The redox state of Ulten Zone peridotites and inference on fluid phase speciation
Crystallization of amphibole and dolomite in the HP Ulten garnet peridotites due to influx of aqueous-carbonic fluids makes these rocks a relevant natural laboratory to understand C and volatile recycling in the mantle via subduction. This represents the "missing link" of global volatile cycles due to inaccessibility of subduction environments. Also, unravelling "how" C-O-H compounds occur in the mantle, i.e. their speciation, is necessary to predict the evolution of carbon species during long-term geochemical cycles. Natural mineral assemblages and laboratory experiments demonstrate that the composition of C-O-H fluids in the mantle closely relates to the oxidation state of the system (Van Roermund et al., 2002; Carswell and Van Roermund, 2005; Scambelluri et al., 2008; Tumiati et al., 2009; Malaspina et al., 2009b; Malaspina et al., 2010). The latter issue is relevant for C recycling, as either diamond/graphite generally crystallize depending on the chemical potential of oxygen and therefore on the oxygen fugacity. Graphite and diamond generally behave as refractory phases, whereas carbonates, although refractory, are highly reactive (Canil, 1990). An estimate of oxygen fugacity (ƒO2) attained by the Ulten Zone garnet (+ amphibole + dolomite)-bearing peridotites is thus essential to model C transfer during subduction.
The equilibria between ferric (Fe3+) and ferrous (Fe2+) iron in mineral assemblages buffer the oxygen fugacity and the C-O-H fluid speciation. On the other hand, the C-O-H species in fluids or melts can control the oxidation state of mantle phase assemblages by redox processes (Luth, 1999). Being the Fe3+/Fe2+ equilibria of mantle parageneses and the speciation of C-O-H fluids so related, Malaspina et al. (2009b) determined the iron oxidation state of garnet in the Ulten Zone peridotites. Several redox equilibria involve Fe3+-garnet components; for an olivine-orthopyroxene-Fe3+-garnet assemblage, one of these reactions is:
(1) 2 Fe2+3Fe3+2Si3O12 (skiagite) = 4 Fe2+2SiO4 (fayalite) + 2 Fe2+2Si2O6 (ferrosilite) + O2 where the ferric garnet component "skiagite" contains both Fe3+ and Fe2+ (Luth et al., 1990; Gudmundsson and Wood, 1995; Woodland and Peltonen, 1999). The electron microprobe analysis of the Fe3+ content of garnet from the Ulten peridotite (equivalent to skiagite substitution for almandine) adopting the "flank method" (Höfer and Brey, 2007; see details in Malaspina et al., 2009b) provided skiagite contents in the order of 3 (±1) mol%. The corresponding ƒO2 has been calculated by Malaspina et al. (2009b) using the Perple_X software (Connolly, 1990) applying the redox equilibrium (1) and integrating solid solution models for garnet (pyrope-grossular-almandine-skiagite; Malaspina et al., 2009b), olivine (forsterite-fayalite; Holland and Powell, 1998) and orthopyroxene (enstatite-ferrosilite; Holland and Powell, 1996). The resulting redox equilibria are shown in the isobaric T-log ƒO2 section computed at 3 GPa pressure (Fig. 14): the dashed curves represent the equilibria selected for pseudocompounds approaching the investigated mineral compositions (olivine = Fo89; orthopyroxene = En89; garnet = 70% pyrope, 10% grossular and variable skiagite/almandine depending on oxygen fugacity). The equilibria involving hematite, magnetite, fayalite, quartz, ferrosilite, wustite and iron at various buffering conditions are also shown in Figure 14 (continuous lines) for comparison. The estimated oxygen fugacities range from -10.69 to -8.94 log units (Fig. 14); the resulting Δlog ƒO2 [=log ƒO2 (sample) - FMQ] range between zero and +2 (Fig.15).
Figure 14. Log ƒO2 and speciation of a graphite–C–O–H fluid.
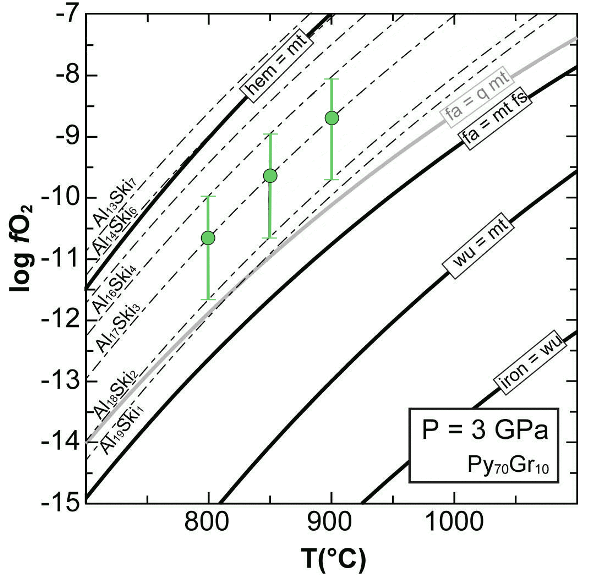
Log ƒO2 (a) and speciation of a graphite–C–O–H fluid (b) calculated at 3 GPa and 850 °C as a function of X(O) = nO/(nO+nH). The green line (a) and dashed green area (b) is the ƒO2 range calculated for the Ulten peridotites. Calculation have been performed with the programme "species" in Perplex package (Connolly, 1995). FMQ = fayalite–magnetite–quartz buffer, WM = wustite–magnetite buffer, IW = iron–wustite buffer.
In Figure 15, the estimated oxygen fugacities of the Ulten Zone garnet peridotites (expressed as Δlog ƒO2 values) are plotted as a function of P, together with other mantle peridotites from distinct geological environments. Measured ƒO2 values vary from: (i) 1 to 3 log units below the FMQ in abyssal peridotites (Bryndzia & Wood, 1990); (ii) Δlog ƒO2 = +1 to –2 for peridotite massifs, with samples from Beni Bousera reaching up to Δlog ƒO2= –4 (Woodland et al., 1992; Woodland et al., 2006); (iii) Δlog ƒO2= –1 to +2 for spinel peridotites from subcontinental lithospheric mantle, with significant heterogeneities (Wood et al., 1990; Canil et al., 1990; Brandon and Draper, 1996; Parkinson and Arculus, 1999). The garnet peridotites from sub-cratonic mantle have the lowest ƒO2 values, below Δlog ƒO2= –2 (Woodland and Koch, 2003). Differently from these mantle rocks, the Ulten peridotites display high Δlog ƒO2 values (0 to +2). These values are comparable with those estimated for spinel peridotite xenoliths from subduction settings (Simcoe, Vanatu and Japan; Δlog ƒO2 between –1 and +1.5; Brandon & Draper, 1996; Parkinson et al., 2003). Importantly similarly high values of ƒO2 are shown by other UHP orogenic garnet peridotites, like the ones from Sulu (China) (Malaspina et al., 2009b; Malaspina et al., 2010). These data point to a lateral oxygen fugacity variation related to different tectonic settings (e.g. Woodland and Koch, 2003; Frost and McCammon, 2008).
As previously mentioned, the ƒO2 recorded by the Ulten peridotites have implications on the speciation of the coexisting C-O-H fluid phase. According to Holloway and Reese (1974) and to the model of Connolly (1995), C-saturated fluids can be either methane-bearing or carbon-dioxide bearing as a function of the oxygen fugacity imposed by the redox conditions of the system. At high pressures H2O is the dominant species in the C–O–H fluid, which will be a mixture of CH4–H2O at lower ƒO2 and of H2O–CO2 at higher ƒO2. Despite the absence of diamond and graphite in the studied peridotites, a C-saturated system can be used as a proxy to estimate the fluid composition. This is possible because at the high pressure and temperature conditions attained by the Ulten Zone garnet-peridotites, the graphite saturation boundary approaches the binary joins H2O–CO2 and H2O–CH4 (Holloway & Reese, 1974). Based on this reasoning and on calculations performed using the GC-O-H-MRK equation of state by Connolly and Cesare (1993) for a C-saturated C-O-H fluid, Malaspina et al., (2009) have evaluated the C–O–H molecular species concentrations of the metasomatic fluid. This points to high water content of the fluid and the presence of CO2 (instead of CH4) at Δlog ƒO2 > 0. This confirms the expectations of what discussed by Rampone and Morten (2001) and Sapienza et al. (2009) on the basis of petrographic evidence and trace element budgets.
Figure 15. ΔFMQ versus pressure.
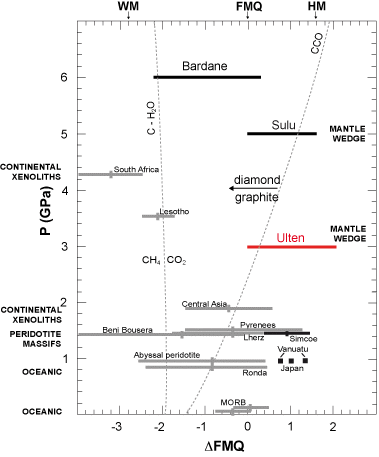
Range and average value of oxygen fugacities relative to FMQ (ΔlogƒO2 = logƒO2sample - logƒO2FMQ) for the Ulten garnet peridotites (red line) plotted as a function of pressure (modified after Malaspina et al., 2009b). This is compared with selected examples of spinel and garnet peridotites from different tectonic settings, equilibrated at similar T conditions (~800-900 °C): oceanic mantle lithosphere (Bryndzia & Wood, 1990), peridotite massifs (Woodland et al., 1992; Woodland et al., 2006), garnet peridotite xenoliths from sub-cratonic mantle (Woodland and Koch, 2003) and mantle wedge from spinel facies (Brandon and Draper, 1996; Parkinson and Arculus, 1999; Peslier et al., 2002). Mantle wedge-derived orogenic garnet peridotites from Sulu (Eastern China) and Bardane (Western Gneiss Region) are also reported in black lines (Malaspina et al., 2009b, 2010). The CCO oxygen buffer (C+O2 = CO2) and the C-H2O join (X(O) = 1/3) separating CH4- and CO2-rich aqueous fluid, calculated at 900 °C, are also portrayed in dashed lines.