The geomaterials in the Neapolitan architecture
The use of building materials of local origin in the Neapolitan area, as well as in many other Italian regions, has been and still is a common tradition, both for the normal building industry and for major structures. The use (or not) of these natural products through the centuries has been conditioned by several factors, including production costs, worker's skill levels and, in particular the choice of the changing architectural styles. Of the available materials, some of them passed these “tests” and were used extensively; however, it should also be noted that in a few cases, poor utilization has had a negative impact on the reputation of certain materials. This is sometimes due to the scarce knowledge from the users of the intrinsic properties of the materials - often a consequence of a lack of importance given to the characterization of the stone and to a lack of appropriate consultation with professional experts. In addition, a deeper knowledge of the mineralogical and petrophysical features of these materials can lead to better interpretation of the weathering processes and, on this basis, to improvements in heritage preservation and restoration projects.
A review of the main materials used in large buildings in Naples urban areas, mainly represented by the volcaniclastic products of the Campi Flegrei and, subordinately, by the Vesuvian and Phlegrean lavas, is presented in the following section with a detailed treatment of their mineralogical and physical-mechanical features as well as a thorough historical excursion on their employment as geomaterials and some remarks on the main weathering processes affecting them.
The Neapolitan Yellow Tuff (NYT)
Figure 11. Quarries in the Neapolitan Yellow Tuff.
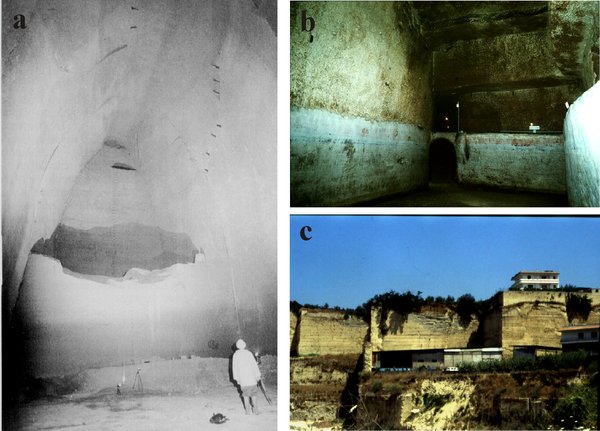
Different kinds of quarries in NYT formation. a) and b) underground quarries below Naples town; c) slope quarry in western Campi Flegrei.
The Neapolitan Yellow Tuff (NYT) represents the most used building stone in Neapolitan and regional architecture since Greek times. The deposits of NYT have been exploited for the production of building dimension stones throughout history. For many centuries, this activity was firstly concentrated and developed beneath the city of Naples (Fig. 11a and b), then in open pit quarries placed at the borders of the old town, such as those at Capodimonte, Rione Sanità, Fontanelle, Camaldoli, Petraio and Pizzofalcone. Only in the 16th century, as a consequence of the further urban development of Naples, a growing demand for material, along with the simultaneous forced reduction in urban exploitation, caused a shift to the western sector of Campi Flegrei which suddenly developed a large number of slope or trench quarries (Fig. 11c).
Figure 12. Mineralogy of the Neapolitan Yellow Tuff.
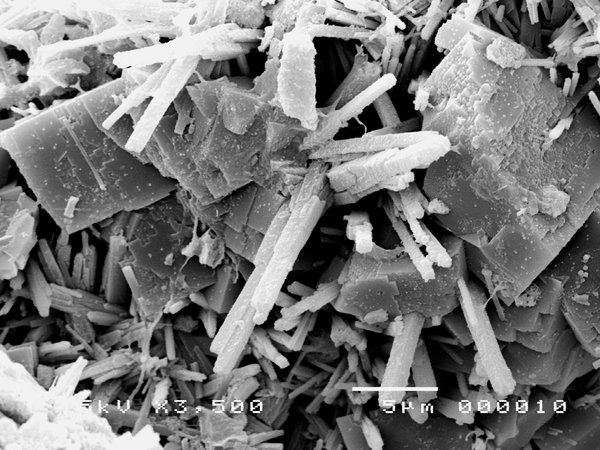
Authigenic minerals in NYT. Chabazite occurs as rombohedral crystals, phillipsite in acicular clusters. Sample from Marano (Naples).
Mineralogical composition of the NYT
The deposits of the NYT are characterized by both pyrogenic and authigenic phases, as a significant portion of these volcaniclastic products of this eruption (>50% in volume) was affected by diffuse zeolitization processes. Among pyrogenic minerals, feldspars prevail, with minor amounts of biotite and pyroxenes. On the other hand, zeolites such as phillipsite, chabazite and analcime (Fig. 12) are the most abundant authigenic phases, along with minor amount of smectite. Quantitative XRD evaluations (Table 1) show phillipsite as the prevailing zeolite of NYT followed by chabazite and, in minor but still significant amount, analcime. The mean content of these phases generally exceeds 50 wt.%, even though it can sometimes reach values of 70-80 wt.%. (de’ Gennaro et al., 1982; de’ Gennaro et al., 1990).
Table 1. Representative mineralogical analysis of NYT. Sample from Marano (Naples; Colella et al., 2009).
Smectite | Biotite | Feldspars | Phillipsite | Chabazite | Analcime | Pyroxene | TOT | Amorphous |
---|---|---|---|---|---|---|---|---|
(%) | (%) | (%) | (%) | (%) | (%) | (%) | (%) | (%) |
12 | 0.5 | 12 | 36 | 19 | 7 | tr. | 87 | 13 |
The genesis of the authigenic phases of the NYT has always been deeply debated, especially in order to clarify its possible relationships with the lithification of the deposits of member B of Scarpati et al. (1993). Many hypotheses, mainly based on mineralogical, volcanological and geochemical data, were formulated to interpret how the process developed. The first author who hypothesized a "lithification due to secondary processes developed in a prevailing glassy matrix and leading to the formation of zeolites" was Scherillo (1955), even though he could not analytically prove it. This hypothesis was successively confirmed by Sersale (1958). In the 1970’s many researchers tried to contribute further. Isotopic data suggested that the zeolitization process lasted at least 4000-5000 years (Capaldi et al., 1971). The stratigraphic relationship between the uppermost incoherent pozzolana facies and the underlying lithified tuff, together with the high reactivity of the trachytic glass, suggested a process due to an open hydrologic system (Hay and Sheppard, 1977) or a diagenetic process in continental environment (Sersale, 1978). Successively, the analogy between the composition of zeolites occurring in NYT and that of the volcanic glass (i.e., phillipsite and chabazite) was considered as proof of a genesis within an open hydrologic system (Passaglia and Vezzalini, 1985; Passaglia et al., 1990). The same process was reported by Scherillo and Scherillo (1990) which also considered the particular texture of pozzolana, here defined as “expanded pozzolana”, as a fundamental to enhancing the zeolitization process.
Different conclusions were proposed by other researchers over a period of about 20 years. Some hypothesized a strong relationship between the minerogenetic process and eruptive mechanism leading to the emplacement of the NYT formation (de’ Gennaro et al., 1982, 1987, 1990, 1995b). In particular de’ Gennaro and Langella (1996), based on the the different zeolitization systems proposed by Mumpton (1973) and Gottardi (1989), excluded that any of them could explain the NYT deposits. On the basis of these research efforts, it was thought that the zeolitization of NYT developed immediately after the eruption in a thermally well-insulated system with the presence of a hot aqueous solution of hydromagmatic origin. Vertical and horizontal variations of the degree of lithification are related to water content and temperature changes during the emplacement of the NYT. In particular, the decrease of lithification towards the top and the bottom of the sequence is attributed to a faster cooling of the deposit, thus inhibiting the zeolitization process. The lack of zeolitization in distal areas led the authors to hypothesize a limit to the thickness of the deposit under which the heat dispersion is so fast as to override the zeolitization process. Finally, the lack of authigenic feldspar indicates that the minerogenetic process was interrupted, thus hindering the evolution of the system towards more stable phases (de' Gennaro et al., 2000b).
Petrophysical features of the NYT
Table 2 summarizes the main petrophysical parameters of NYT. Bulk density values, as many of the following reported parameters, range in a quite wide range (10.2-14.1 kN/m3) as a consequence of the high textural heterogeneity of the tuff (variable amounts and sizes of pumices, lithics, etc.). Apparent density shows a more limited range of values (22.06-22.65 kg/m3). Bulk and apparent density define this material as a light rock (Primavori, 1997).
Table 2. Main petrophysical features of NYT (Colella et al., 2009).
Dry density | Specific gravity | Open porosity | Imbibition coefficient | Compressive strength (UCS) | Ultrasonic velocity |
---|---|---|---|---|---|
(kN/m3) | (kN/m3) | (%) | (%) | (MPa) | (m/s) |
10.20-14.10 | 22.06-22.65 | 39.50-63.20 | 32.51-45.77 | 0.7-11.9 | 1602-2267 |
Total open porosity is definitely high in a wide range of values (39.5-63.2%) thus determining a high attitude of this material at adsorbing water due to the occurrence of a thick net of interconnected pores. Again, this data variability is definitely due to the heterogeneity of the material whereas the high values of porosity should be related to the particular texture of the rock with abundant pumices and lithics set in a ashy matrix deeply transformed in aggregates of phillipsite and chabazite characterized by a microporous structure. The results of Hg-porosimetry are reported in Table 3 and Figure 13. As far as pore distribution is considered, NYT shows a bimodal distribution characterized by a first class, less represented, within the macropores and a second one above the meso-micropore field (0.01-1μm).
Table 3. Parameters determined by Hg-porosimetry on NYT (Colella et al., 2009).
Mean pore diameter (μm) | Specific surface (m2/g) |
---|---|
0.055 | 18.97 |
Figure 13. Porosity of the Neapolitan Yellow Tuff.
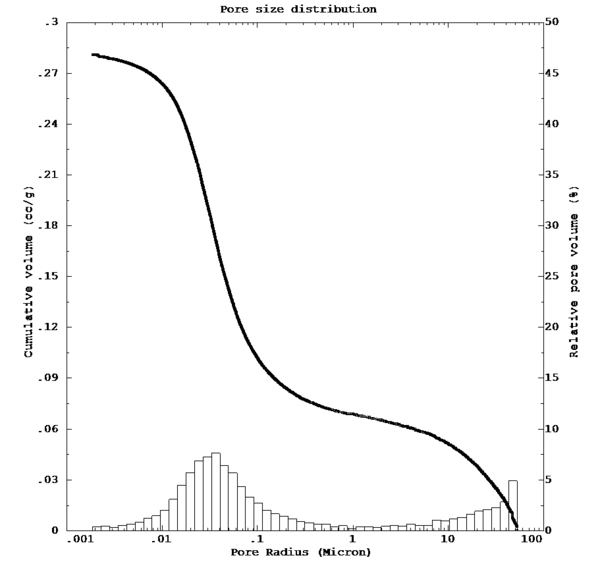
Pore size distribution obtained by Hg-porosimetry in a NYT sample [after Colella et al. (2009), modified].
Water absorption by total immersion tests revealed the high attitude of this material in allowing fluid circulation (Table 2) linked to the occurrence of a net of interconnected pores.
Uniaxial compressive strength values range between 4.1 and 6.65 MPa. This low and dispersed values are once again due to the particular heterogeneity of the rock and their textural features, among which porosity plays a relevant role (Evangelista and Pellegrino, 1990). The same features also account for the low and wide ranged ultrasonic velocities (Table 2).
The NYT as building material
The use of NYT as building stone is a thousand-year-old custom. As outlined above, its use is recorded since Greek times, mainly in columns and low-reliefs, but even older examples can be found in some Eneolitic Age tombs (about 4500 years ago). As previously stated, the exploitation was mainly underground, sometimes directly below the construction area. This procedure had some advantages - such as decreasing the cost of material transportation and preserving the surface ground from other anthropic activities. The extended network of underground galleries formed during many centuries beneath the old town was used for several purposes: worship places, rain water reservoirs, cellars or more recently, as air-raid shelter and today, they represent an evocative tourist stop (e.g., see http://www.napolisotterranea.org/. and http://www.lanapolisotterranea.it/). The urban development was strongly determined by the exploitation, thus creating a strong relationship between the urban growth on surface and the creation of a network of caves and tunnels underground (Cardone, 1993).
The abundance of NYT, along with its good physico-mechanical features and excellent thermal and acoustic coibentation properties (due to the high porosity and the abundance of zeolites in the matrix), resulted in extensive structural use of the yellow tuff - even though in many examples it was used as facciavista material (Fig. 14). The most relevant expressions are the Gothic Cathedrals (Basilica di S. Chiara, S. Domenico Maggiore, Cappella Pappacoda, etc.; Fig. 15), the Castel dell’Ovo (Fig. 16) and the Castel Sant’Elmo. Also worth noting are the underground works such as the cripta neapolitana, the grotta di Cocceo, the Volla aqueduct, etc.
Figure 15. Neapolitan stones and monuments.
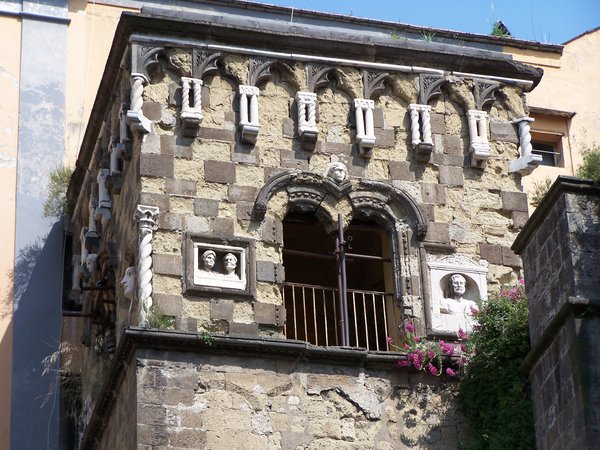
Belltower of Pappacoda Chapel (1415, restored in 1772) made with NYT, Campanian Ignimbrite and Piperno.
Its use continued in the following centuries in some important buildings such as the Accademia delle Belle Arti (1864), including today as the facades of the municipal swimming pool in Via Consalvo and many other public and residential houses built during the post-earthquake period.
The most successful period in the use of the “nude tuff” in Neapolitan architecture was the Angevin age, as in several gothic style churches such as those of S. Domenico Maggiore (Fig. 17), Santa Chiara (Fig. 18), S. Lorenzo Maggiore, etc. At that time, the Phlegrean stone was being accurately cut, thus providing peculiar architectural elements (arches, rose-windows, capitals, carvings). The choice of NYT as the predominant material was mainly dictated by artistic and architectural preferences, and was not due to needs deriving by economic crises or by autarchic recess. In fact, the Angevin age was one of the most superb periods that Naples, capital of the reign, had ever experienced; thus, it would have been easy to provide even more valuable materials or by using bricks (Cardone, 1990).
Since the Aragonese age, in contrast, the use of “nude tuff” became rarer and rarer, and walls made in NYT in the new buildings were plastered or coated by slabs of other materials such as Piperno and marble. This was further enhanced by the characteristic of the tuff to adhere to the mortars due to its high surface porosity and roughness. Such adhesion is even stronger with pozzolanic mortars used in Neapolitan architecture, since pozzolana and tuff are the result of different volcanic processes starting from the same original product.
Figure 18. The Neapolitan Yellow Tuff as a building stone.
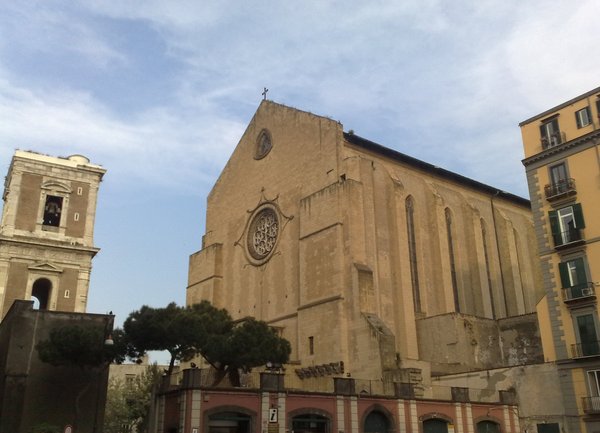
Use of the NYT in the S. Chiara Chatedral (1310-1340). Reconstructed after a fire in 1943 according to its original baroque style in 50s.
Weathering processes affecting the NYT
The mineralogical composition and the physical properties previously described make the NTY a geomaterial particularly susceptible to the action of the decay agents, as demonstrated by several studies carried out on some wall curtains of important Neapolitan monuments and by laboratory accelerated ageing tests.
In fact, all the facciavista surfaces are affected by numerous pathologies such as efflorescences, black crusts, alveolization, scaling, esfoliation which may lead in some cases to severe damage as a consequence of stone disaggregations. The basic agents of all these processes are definitely represented by capillary and surface waters which, by interaction with mortars and the zeolitic constituents of the rock (characterized by a high cation exchange capacity) define the settlement of different pH environments leading to the dissolution of zeolites which represent the cement of the tuff and the consequent tuff disaggregation (Fig. 19).
The Campanian Ignimbrite (CI)
Among local building stones used in the region, the Campanian Ignimbrite (CI) plays a significant role. As discussed in the “Geological outline” section, the CI eruption emplaced a complex and characteristic sequence of deposits, both in proximal and distal facies (e.g., Cappelletti et al., 2003; Fedele et al., 2008), including the Piperno, one of the most important building stones of the Neapolitan architecture. This section focuses only on the tuffaceous deposits of the CI, namely the grey WGI and the yellow LYT facies, reserving the following section to a more detailed treatment on the Piperno.
Figure 20. The Campanian Ignimbrite in a quarry.
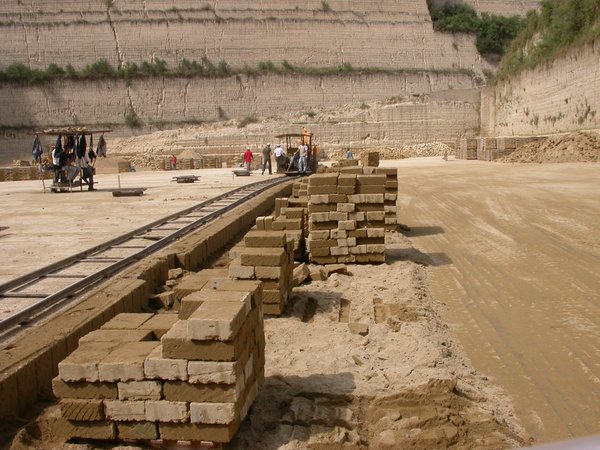
Outcrop of the CI in the yellow LYT facies used for the production of dimension stones (Comiziano, Naples).
Because of the abundance of these materials in Campania (Fig. 20 and 21) and their easy workability, they were used extensively in Campanian architecture since Roman times: many facades and/or elements (columns, etc.) of ancient Pompeii are made of tuff, often from the nearby town of Nocera. It has been often used facciavista in some relevant monuments such as the Mastio del Castello of Casertavecchia (CE), Castle of Manocalzati (AV), Cathedral of Sessa Aurunca, Basilica of S. Angelo in Formis and the Cathedral of Casertavecchia. The most highly exploited areas were located in the Caserta and in the Sarnese-Nocerino areas even though several quarries were identified over the whole region (Penta, 1935).
Figure 21. The Campanian Ignimbrite in the field.
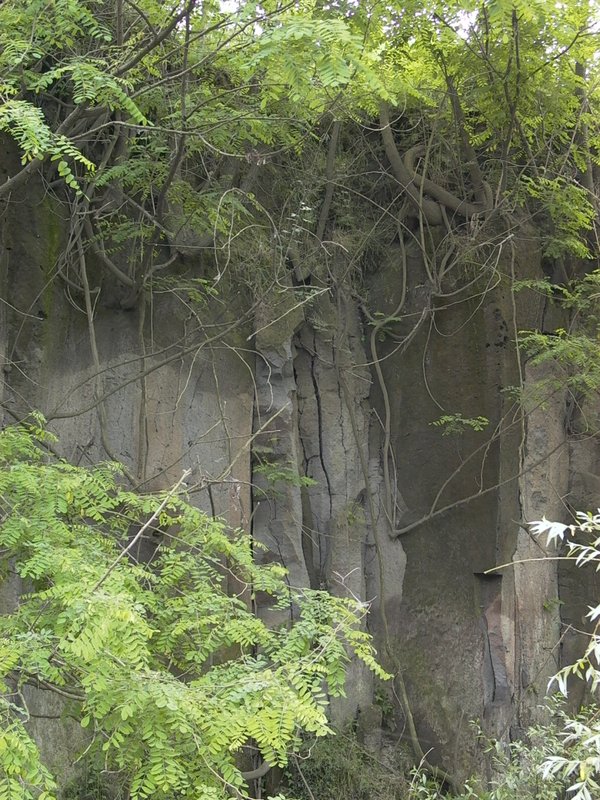
Outcrop of CI in the grey WGI facies showing typical columnar jointings (Tufara, Benevento).
Mineralogical composition of the CI
The most relevant WGI facies of the CI, historically referred to as the “Campanian Grey Tuff”, is mainly constituted by K-feldspar and plagioclase, together accounting for ~90% (Table 4). Clinopyroxene, biotite and hematite are definitely subordinate. Meionite was only recorded in some layers of the Sarnese-Nocerino district. The lithification process is mainly related to post-depositional devitrification phenomena leading to the crystallization of authigenic feldspar.
Figure 22. Mineralogy of the Campanian Ignimbrite.
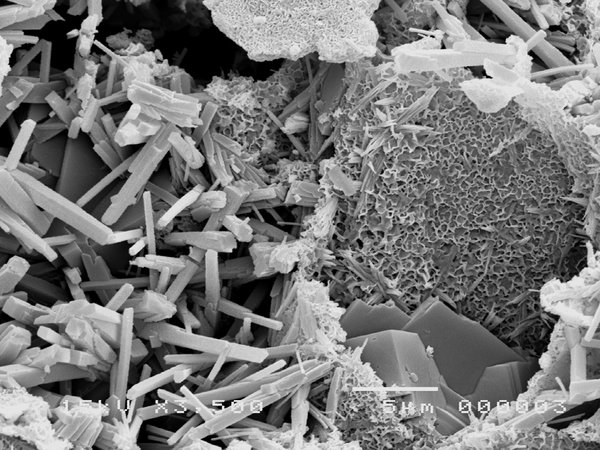
Phillipsite crystals along with smectite and chabazite in CI yellow LYT facies in a sample from Comiziano (Naples).
As regards the overling yellow facies (LYT), the most common mineral phases are represented by phillipsite and chabazite, commonly occurring in similar abundances (Table 4; Fig. 22), sometimes along with minor amounts of analcime and smectite (de’ Gennaro et al., 1987; Cappelletti et al., 2003). The genesis of such authigenic phases is probably related to interactions between infiltrating waters and the glassy matrix in a still hot system. Pyrogenic phases are feldspar (about 20%) and biotite in traces.
Table 4. Representative mineralogical analyses of CI grey (WGI, Piedimonte di Casolla, Caserta; Calcaterra et al,. 2004) and yellow facies (LYT, Comiziano, Naples; Colella et al., 2009). tr. = traces.
Smectite | Biotite | Feldspars | Phillipsite | Chabazite | Analcime | Pyroxene | TOT | Amorphous | |
---|---|---|---|---|---|---|---|---|---|
(%) | (%) | (%) | (%) | (%) | (%) | (%) | (%) | (%) | |
Grey facies | - | - | 90 | - | - | - | - | 90 | 9 |
Yellow facies | 8 | 1 | 17 | 30 | 25 | 2 | tr. | 83 | 17 |
Petrophysical features of the CI
Table 5 indicates some technical features of CI. Data for the grey WGI facies refer to a large sampling carried out on the most important outcrops of the formation, whereas those concerning the yellow LYT facies were collected on samples from a quarry in Comiziano (Naples). This explains the much higher variability of each single parameter in the grey facies and the quite homogeneous data in the yellow one.
Table 5. Physical parameters of CI in grey and yellow facies (WGI and LYT, Comiziano, Naples; Papa, 2010).
Dry density | Specific gravity | Open porosity | Imbibition coefficient | Compressive strength (UCS) | Ultrasonic velocity | |
---|---|---|---|---|---|---|
(kN/m3) | (kN/m3) | (%) | (%) | (MPa) | (m/s) | |
Grey facies | 10.40-13.40 | 22.26-25.90 | 50.40-58.60 | 42.23-52.28 | 1.1-7.1 | 1254-2981 |
Yellow facies | 10.20-12.00 | 23.10-23.40 | 49.10-55.40 | 28.50-30.40 | 6.6-8 | 1935-2090 |
Both facies are characterized by a high porosity associated with a strong tendency to absorb water, and quite low values of uniaxial compressive strengths that, analogous to NYT, are related to the heterogeneity of the rock and to its textural features. On these bases, the two facies are defined as weak, macroporous and light rocks (Primavori, 1997).
Table 6. Parameters determined by Hg-porosimetry on grey (WGI) and yellow (LYT) facies of CI (Papa, 2010).
Mean pore diameter (μm) | Specific surface (m2/g) | |
---|---|---|
Grey facies | 6.84 | 1.11 |
Yellow facies | 0.79 | 9.6 |
Figure 23. Porosity of the Campanian Ignimbrite.
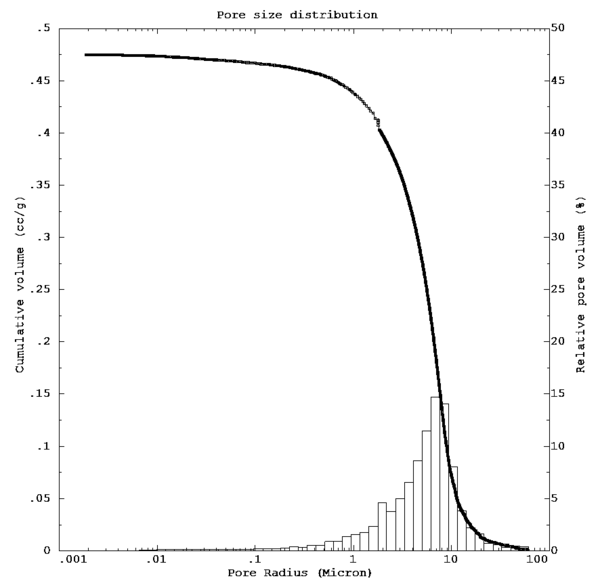
Pore size distribution for the grey facies (WGI) of CI (from Papa, 2010, modified).
Results of Hg- porosimetric tests are reported in Table 6. Both facies are characterized by an unimodal pore distribution (Fig. 23 and 24) falling in the macropore class. Mean pore diameter is for the grey facies one order of magnitude higher (6.84 μm) than that of the yellow one (0.79 μm). The higher value of specific surface recorded for the yellow facies is definitely linked to the presence of zeolites.
Figure 24. Porosity of the Campanian Ignimbrite.
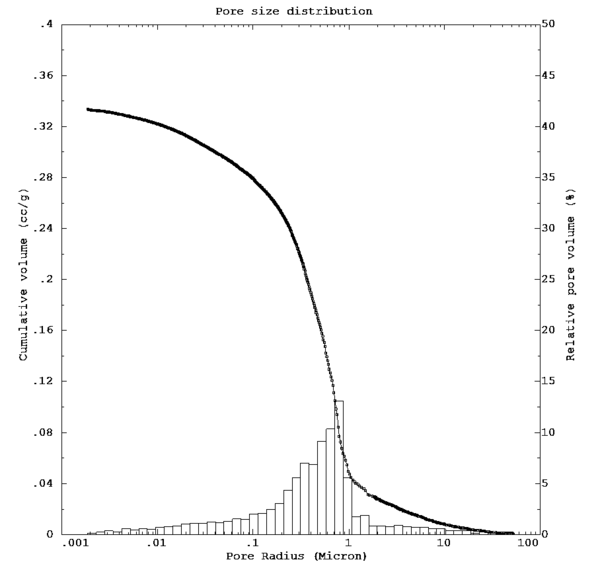
Pore size distribution for the yellow facies (LYT) of CI [after Colella et al. (2009), modified].
The CI as building material
The widespread occurrence of this product on the Campanian territory along with its good technical features and easy workability made this volcanoclastite one of the most used building stones in the Campanian architecture (Cardone, 1990; de’ Gennaro et al., 1995a).
The main sources are located in the Caserta province and in the Sarnese-Nocerino district, even though several quarries have been identified in the region. The yellow LYT facies, while still being requested and appreciated as a building stone due to its technical features and widespread field occurrence, never had a relevance comparable to that of the grey WGI facies in the historical architecture. As far as the Casertavecchia Mastio is considered, recent studies show some minero-petrographical differences thus giving rise to some doubts about the provenance of this tuff (Calcaterra et al., 2004). Few other examples of architectural works are found where this material was used without a plaster coating.
Figure 25. The building stones of the ancient Caserta.
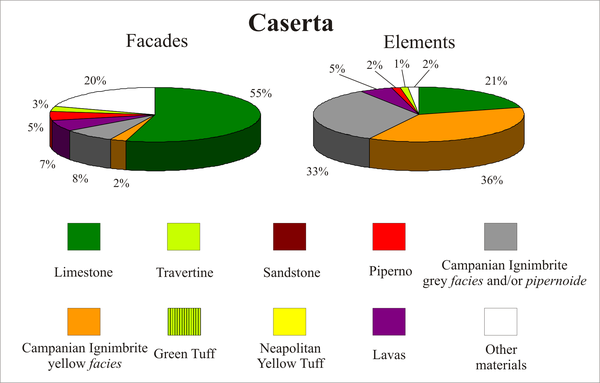
Distribution of stone materials in Casertavecchia [after Calcaterra et al. (2003a), modified].
Figure 26. The Campanian Ignimbrite in the Campanian monuments.
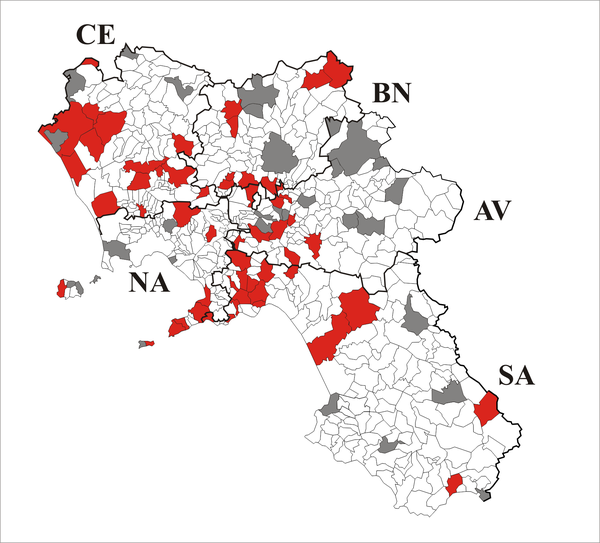
Distribution of Campanian municipalities (red coloured) where CI was recorded in some relevant monuments [after Calcaterra et al. (2003a), modified].
The grey facies, due to its easy workability and better petrophysical features, has been frequently used in particular architectural elements. Valuable examples of uses of this material as facciavista are widespread on the region; however, a particular concentration was recorded in the Caserta province (Fig. 25 and 26). Former examples date back to the 7th century B.C. with the Matres Matutae, votive sculptures symbolizing fertility and abundance, currently located in the Archaeological Museum of Capua; Campanian Ignimbrite, alone or along with other materials was therefore one of the first building materials since Roman times, as testified by the ruins of theatres, bridges, tumbs and the city walls of the old Suessa (today Sessa Aurunca), Pompei, S. Maria Capua Vetere and Treglia (Caiazza, 1986; Villucci, 1980). The popularity of this material, due to its color hues and texture, is admirably expressed in the Cathedrals of Sessa Aurunca, S.Angelo in Formis and Casertavecchia (Fig. 27).
Figure 27. The Campanian Ignimbrite as a building stone.
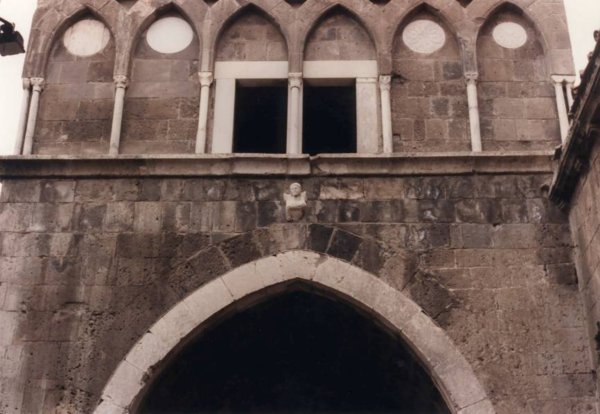
Belltower of the Cathedral in Casertavecchia made in the grey facies of CI (WGI) and marble.
During the Gothic period (12th - 15th century) the CI was being largely used as load-bearing structure but, at the same time, it started to be used to make decorative elements. Nice examples can be found in the towers of Federico II in Capua, Fieramosca building and Antignano palace in Capua and in the Church of Annunziata in Carinola (Caserta), the latter in gothic-catalan style (Robotti, 1983; D’Angelo, 1958). It cannot be disregarded, in addition, the wonderful decorative effect obtained by combining the grey tuff with other materials (travertine and bricks) in the medieval quadriportico of the Salerno Cathedral (Fig. 28).
Figure 28. The Campanian Ignimbrite as a building stone.
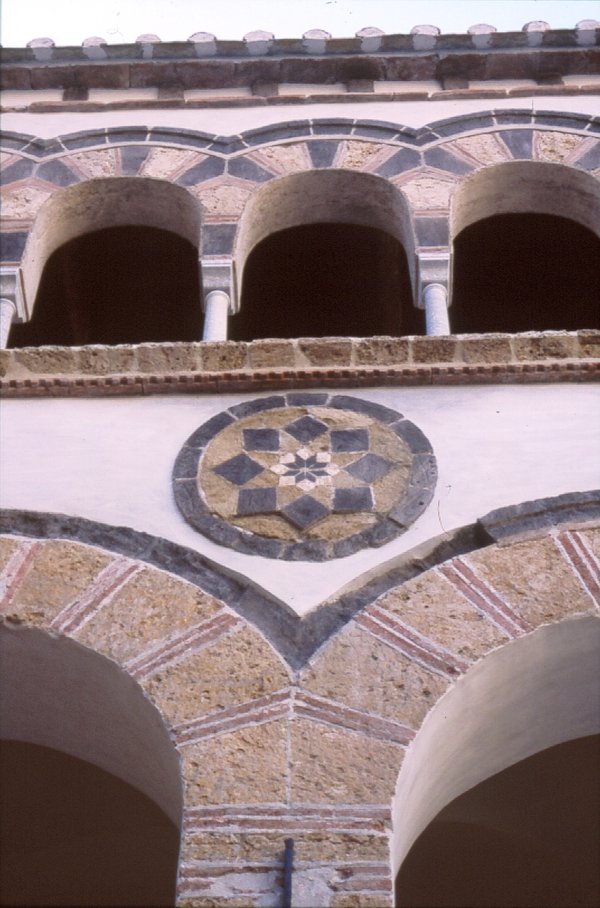
Detail of quadriportico of the Salerno Cathedral in grey facies of CI (WGI) with travertine and bricks.
Finally, the 16th century started the decline in the use of CI used facciavista, even though an outstanding example from this period is the Caroline aqueduct (close to Maddaloni) constructed by Vanvitelli (Maiuri, 1950).
Although its use was prevailingly local, the main example of exportation is to Naples where CI had a particular relevance mainly at the end of 19th century replacing the less available Piperno to produce architectural elements and less exposed portions of buildings (Fiengo and Guerriero, 1999; Calcaterra et al., 2000a). Possible areas of provenance of the material used in this period are those mainly located in the Nocerino-Sarnese area and, probably, in Puccianello (Caserta Province).
Weathering processes affecting the CI
The two facies of Campanian Ignimbrite, the welded WGI with prevailing authigenic feldspar and the yellow LYT deeply zeolitized, due to their different composition and texture, generally show different weathering forms. The grey facies is more sensible to the physical action of the decay agents, the yellow one to the chemical action of environmental factors (de’ Gennaro et al., 1995a).
Figure 29. Weathering of the Campanian Ignimbrite.
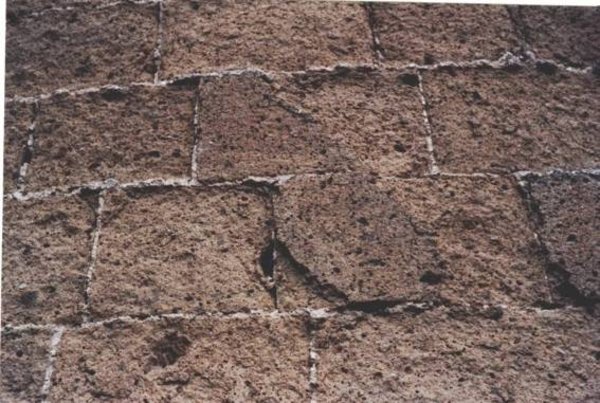
Casertavecchia Castle Mastio, example of disaggregation and alveolization in the yellow facies of CI (LYT).
For both facies the weathering is particularly evident when the stone is contacting different materials; alveolization, patinae, biological activity, scaling and disaggregation are almost always present (Fig. 30). Many facades show a weathering sequence such as: esfoliation → scaling → disaggregation. This final decay phenomenon will expose new fresh surfaces that will be affected by the same weathering. These decay forms are differently developed also as a function of the manufact exposure. Generally, esfoliation, efflorescences and disaggregation are more frequent in buildings south or eastward exposed whereas humidity traces and biological activity occur in those north and westward exposed (de’ Gennaro et al., 1995a; Fig. 29 and 30).
The Piperno
The Piperno is surely one of the most well-known and characteristic magmatic building stones in the Neapolitan area. This lithotype is exposed at the northern and southern foot of Camaldoli Hill, at Pianura and Soccavo (Torre Franco and Verdolino).
The term “Piperno” probably derives from the Latin piperinus used by Romans to indicate a particular kind of volcanic rock “lapis piperinus, seu albidus cum punctuis nigris, durus atque fortissimus” (Isidori Hispaniensis Episcopi, 1911). The only documented quarries are located in the above-cited areas of Campi Flegrei and are formed by tunnels branching under the hill (Carletti, 1787; Cardone, 1990). The formation seems to gently dip northward, but its base is not exposed. However, whenever visible, thickness is about 20 m (Perrotta and Scarpati, 1994; Rosi et al., 1996; Perrotta et al., 2006).
The origin of this deposit has been widely debated. The occurrence of the scoriae, called for the first time fiammae by von Buch (1867), led some authors to a very complex interpretation of the depositional mechanism as a lava flow. Since the beginning of the 20th century, many researchers have addressed their attention to this typical deposit. Dell’Erba (1892) and Zambonini (1919) related Piperno to a high temperature “pyroclastic cloud”. De Lorenzo (1904) defined it as a trachytic schlieren-lava. Rittmann (1950) and Gottini (1963) related it to a lava-lake activity. More recently, Fisher and Schmincke (1984) considered the deposit as a welded fallout tuff (agglutinate), whereas Rosi et al. (1996) defined Piperno as a pyroclastic flow deposit resulting from a substained activity. In the last years, some authors have differently interpreted Piperno in a wider volcanological context.
Field features are also debated. De Lorenzo (1904) described two layers of Piperno interspersed by breccia layers. Maggiore (1936) accurately described six layers characterized by very different technical properties. These layers have been exploited over different ages. On the other hand, Rosi et al. (1996) identified four layers of eutaxitic tuff, interspersed by loose lithic-rich breccias beds. At this time the outcrops are barely accessible and the entrance to the underground quarries are almost hidden.
Among the main Piperno outcropping areas, the section located at Masseria del Monte (Pianura, western side of Camaldoli Hill; Fig. 31) is the most complete in terms of vertical exposure, whereas the other investigated sectors (Piccola Lourdes, Pianura- western side; Verdolino, Soccavo-southern side of Camaldoli Hill) only provide partial information. In addition, data from drilling cores carried out on the slope of the hill for other purposes, gave an overall indication of the possible thickness of the Piperno formation.
Figure 31. Stratigraphy of the Piperno.
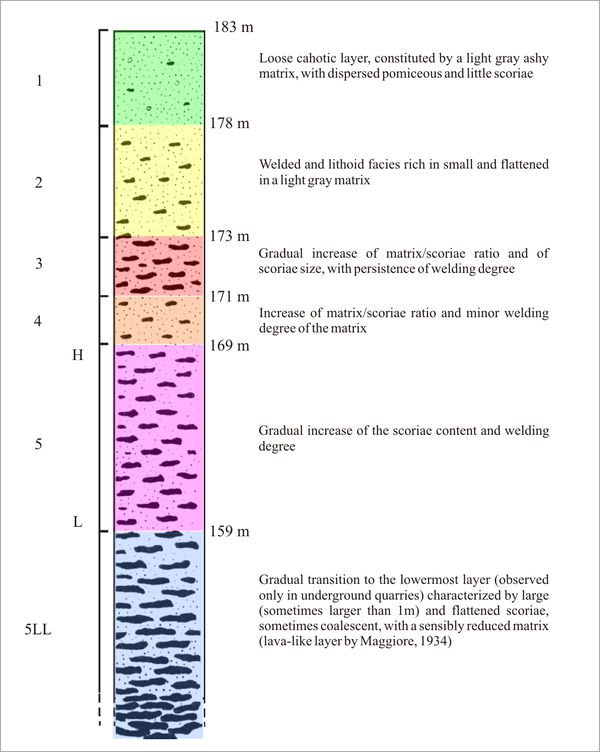
Reconstructed lithostratigraphic sequence of Piperno formation [after Calcaterra et al. (2005), modified].
One of the main aims of the studies on Piperno is to rediscover its exploitation sites, located at the foot of the Camaldoli hill, in Naples. In this view, a preliminary investigation showed that, among the main historical underground quarries, the one located in Pianura (Masseria del Monte) was the only accessible for the required studies. The study of the Masseria del Monte site started with a topographical survey (Fig. 32) carried out following the standard techniques adopted in a speleological context. The final report of the survey enabled the editing of a 1:200 scale map and of a relevant number of longitudinal and transversal sections. Finally, the main joints, reporting their dip direction, persistence, the width and possible filling materials, were also surveyed.
Figure 32. Quarry in the Piperno.
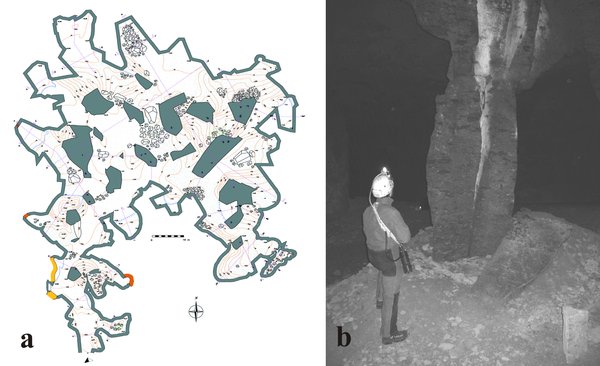
Masseria del Monte, Pianura (NA), map of the cavity (a) and cavity pillar, with evident fissural crack (b).
Mineralogical composition of the Piperno
Piperno is characterized by an eutaxitic texture with black flattened scoriae (fiammae) set in a hard and light grey matrix. At a macroscopic scale (Fig. 33) Piperno shows centimeter-to decimeter-sized fiammae showing a maximum length of 30-40 cm and an average flattening ratio of 1:10.
The main phases are sanidine, subordinate plagioclase, clinopyroxene ranging from diopside to salite, biotite, amphibole, magnetite and sodalite. These phases are set in a totally recrystallized matrix, where alkali feldspar represents the neoformed phase (Calcaterra et al., 2000b, c). Fiammae are also recrystallized by tiny new crystals of alkali feldspar with the same composition as those of matrix. Table 7 reports the results of a mineralogical quantitative evaluation carried out on representative samples of Piperno from Pianura and Soccavo. For both groups of samples the prevailing phase is sanidine ranging between 89% and 95%. Subordinate amounts of sodalite and magnetite were also recognized. The only Pianura sample shows a residual fraction of unreacted glass (about 5.5%). Only a very limited portion of feldspar can be ascribed to a primary genesis, most of it deriving from devitrification processes (vapour phase crystallization) which involved the glassy fraction occurring both in the matrix and scoriae. These processes led to significant lithological changes. The large glassy scoriae as well as the matrix lost their primary features thus becoming hard and compact as a consequence of welding and/or feldspar crystallization that also reduce the available pore space.
Figure 33. Piperno rock samples.
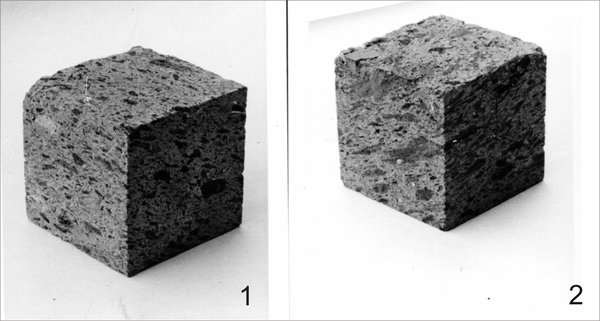
Specimens of Piperno showing the typical eutaxitic texture. Samples from Pianura (1) and Soccavo (2). Sample size: 71 mm [after Calcaterra et al. (2000c), modified].
Table 7. Mineralogical quantitative evaluation of representative samples of Piperno from Pianura and Soccavo (Calcaterra et al., 2000c). tr. = traces.
Feldspars | Sodalite | Magnetite | Biotite | Amphibole | Amorphous | |
---|---|---|---|---|---|---|
Pianura | 95.4 | 3.5 | 0.5 | - | tr. | 0.8 |
Soccavo | 89.3 | 3.9 | 1.5 | tr. | - | 5.4 |
The products of vapour phase crystallization in Piperno are alkali feldspars with a narrow range in chemical composition (Or53–34; Calcaterra et al., 2000b, c). Vapour phase crystallization results from hot gases passing up through the body of the deposit. Some fluids may be of juvenile origin, exsolved from pumice and vitric particles, and some may be from heated groundwater (Calcaterra et al., 2000b, c). These authigenic feldspars are observed in fiammae as well as in the matrix and their composition is distinguishable from the few phenocrysts present in the rock (Or60-53). The minerogenetic process seems to be confirmed by many gas-escape pipes present in the upper breccia (e.g. at Verdolino); these vertical channels testify to the wide degassing of the underlying Piperno unit. Electron microscopy observations (SEM) confirmed the above considerations and demonstrated the presence of feldspar crystals, with a typical tabular shape, growing on the glassy matrix (Fig. 34).
Petrophysical features of the Piperno
The characterization of the Piperno was formerly attempted bearing in mind the limited extension and the spot size distribution of the formation. Therefore, the entire set of data was handled as a unique population. This analytical approach was also dictated by the chemical and mineralogical homogeneity of the rock.
As far as physical properties are concerned, some representative parameters confirmed that all the analyzed samples can be treated as belonging to a single group. The example reported in Figure 35 shows a good correlation between dry and saturated ultrasonic velocities. However, most of the measured parameters (Table 8) show a marked variability as well; shown, for example, by the open porosity ranging between 12 and 50% (Fig. 36). This wide variability, mainly due to the heterogeneity of the material, can also be ascribed to the oriented texture of the collapsed scoriae (fiammae). In fact, some specific non-volumetric parameters (i.e., depending on measurement direction), such as ultrasonic velocities, display higher values after tests performed parallel to the elongation (Fig. 37), both in dry and saturated condition (+11% dry, +13% saturated). The same evidence results from the UCS tests, which, on average, show 23.4 MPa and 29.6 MPa for normal and parallel measurements, respectively.
Table 8. Main petrophysical features of Piperno (Calcaterra et al., 2005).
Dry density | Specific gravity | Open porosity | Imbibition coefficient | Compressive strength (UCS) | Ultrasonic velocity |
---|---|---|---|---|---|
(kN/m3) | (kN/m3) | (%) | (%) | (MPa) | (m/s) |
12.95-22.59 | 25.18-26.12 | 12.03-49.90 | 8.66-27.77 | 4.7-67.5 | 2229-3239 |
Figure 35. Technical features of the Piperno.
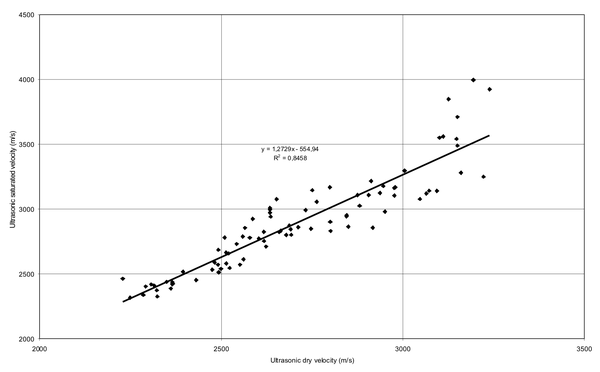
Dry vs. saturated ultrasonic velocity for Piperno [after Calcaterra et al. (2005), modified].
Figure 36. Technical features of the Piperno.
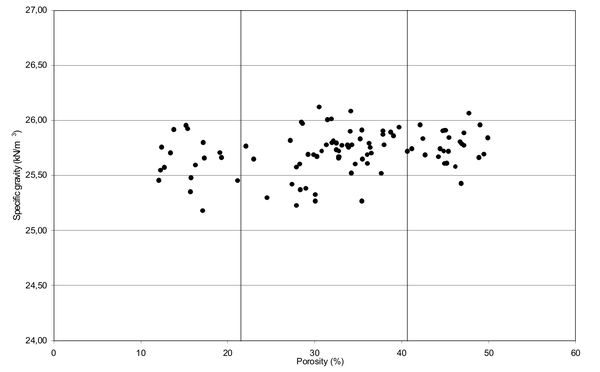
Porosity vs. Specific Gravity for Piperno [after Calcaterra et al. (2005), modified].
Figure 37. Technical features of the Piperno.
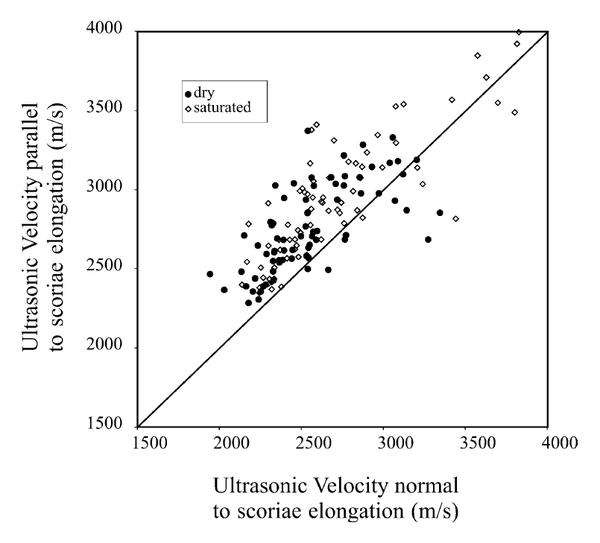
Ultrasonic velocities measured normal and parallel to the elongation, both in dry and saturated condition for Piperno [after Calcaterra et al. (2005), modified].
A tentative explanation of this behaviour, that requires further investigation (i.e., by integrating these tests with image analyses), is given by the role played by the morphology of the fiammae and their dimension. In fact, it is similarly hypothesized that the direction normal to the scoriae flattening axis “intercepts” a higher amount of denser material (fiammae) thus, deeply influencing the measured parameter. The above reported considerations led Calcaterra et al. (2004) to revise the petrophysical results taking into account also the lithostratigraphical reconstruction, hence coupling the laboratory data to the aforementioned layers.
In fact, a first confirmation of the relevant role played by the scoriae/matrix ratio is given by an image analysis carried out on samples collected from layers 2, 3/5H and 5L (Fig. 38). Mean values of the scoriae/matrix ratio show a remarkable and progressive increase from layer 2 to layer 5 (maximum difference ranging between 60 and 65%). The wide variability of the textural features is also shown by other experimental relationships such as Uniaxial Compressive Strength (UCS) versus Dry Density (Fig. 39), UCS versus Dry Ultrasonic Velocity (Fig. 40) and Porosity vs. Dry Specific Gravity (Fig. 41). In all graphs three distinct clusters, with a progressive improvement of petrophysical features (2<3/5H<5L) can be identified. Moreover, layers 2 and 3/5H, characterized by UCS values <25 MPa, can be classified as weak rocks whereas layer 5L falls within the field of the hard rocks (Hawkins, 1998).
Figure 38. Technical features of the Piperno.
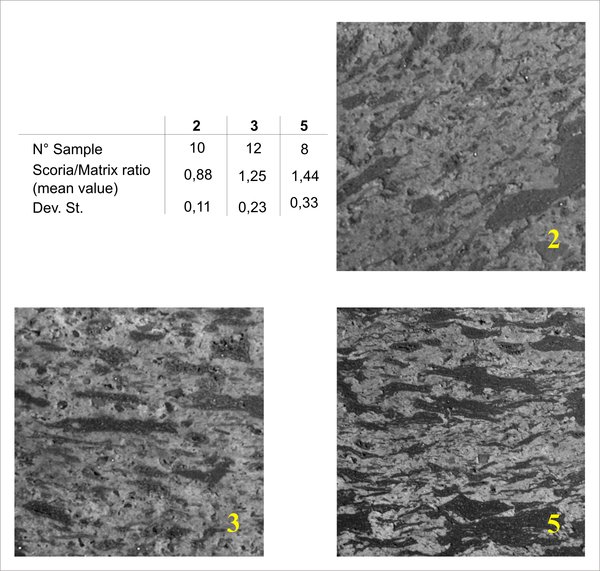
Scoriae/Matrix ratio for the main layers (2, 3 and 5) of Piperno exploited as ornamental stone [after Calcaterra et al. (2005), modified].
Figure 39. Technical features of the Piperno.
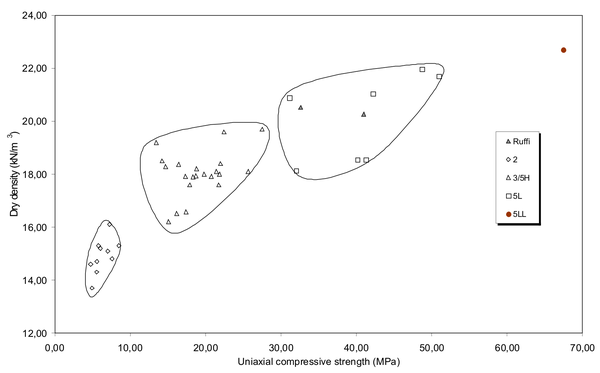
Uniaxial Compressive Strength vs. Dry Density for Piperno samples collected in the field and on monuments [Ruffi = Church of S. Giuseppe dei Ruffi; after Calcaterra et al. (2005), modified].
Figure 40. Technical features of the Piperno.
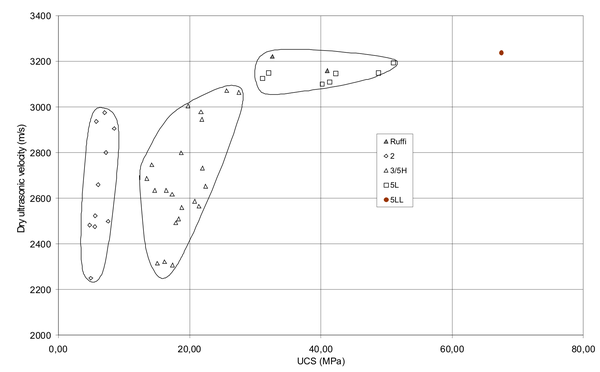
Uniaxial Compressive Strength vs. Dry Ultrasonic Velocity for Piperno samples collected in the field and on monuments [Ruffi = Church of S. Giuseppe dei Ruffi; after Calcaterra et al. (2005), modified].
Figure 41. Technical features of the Piperno.
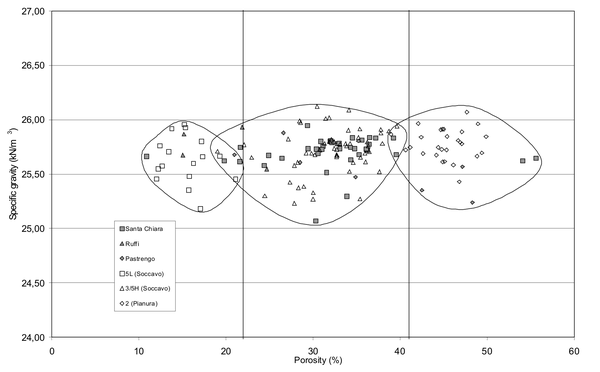
Porosity vs. Dry Specific Gravity for Piperno samples collected in the field and on monuments [Ruffi = Church of S. Giuseppe dei Ruffi; Santa Chiara = Santa Chiara Belltower; S. Anna = Monastery of S. Anna dei Lombardi Complex; after Calcaterra et al. (2005), modified].
Figure 42. Technical features of the Piperno.
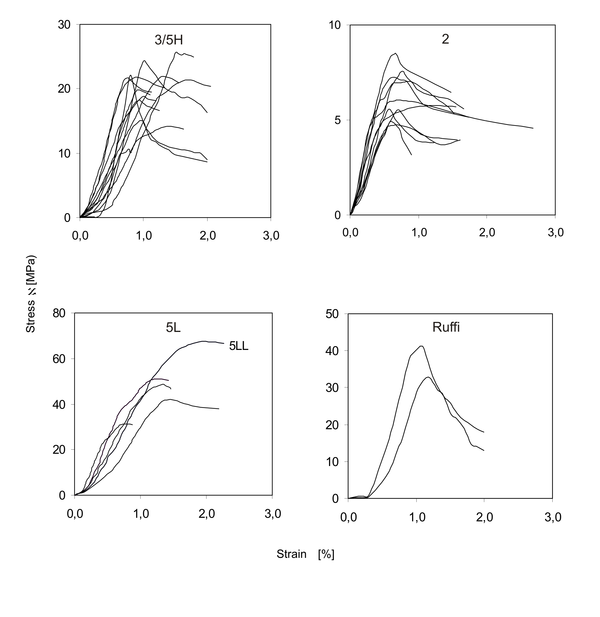
Stress-strain diagrams for samples collected in the field (layers 2, 3/5H, 5L) and on monuments [Ruffi = Church of S. Giuseppe dei Ruffi; after Calcaterra et al. (2005), modified].
The stress/strain curves of Figure 42 also show some differences in terms of elastic behaviour for layers 2, 3/5H and 5L. On the whole, all the varieties of Piperno should be defined as non-elastic rocks (Farmer, 1968), since they display an initial concavity due to closing of excess pore space or microcracks, and a terminal plastic zone approaching a level of failure strain. Adopting the classification proposed by Deere and Miller (1966), based on the modulus ratio (i.e. the ratio between tangent elastic modulus and compressive strength) all the materials would fall in the Low Modulus Ratio field (<200:1), with a very low degree of stiffness, thus indicating a distinct tendency to non-elastic deformation.
The Piperno as building material
Notwithstanding the fact that the most widespread dimension stone in Neapolitan architecture is the NYT, this material is often protected by plaster, so that Piperno ends up as the most diffused stone used facciavista (i.e., about 71,000 m2 out of 130,000 m2 of surveyed natural building stones; de’Gennaro et al., 2000a). Historical sources (Cardone and Papa, 1993) testify to quarrying in the rural village of Pianura (nowadays an urban district of Naples) since the 13th century. At that time, under the Angevin kings, Piperno, along with the NYT, represented the most used building stone for some of the most outstanding monuments that are still today a marker in the urban setting of Naples. These include Santa Chiara Church, San Domenico Maggiore Church and the San Pietro a Maiella Church. Further proof of the importance that this quarrying gained with time is given by the name of Soccavo (in Latin, sub cava = near the quarry), another village located at the foot of the Camaldoli hill. Under the Aragonese domination (15th century) the demand for Piperno greatly increased, as a consequence of its use in the main buildings of that time: the Gesù Nuovo Church (Fig. 43), the renovation of Maschio Angioino Castle (Fig. 44), the Royal Palace, the Sanseverino Palace, Cuomo Palace (Fig. 45).
The importance of the stone also led to the creation of a specific guild of workers (pipernieri), which increased in importance from the 15th to the 18th century. The exploitation of Piperno continued mainly through the exploitation of underground quarries at Pianura, Soccavo and Verdolino. The environmental conditions were, however, very dangerous and, on 22 October 1739, 11 miners died as a consequence of a vault collapse while working in one of the underground quarries (Cardone and Papa, 1993).
Figure 43. The Piperno as a building stone.
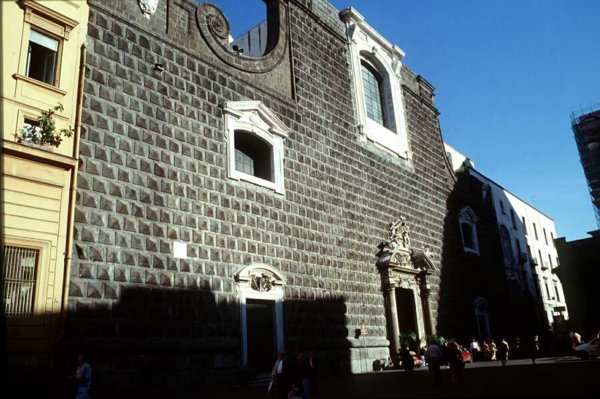
Gesù Nuovo Church. The façade of this building (begun in 1584 and completed in 1601) is of Piperno ashlar.
Figure 44. The Piperno as a building stone.
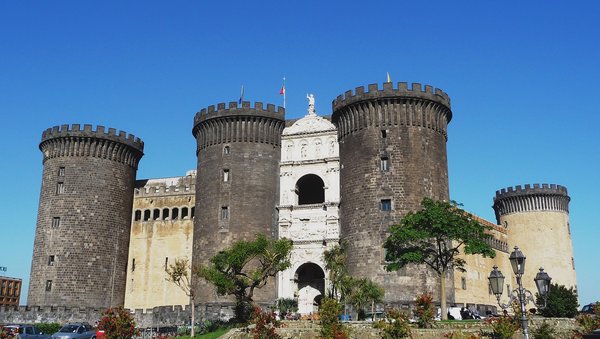
Castel Nuovo, also known as Maschio Angioino built in 1279. The current appearance was taken between 1443 and 1458.
From the 18th century onwards, Piperno was progressively replaced by less expensive materials, such as lavas of the Phlegrean and Vesuvian districts that are now seen in many buildings of that period in Naples and other Campanian towns. However, the Piperno quarries of Pianura remained active until the first decades of the 20th century. Today, the textural imprinting of Piperno, when used as a dimension stone in modern buildings, is improperly replaced with a similar volcanoclastic rock coming from the Viterbo area (Lazio region), known as “peperino”.
Weathering processes affecting the Piperno
A first evaluation of the weathering intensity of the Piperno was provided by de' Gennaro et al. (2000a), which expressed it as high, moderate and negligible. About 95% of the exposed surfaces was affected by moderate to high weathering grade.
Figure 46. Weathering of the Piperno.
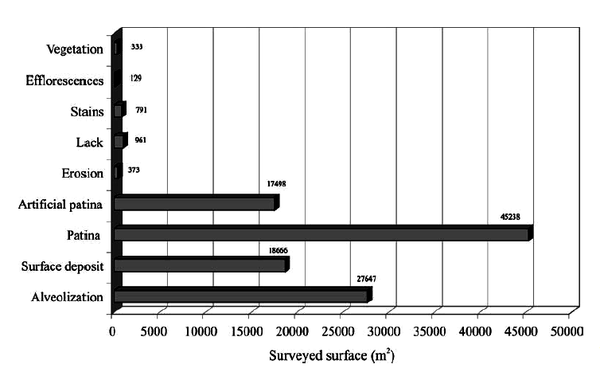
Distribution of different weathering typologies surveyed on Piperno from monuments of ancient centre of Naples [after Calcaterra et al. (2005), modified].
The survey carried out in the following research studies (Calcaterra et al., 2004) provided further knowledge on the weathering of Piperno, both in terms of intensity and typology. The weathering typologies were described according to Normal 1/88 (1988). About 87% of the investigated façades and elements is affected by high weathering. Patina, both natural and artificial, is the most diffused weathering typology, followed by alveolization and surface deposits (Fig. 46). Minor typologies are lacks, stains, erosion and vegetation.
Some examples of the most important weathering typologies are reported in Figure 47. Patina (Fig. 47a) produces an almost total annealing of the chromatic contrast between matrix and scoriae, thus giving to the whole surface a uniform dark grey colour. The artificial patina prevails in the lower portions of the buildings, mainly due to vandalism (Fig. 47b). The surface deposits are enhanced in buildings characterized by portions projecting from façades (Fig. 47c). Finally, alveolization (Fig. 47d), favoured by the particular texture and heterogeneity of the rock, and disaggregation affecting monuments exposed to marine aerosol (Fig. 47e), where mechanical effects of salt crystallization are more pronounced, are a clear evidence of the narrow relationships between weathering typologies and microenvironmental conditions.
Figure 47. Weathering of the Piperno.
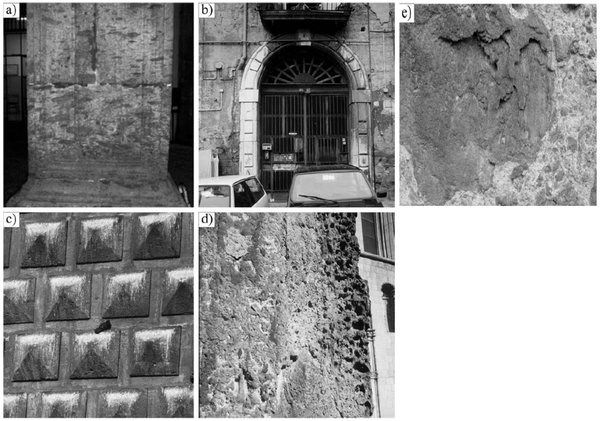
Main weathering typologies on Piperno surface from monuments. a) patina; b) artificial patina; c) surface deposits; d) alveolization; e) disaggregation [after Calcaterra et al. (2005), modified].
Vesuvian and Phlegrean lavas
Vesuvian lavas used in the architecture of the Neapolitan area belong mainly to the recent historical activity, namely between A.D. 1631 and 1944. Lava flows belonging to this period are located just in the southern sector of the complex and depart from the highest slope of the volcano. In some instances, they reach the sea. Most of the exploitation areas are located in this sector of the Vesuvius.
As far as petrophysical properties of this rock are concerned, literary evidence available is rare and often contradictory. Also, the historical data on the exploitation activity is scarce and incomplete. According to Fiengo (1983), in the middle of the 8th century about ten quarries were still active in an area located around Somma-Vesuviana, Terzigno, S. Giorgio a Cremano, Torre del Greco, Torre Annunziata, Granatello (Portici), and Resina (Ercolano). All these exploitation sites provided a very tough material, particularly suitable for flag-stone roads. The same author, starting from the data of Maggiore (1936), reports that one century later, a few decades before their definitive falling off, the exploitation sites became 74, in most of which lavas emplaced after the 1631 eruption were quarried. Only the paper by Penta and Del Vecchio (1936) reports a complete list of the main quarries (both active and abandoned) occurring on the Vesuvian territory. Most of them, however, were likely placed on old quarry fronts. Among all those reported, only a few can be still recognized and just a couple are active, at the sampling time, as a consequence of the intense urbanization of the area (Fig. 48). The exploitation activity was mainly concentrated in three sectors characterized by important lava outcrops. In the eastern sector, in Terzigno and Boscoreale territory, three sites were identified: the Vitiello quarry, likely representing the old De Medici quarry as reported by Penta and Del Vecchio (1936), which gave the so-called “Mauro lavas” from the flow activity of 1754. The other two sites of this sector, the D’Oriano and Ranieri quarries, are placed on the same lava flows.
The most important exploitation site of the whole Vesuvian district is located in the southern sector of the volcanic apparatus, within the urban limits of Torre del Greco. Known as the “Villa Inglese” quarry, this site was active up to the first half of the ‘70s. Two superimposed lava flows separated by a paleo-soil have been deeply exploited: the upper horizon was attributed by Penta and Del Vecchio (1936) to the A.D. 1760 eruption, whereas the lower one was ascribed alternatively to a presumed effusive event linked to the A.D. 1631 explosive eruption (Penta and Del Vecchio, 1936; Vittozzi and Gasparini, 1964; Rapolla and Vittozzi, 1968) or to older phases of activity (Arnò et al., 1987; Principe et al., 1987). In the same town of Torre del Greco, NW to Villa Inglese quarry another important site named ”La Scala” set out on a lava flow that, according to Penta and Del Vecchio (1936), belongs to the aforementioned presumed effusive event of A.D. 1631.
Figure 48. Vesuvian lava quarries.
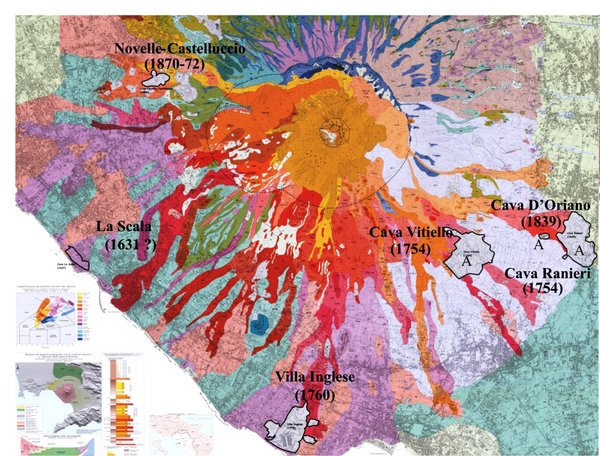
Location of the Vesuvius lava exploitation sites; in brackets the activity age [Somma-Vesuvius Geological Map 1:15,000, from Santacroce et al. (2003)]. A = active quarry (in 2002).
In the north-western sector of the Vesuvian area (Ercolano and Somma-Vesuviana) four inactive quarries are present. They can be considered as historical sites quarrying two superimposed lava flows both associated with the 6th cycle of Vesuvius activity (Arnò et al., 1987). Among these sites, only the so-called “Novelle-Castelluccio” quarry (Penta, 1935) still shows a clearly evident front wall.
As remarked in the “Geological outline” section, Phlegrean lavas are very rare and limited to small-scale manifestations. They are basically restricted to the young eruptions of Astroni (Di Vito et al., 1999a; Isaia et al., 2004), Monte Spina (de Vita et al., 1999) and Monte Olibano (Di Girolamo et al., 1984; Rosi and Sbrana, 1987; Isaia et al., 2009), and to the pre-CI domes of Cuma, Punta Marmolite and S. Martino (Di Girolamo et al., 1984; Rosi and Sbrana, 1987; Pappalardo et al., 1999; Fedele et al., in press; Fig. 49), in both cases of mainly trachytic composition. Monte Olibano lava flow, definitely representing the most important deposit, was the object of a significant exploitation activity, made even easier via sea transportation facilities (Cardone and Papa 1993). Within this deposit, three different types of lava were identified by Sinno (1955): a basal one, apparently homogeneous, ash grey in colour, where feldspar phenocrysts are hardly distinguishable due to the colour of the matrix; a “powdery” intermediate one, with clearly visible feldspar phenocrysts; an upper one with feldspar phenocrysts evident in a dark grey matrix. As far as petrophysical features are concerned, Maggiore (1936) distinguishes two different typologies of Phlegrean trachytes: a “compact” and a “porous” one. These two different types can even occur within the same flow unit, gradually passing from one variety to another from the bottom to the top of the formation.
Figure 49. Phlegrean lava quarries.
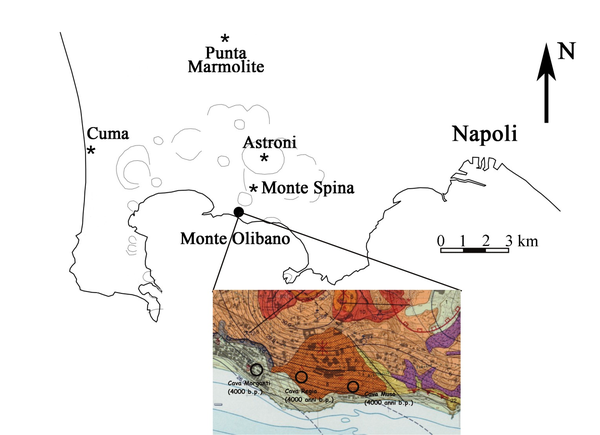
Location of extraction sites of the Phlegraean lavas [Monte Olibano; extract from Geological Map 1:15,000 from Rosi and Sbrana (1987)].
The main exploitation areas of the so-called “Phlegrean trachyte” are represented by some well-preserved old quarries sited close to the west side of the town of Pozzuoli: “Cava Regia”, “Cava Muso” and “Cava Morganti”, on the Monte Olibano lava dome. Cava Regia is undoubtedly the most important both in terms of thickness and in the amount of exploited material. This is also the only accessible quarry, as the others are located in a military area protecting the Aeronautic Academy. The front wall of the Cava Regia quarry (Fig. 50) is about 70 m high and two different layers can be identified from the bottom to the top: a) an ash grey compact “trachyte” with lighter area (thickness = 55-60 m); b) a light grey less compact and powdery trachyte, deeply fumarolized (thickness = 10-15 m).
Mineralogical composition of the Vesuvian and Phlegrean lavas
All the Vesuvian samples have a porphyritic texture, with clinopyroxene representing the only phenocryst, sometimes along with leucite. Microphenocrysts are represented by leucite, clinopyroxene and biotite. The results of optical microscopy have been confirmed by the XRD analyses, with the identification of the the following minerals: clinopyroxene (42.0-26.6%), Na-plagioclase (33.9-25.1%), leucite (22.6-17.3%), sodalite (4.3-3.4%), sanidine (5.5-3.7%,), biotite (3.3-1.9%), horneblende (2.6-0.2%) and magnetite (0.8-0.1%).
Phlegrean lavas, on the other hand, show a porphyritic texture with phenocrysts of sanidine and, subordinately, clinopyroxene. Microphenocrysts are mostly magnetite and strongly zoned Na-plagioclase. Groundmass is mainly constituted by feldspar, diopsidic pyroxene, brown biotite, magnetite and very-rare plagioclase. XRD quantitative analyses gave the following results in order of abundance: sanidine (80%), Na-plagioclase (6.9-4.5%), diopside (5.7-5.4%), and biotite, magnetite and horneblende in very low amount (1%).
Petrophysical features of the Vesuvian and Phlegrean lavas
Table 9 shows the main physico-mechanical parameters of Vesuvian and Phlegrean lavas. In the former, the low values of open porosity clearly determined a reduced difference between bulk and apparent density, with consequent low values of total water absorption (Fig. 51) and capillary absorption coefficients (Fig. 52). A substantial homogeneity was recorded for the water absorption curves after total immersion in samples from different outcrops. The same remarks apply to capillary water absorption curves which also show an overall homogeneity with only one exception (sample VI) characterized by a higher value, along with the highest total apparent porosity value. A unimodal mesocurtic pore size distribution was also observed with highest concentration of pores in the 0.3-1.2 μm size range (Fig. 53).
Table 9. Main physical properties of Vesuvian and Phlegrean lavas (Langella et al., 2009).
Dry density | Specific gravity | Open porosity | Imbibition coefficient | Compressive strength (UCS) | Young’s elastic modulus | |
---|---|---|---|---|---|---|
(kN/m3) | (kN/m3) | (%) | (%) | (Mpa) | (GPa) | |
Vesuvian lavas | 25.70-27.10 | 28.30-29.00 | 6.46-9.25 | 1.41-1.63 | 165-181 | 26.92-49.98 |
Phlegraean lavas | 21.30-25.00 | 26.60-27.20 | 7.67-21.11 | 1.43-7.31 | 38-208 | 12.58-56.80 |
Figure 51. Technical features of Vesuvian and Phlegrean lavas.
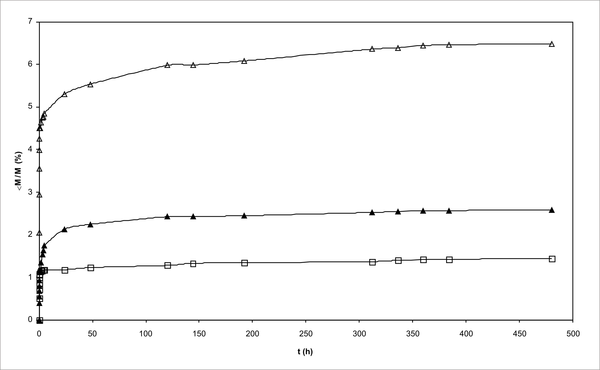
Water absorption curves by total immersion as a function of time [empty squares = Vesuvian lavas; solid triangles = Phlegraean lavas (lower level); empty triangles = Phlegraean lavas (upper level)] [after Langella et al. (2009), modified].
Figure 52. Technical features of Vesuvian and Phlegrean lavas.
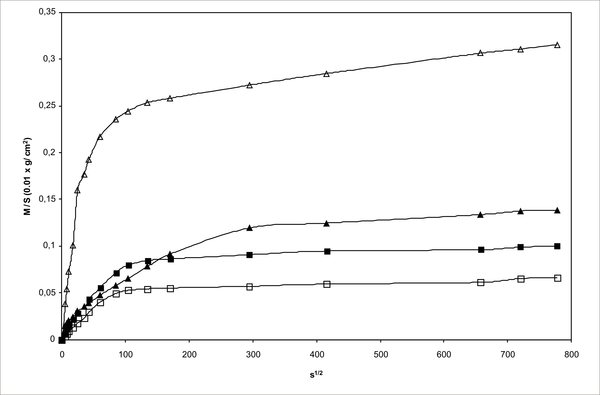
Capillary water absorption curves as a function of time [squares = Vesuvian lavas, black square = VI sample; solid triangles = Phlegraean lavas from Monte Olibano, lower level; empty triangles = Phlegraean lavas from Monte Olibano, upper level; after Langella et al. (2009), modified].
Figure 53. Technical features of Vesuvian lavas.
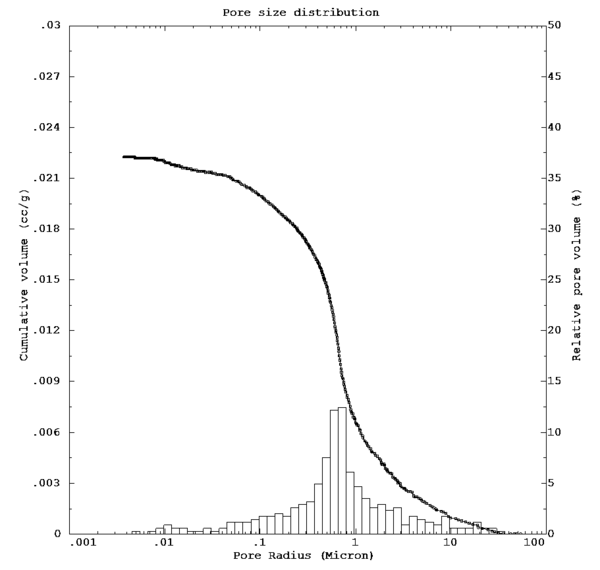
Pore size distribution in Vesuvian lava sample from Terzigno Tz-1 [after Langella et al. (2009), modified].
In the case of the Phlegrean lavas, petrophysical tests were carried out on samples collected from the two layers of the investigated outcrop at Monte Olibano. Lava samples collected from the lower layer (OL1, OL4 and OL5) show a negligible difference between bulk and apparent density and much wider from those belonging to the upper one (OL2 and OL3). This difference is confirmed by the values of total open porosity, close to 10% for the former set of samples and close to 20% for the latter. Pore size distribution also allows us to distinguish two different typologies of material: the first (typical of lava samples OL1, OL4 and OL5) shows low values of open porosity and a unimodal platicurtic pore size distribution; the second one (typical of all the other lava samples) shows a bimodal distribution (Fig. 54). Such variability also occurs in terms of water absorption by total immersion (Fig. 51) and by capillary action (Fig. 52), with the highest values perfectly corresponding to those of porosity.
Figure 54. Technical features of Phlegrean lavas.
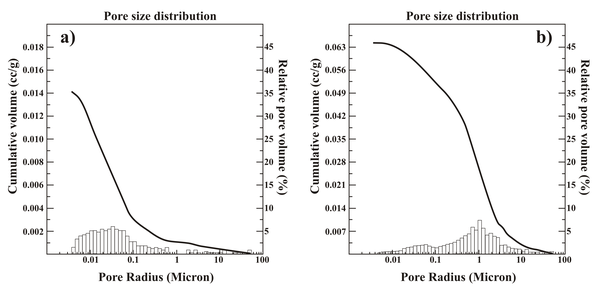
Pore size distribution in Phlegraean lava samples from Monte Olibano OL 1 (a) and OL 2 (b) [after Langella et al. (2009), modified].
For uniaxial compressive strength values, a substantial homogeneity of mean values was recorded for Vesuvian samples, ranging between 165 MPa and 181 MPa. These data are very homogeneous and show a very good fit with those of total open porosity, always lower than 10%. Phlegrean lavas, on the contrary, show quite variable mean values of UCS. Two different classes are therefore distinguished: one with values ranging between 138 MPa (OL4 and OL5) and 208 MPa (OL1), and a second one with definitely lower values (38 and 63 MPa, OL3 and OL2 samples, respectively). It should be said that lava samples characterized by porosity values close to or lower than 10% belong to the first class whereas those with higher porosity values (up to 20%) to the second one.
Young’s modulus values confirm the previous considerations even though a higher variability was recorded for Vesuvian lavas (26.92-49.98 GPa). The already reported two-class division for Phlegrean lava is also verified - with a marked homogeneity of the values being noted within each class. Following the classification of Deere and Miller (1966), Vesuvian lavas are defined as strong to very strong and on an average stiff to stiff materials, while Phlegrean ones are considered as strong to very strong and stiff (Fig. 55).
Figure 55. Technical features of Vesuvian and Phlegrean lavas.
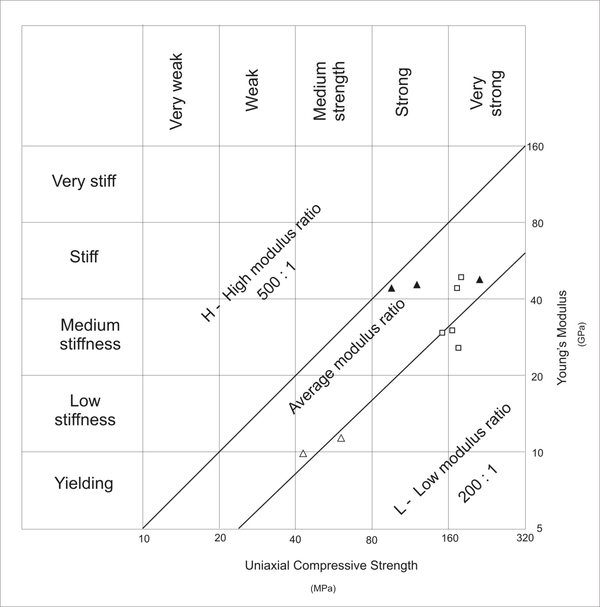
Engineering classification of the investigated lavas according to Deere and Miller (1966) [squares = Vesuvian lavas, black square = VI sample; solid triangles = Phlegrean lavas from Monte Olibano, lower level; empty triangles = Phlegraean lavas from Monte Olibano, upper level; after Langella et al. (2009), modified].
The Vesuvian and Phlegrean lavas as building materials
Lava has been used as a building stone in the Campanian architecture intermittently, mainly after the second half of the 17th century and, particularly, between the 18th and 19th centuries. Examples of uses of these rocks since Greek–Roman ages are evident nevertheless, principally for paving roads. Notable examples include the roads within the Cuma acropolis (Fig. 56), those present in the archaeological area of the Arco Felice made of Phlegrean trachyte or even those within old Pompei made of Vesuvian lava. Even though the historical use of this stone is definitely subordinate when compared to other more easily workable materials (such as the yellow tuffs (LYT) of the CI) uses of Vesuvian lava in Pompei to perform architectural details such as opus reticolatum (Odeon/Theatre) and columns, or of Phlegrean lava in Pozzuoli (piers of brick arches within the Flavio Amphitheatre) are not lacking (Cardone and Papa, 1993). In the 14th century (1317) a large amount of Phlegrean trachyte was used to pave many roads of Naples town by arrangement of Roberto d’Angiò (Rodolico, 1953).
The period between the end of 13th and 14th centuries signifies an important step in the use of the Phlegrean lava in Neapolitan architecture, as seen in the S. Lorenzo Maggiore (1270-1275; basement and some columns), San Domenico Maggiore (1283-1324; piers of the arches), Santa Chiara (1310-1328; columns of the arcade; Fig. 57), S. Maria Donnaregina (1307-1316; pillars of the choir) and S. Giovanni a Carbonara (1343-1418; big triumph Arch) churches. Some architectural elements of the Maschio Angioino Castle (1281-1284; base of the triumph arch and frames in the Barons’ Hall) were also made with lava from Campi Flegrei whose use continued also in the following centuries as confirmed by the 16th century building at Spirito Santo (1539) currently hosting the Banco di Napoli, and the central colonnade of the S. Francesco di Paola Church (1817; Penta, 1935; Cardone and Papa, 1993; Fig. 58). From the end of the 17th century through the end of the 18th century, the increasing use of Piperno (which displayed better technical features), instead of Phlegrean lava is recorded (Cardone and Papa, 1993; Aveta, 1987). Vesuvian lava, also known as “Pietrarsa” (i.e., burned stone), only from the 19th century became a fundamental stone in the religious and civil architecture of the town of Naples. This aspect is related to the fact that, only after the 1631 A.D. and subsequent Vesuvius eruptions, a certain amount of material qualitatively and quantitatively suitable for that purpose was available (Penta, 1935; Rodolico, 1953). The main use of lava stone was in slabs for basal coatings [basal facings, such as in S. Giorgio Maggiore Basilica, rebuilt in 1631 and restored in the second half of 19th century (Capuano Castle, twelfth century, 1857); the Federico II University, 1908], or to perform architectural elements such as portals, corner stones, frames, sills, brackets, stairs, etc. (Penta, 1935; Fiengo and Guerriero, 1999). The use of lava for internal coatings or decorative elements was demonstrated by Vanvitelli’s work in the Caserta Royal Palace (1752) (Patturelli, 1826). Also worth noting is the combined use of Vesuvian lava (Pietrarsa) and Piperno in the Schilizzi Mausoleum (now the war memorial Votive Altar; Fig. 59) at Posillipo (Penta, 1935) and the huge use in the funeral architecture of the historical part of the Poggioreale Cemetery (Penta, 1935).
Figure 59. The Vesuvian lavas as building stones.
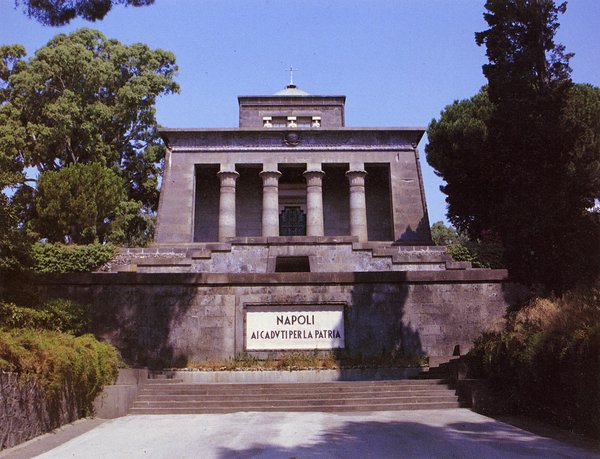
Vesuvian lavas used in the Schilizzi Mausoleum, currently war memorial Votive Altar (1883-1923)
Weathering processes affecting the Vesuvian and Phlegrean lavas
A detailed analysis of the conservation state of the Vesuvian lavas showed that more than 50% of the exposed surfaces are affected by a high-grade decay process, about 20% by a medium-grade and about 23% by a negligible grade (Calcaterra et al., 2000a, b). Previous data have been supplemented with a more complete weathering evaluation, taking particular account of the decay forms and their percentage frequency. Figure 60 reports the weathering forms and the relative per cent frequency for the Vesuvian lavas in the Ancient Centre of Naples.
The most diffused weathering forms are patina and artificial patina (mainly writings and graffiti). More than 20% of the surfaces are affected by exfoliation processes which are manifested by a detachment, often followed by the fall of one or more sub-parallel surface layers (Fig. 61). Incrustations, scaling (Fig. 62), lacks, alveolization and erosion are widespread but with a frequency always lower than 10%. All the remaining weathering forms never exceed 3%. Optical microscopic studies of samples collected from some buildings (Policlinico building on via del Sole) detected a continuous 0.2 mm thick black crust (unresolvable, however, with this technique). Later XRPD investigation identified gypsum as the main mineralogical constituent of these crusts.
Figure 60. Weathering of the Vesuvian lavas.
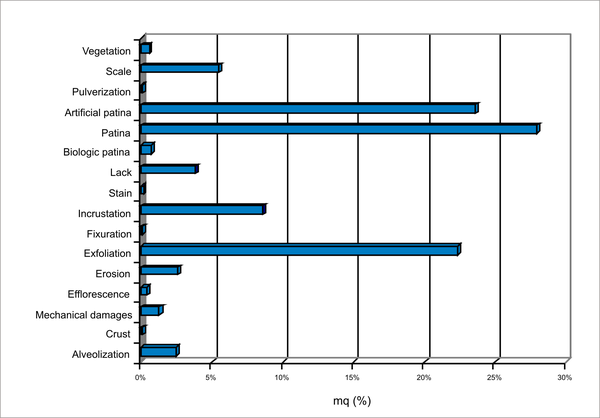
Weathering typologies affecting the Vesuvian lavas of the Ancient Centre of Naples [after Langella et al. (2009), modified].
Phlegrean lavas are definitely subordinate (about 2% of the total) and are mainly concentrated (about 70%, 275 m2) in the colonnade of the Clarisse Cloister (Santa Chiara Basilica). A comparison with the Vesuvian lavas indicates a slightly worse state of conservation of Phlegrean lava. In fact, ignoring the stone used in the colonnade of the Clarisse Cloister (which is well preserved due to several restorations), 99% of the remaining surfaces are affected by high-grade weathering processes.
Figure 61. Weathering of the Vesuvian lavas.
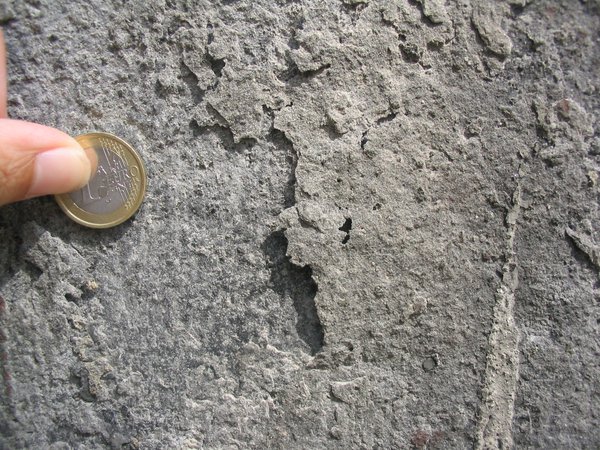
Example of weathering forms affecting Vesuvian lavas: exfoliation, Via del Sole (Naples).
Figure 62. Weathering of the Vesuvian lavas.
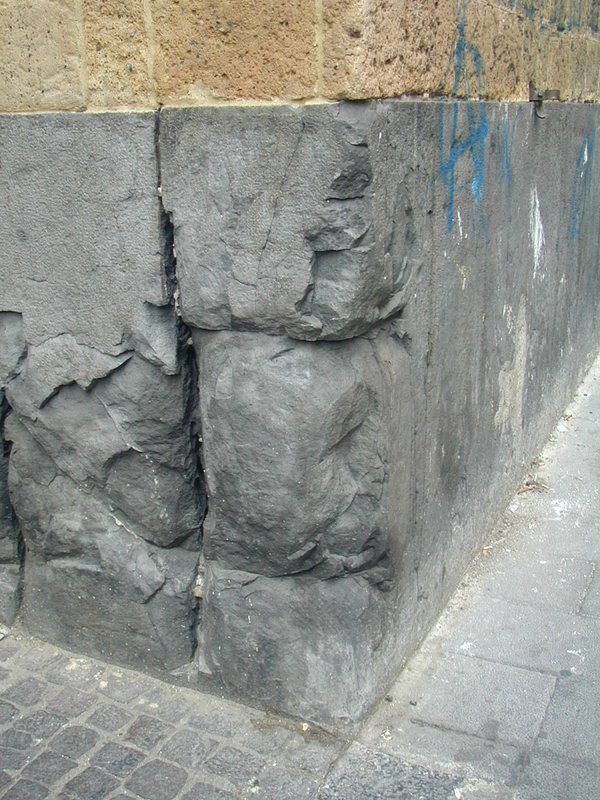
Example of weathering forms affecting Vesuvian lavas: scaling, on basal facing of the Accademia di Belle Arti (Academy of Fine Arts).
Figure 63. Weathering of the Phlegrean lavas.
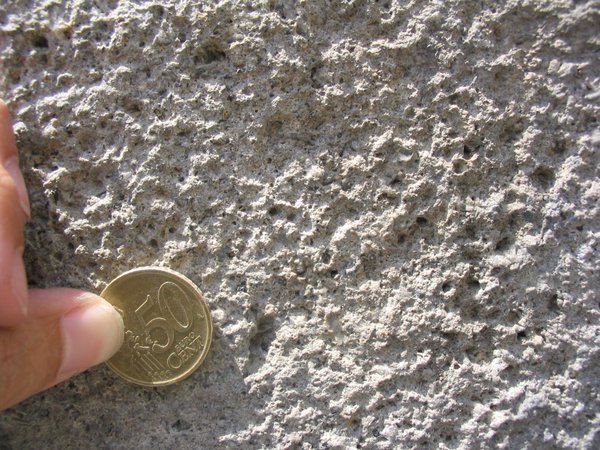
Example of weathering forms affecting the Phlegrean lavas: alveolization, Clarisse cloister, S. Chiara Basilica.
The most diffused weathering typology is undoubtedly the exfoliation, followed by scaling and alveolization (Fig. 63); these lavas are characterized by a more evident occurrence of efflorescences (Fig. 64). The other typologies do not affect more than 10% of the exposed surface (Fig. 65). Again, optical microscope studies (S. Maria La Nova Church) reveal a dark patina about 0.1 mm thick, likely gypsum, and frequent fissures normal to the surface.
Figure 64. Weathering of the Phlegrean lavas.
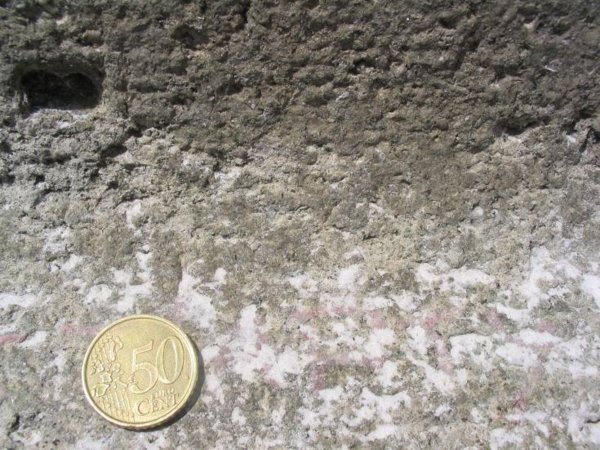
Example of weathering forms affecting the Phlegrean lavas: efflorescence, S. Chiara Basilica.
Figure 65. Weathering of the Phlegrean lavas.
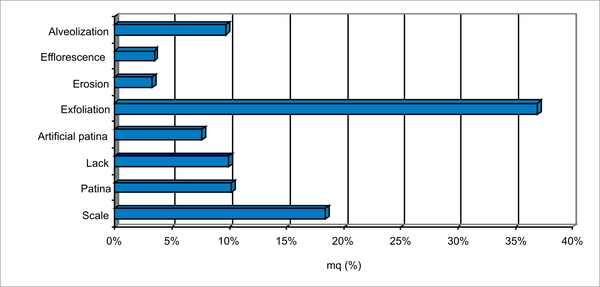
Weathering typologies affecting the Phlegrean lavas of the Ancient Centre of Naples [after Langella et al. (2009), modified].
The Phlegrean “Pozzolana”
A detailed discourse on the uses of geomaterials in the Neapolitan area cannot ignore some aspects concerning the extensive use of the so-called “Pozzolana”. This natural product of Campi Flegrei activity, widespread in the Campanian region, was a fundamental component of mortars and plasters since Roman times. The term "pozzolana" refers to a volcanic product, prevailingly glassy with small pumices, lithic fragments and K-feldspar crystals set in an ashy matrix. The glassy component sometimes exceeds 90%. The same term is generally used for all loose products of the Phlegrean volcanism with similar compositional features, even though the most well known and used for its particular features is the pozzolana ascribed to the activity of Fondi di Baia and Bacoli volcanoes.
The prevailing glassy fraction of pozzolana makes it particularly reactive when mixed with lime [Ca(OH)2] and water. The products of this reaction are silicates and calcium aluminates which determine the setting and the hardening of the mortar. Therefore, unlike air-setting limes, these mortars can set underwater. The so-manufactured mortars are definitely better than those made with other inert aggregates. This specific property, as previously stated, was already known in the Roman age; in fact, Marco Pollio Vitruvio in his “De Architectura” distinguishes the volcanic sands from Baia and Cuma suitable for providing hydraulic mortars from other volcanic products that can only be used to produce air-setting mortars. The discovery of hydraulic limes certainly started the production of the commonly defined roman concrete (opus caementicium) - that is, a slurry composed by a mixture of lime, pozzolana, brick fragments and water. This technique enabled Romans to carry out great architectural works such as bridges on Tevere river, many aqueducts and harbours (Ostia, Pozzuoli, Civitavecchia, Anzio). Also, these mortars turned out to be particularly suitable in the Neapolitan area for walls made up of tuff bricks which showed a good adherence to the mortar. On the other hand, this was likely the reason why Vitruvio suggested the use of lime- and pozzolana-based concretes mixed with tuff shards for objects that would not interact with water. This aspect led to a growing demand of pozzolana which was exploited almost everywhere, but mostly in the deposits of Baia and Fondi di Baia, and from there subsequently redirected to the nearby dock. This activity lasted up to the second half of the last century. During this time, several quarries were located in the Phlegrean area, and most of them were active until they were exhausted (Sersale, 1991; Cardone and Papa, 1993; Collepardi, 2008).