Somma-Vesuvius products show alkaline potassic affinity (e.g., Santacroce et al., 1987; Peccerillo, 2005 and references therein) and range from slightly to strongly silica-undersaturated. Several authors have related the variability of silica undersaturation degree to the age of the products [e.g., Joron et al. (1987), Ayuso et al. (1998), Peccerillo (2005)], up to the most recent paper of Santacroce et al. (2008), which proposed the distinction of three groups of rocks: 1) slightly silica-undersaturated, older than the Mercato eruption (>8.9 ka); 2) mildly silica-undersaturated, from the Mercato to the Pompeii eruption (i.e., from 8.9 ka to A.D. 79); 3) highly silica-undersaturated, younger than the Pompeii eruption (<A.D. 79). Some exceptions to this schematization do exist, but they can be considered negligible. Regardless of the serial affinity, a correlation between eruptive style and rock compositions seems to exist, with a linear increase of the degree of differentiation moving from the least differentiated lavas through the more evolved pyroclasts from subplinian and plinian eruptions (the latter being characterized by the most evolved compositions; e.g., Joron et al., 1987; Civetta and Santacroce, 1992; Santacroce et al., 2008).
Somma-Vesuvius slightly undersaturated rocks range in composition from K-trachybasalt to trachyte, with moderately to poorly porphyritic textures dominated by plagioclase and clinopyroxene (plus olivine and leucite in the less evolved products, biotite, K-feldspar and amphibole in the intermediate/evolved ones) set into a holocrystalline to hypocrystalline groundmass (Fig. 10a). Rocks of the mildly undersaturated series are phonotephrites, tephriphonolites and phonolites, commonly showing strongly porphyritic textures (with a general decrease of the degree of porphyricity with increasing degree of evolution) with phenocrysts of plagioclase, clinopyroxene and leucite (plus K-feldspar and biotite in the most evolved tephriphonolites and phonolites) set in a groundmass formed by the same phases and glass (Fig. 10b). Rocks from the highly undersaturated series range from leucite tephrite to leucite phonolite and generally show strongly porphyritic textures characterized by phenocrysts of clinopyroxene plus minor leucite and olivine (absent in phonolites) set into a holocrystalline to hypocrystalline groundmass (Joron et al., 1987; Peccerillo, 2005; Fig. 10c-d).
Figure 10. Petrography of Somma-Vesuvius rocks.
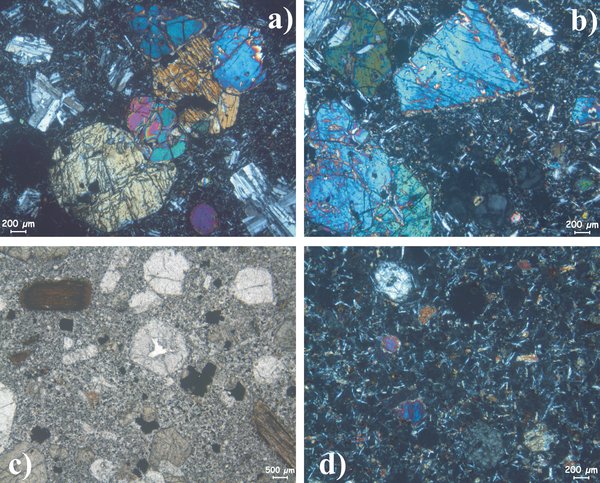
Microphotographs of some representative Somma-Vesuvius lavas: a) crossed polars view of a shoshonite from the slightly undersaturated series showing a strongly porphyritic texture with phenocrysts of clinopyroxene, plagioclase and olivine (bottom and center of the picture) with minor leucite microcysts in the groundmass (sporadic rounded extinct individuals); b) crossed polars view of a phonotephrite from the mildly undersaturated series showing a strongly porphyritic texture with phenocrysts of clinopyroxene, leucite and plagioclase; c) plane polarized view of a leucite phonotephrite from the highly understurated series showing a moderately porphyritic texture with phenocrysts of clinopyroxene, leucite, and biotite (right and left side of the picture); d) crossed polars view of a leucite tephriphonolite from the highly undersaturated series showing a moderately porphyritic texture with clinopyroxene and leucite phenocrysts set into a groundmass with diffuse feldspar microcrysts (sparse small laths).
The main chemical features of the most recurrent mineral phases of Somma-Vesuvius volcanics are briefly presented here.
Olivine. In highly undersaturated lavas olivine is present as rare microphenocrysts (Fo78-60) and as small groundmass individuals (Fo66-48), commonly showing extensive zonation. In slightly undersaturated rocks olivine occurs at any degree of evolution, ranging in composition from Fo75 (usually in the cores of crystals) to Fo40-45 (in the groundmass; Joron et al., 1987), showing a fairly regular Fe and Mn enrichment with increasing degree of differentiation.
Clinopyroxene. Clinopyroxene is an ubiquitous mineral in Somma-Vesuvius products. Three types of clinopyroxene crystals are commonly observed: diopside, salite and Al-Fe-rich diopside (with a general Al and Fe increase with increasing degree of differentiation of the host rock). Obscillatory, normal and sector zonings are very frequent. Clinopyroxene phenocrysts of the slightly undersaturated series have a moderately variable salitic composition (Wo47-44En41-48Fs12-15; Fig. 5) and commonly show lower Al2O3, higher TiO2 and lower Wo contents with respect to those from highly undersatured series (Joron et al., 1987). Groundmass individuals are usually Ti-enriched with respect to Al (Joron et al., 1987).
Plagioclase. Somma-Vesuvius plagioclase is generally characterized by strong An contents (even in the more evolved rock compositions), probably due to high temperatures of the melts or high fluid pressure during the crystallization (Joron et al., 1987). Compositional ranges are generally wide (e.g., An41-91Ab8-55Or1-8) essentially ranging from bytownite to andesine (Fig. 6).
K-feldspar. Sanidine crystals are confined to sialic pyroclastics and to the groundmass of the most evolved lavas. In latitic lavas sanidine shows more sodic compositions (up to Ab38), whereas in K-richest trachytes and phonolites sanidine crystals are more potassic (~Or85) and show very high Ba contents (up to 1.30 wt.%; Joron et al., 1987; Landi et al., 1999).
Leucite. Leucite is the most common feldspathoid of Somma-Vesuvius products, occurring almost in all volcanics from series of any degree of saturation, being absent only in the products of the older major plinian eruptions (Joron et al., 1987). Leucite crystals typically show significant silica excess with respect to the stoichiometric compositions, particularly evident in rocks from the slightly undersaturated series (Joron et al., 1987).
Mica. Biotite is very common in the most evolved rocks, with a magnesian character and a moderately high TiO2 content. Fe-richer varieties are confined to the groundmass of sialic products (Joron et al., 1987).
Opaque oxides. Crystals are mainly present as microphenocrysts or in the groundmass of all Somma-Vesuvius volcanics. In the products of recent Vesuvius activity (leucitites) they are present as titaniferous individuals, rich in ulvöspinel component (45-56%; Joron et al., 1987).
On the whole, the Somma-Vesuvius rocks display SiO2 contents between ~47 and 66 wt.%, CaO <14 wt.%, TiO2 <1.3 wt.% and MgO up to 7.5 wt.%. Rocks of the three series show very similar differentiation trends which, however, can be easily distinguished from each other on the basis of different contents or different slopes of some key-elements (e.g., TiO2, Al2O3; Na2O, K2O; Fig. 7). With increasing differentiation, a general decrease of TiO2, CaO, Fe2O3, MgO and P2O5 and an increase of SiO2, Na2O and K2O can be observed. Rb, Nb, Zr, Hf, Th, U, Ta, Pb and Yb are incompatible, Cr, Ni, Sc, and Cu are compatible (Fig. 8). REE patterns show a marked LREE/HREE enrichment. Throughs at HFSE and a Pb spikes are typical features of Somma-Vesuvius normalized diagrams (e.g., Joron et al., 1987; Peccerillo, 2005). The low MgO, Cr, Ni and Co contents indicate that the most “primitive” magmas do not represent primary mantle melts, but that fractional crystallization, with the removal of clinopyroxene, leucite, plagioclase and Ti-magnetite, probably occurred prior to their emplacement (Ayuso et al. 1998).
Fractional crystallization is generally thought to be the main evolutionary process, with the different trends of the three series being ascribable to the existence of different parental magmas (e.g., Joron et al., 1987) or to different ratios of clinopyroxene/feldspar fractionation due to variable crystallization pressures (Trigila and De Benedetti, 1993). In addition, petrographic, geochemical, isotopic and experimental evidences suggest that open-system processes such as magma mixing and assimilation of carbonate wall rock also played some role in the evolution of Somma-Vesuvius magmas (e.g., Savelli et al., 1967a,b; Civetta et al., 1991; Civetta and Santacroce, 1992; Cioni et al., 1995; Ayuso et al., 1998; Civetta et al., 2004; Piochi et al., 2006; Iacono Marziano et al., 2008).