The geochemical fingerprint of the Ulten Zone garnet-bearing peridotites: record of crustal metasomatism by subduction fluid
The major and trace element composition of the Ulten Zone peridotites has been the subject of several papers that contributed to build a large and complete dataset (Obata and Morten, 1987; Morten and Obata, 1990; Rampone and Morten, 2001; Scambelluri et al., 2006; Tumiati et al., 2007; Marocchi et al., 2007, 2010; Sapienza et al., 2009). We refer to the above contributions for details and tables with analytical results. In terms of most major and trace elements (Si, Mg, Ca, Al, Sc, Co, V, Ni), no systematic correlation exists between bulk peridotite compositions, textural types (coarse vs. fine) and metamorphic assemblage (spinel vs. garnet + amphibole; Morten and Obata, 1990; Bondi et al., 1992). Variations in element concentrations most likely reflect primary heterogeneities of the mantle protoliths. The fine-grained (garnet + amphibole) types are significantly enriched in alkalis (especially K2O) and Large Ion Litophile Elements (LILE): this enrichment is unrelated to major element chemistry and is roughly correlated with the Sr isotopic compositions of the peridotites (Bondi et al., 1992; Petrini and Morten, 1993; Rampone and Morten, 2001).
Hereafter we present separately the main bulk-rock and mineral chemistry features of the coarse spinel- and of fine-grained (garnet + amphibole)- bearing peridotites.
Bulk rock compositions.
The spidergrams in Figure 10 report the REE and the other trace element compositions of spinel (Fig. 10A, B) and (garnet + amphibole) peridotites (Fig. 10C, D) normalized to the Primitive Mantle (Mc Donough and Sun, 1995). All samples display decreasing heavy REE (HREE) with decreasing atomic number, a common feature of depleted peridotites that is in agreement with the variability of Al2O3, CaO, MgO and indicates that the starting peridotites recorded variable degrees of depletion (Rampone and Morten 2001). For this reason they are compared in Figure 10 to a depleted mantle source (DMM, Workman and Hart, 2005).
Figure 10. Bulk-rock REE and trace element samples.
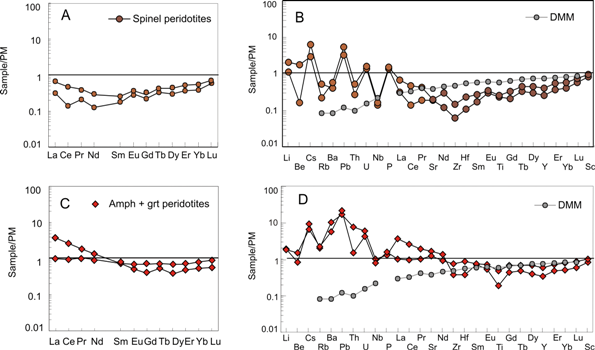
Bulk-rock REE (left side) and trace element (right side) compositions of spinel peridotites (A and B) and of (garnet + amphibole ± dolomite) peridotite samples (C and D). Normalization to Primitive Mantle by McDonough and Sun (1995)
The spinel peridotites of Figure 10A, B did not recrystallize to garnet-facies assemblages (i.e. they do not contain appreciable modal garnet and amphibole): they have low REE abundances, below 1 x PM and U-shaped REE patterns variably enriched in LREE. Comparable features pertain to samples with coronitic garnet, scarcely affected by garnet + amphibole crystallization (see Rampone and Morten, 2001).
The (garnet + amphibole) peridotites (Fig. 10C, D) display REE abundances around the PM: they have flat HREE to MREE spectra and LREE enrichments up to 10 x PM. As outlined by Rampone and Morten (2001), this variability is unrelated with the degree of major element depletion. Figure 10 shows that the amphibole-free spinel peridotites and the garnet + amphibole peridotites are all affected, although in different extent, by metasomatic LREE enrichment.
The trace element spidergrams (right hand side of Fig. 10) show that all samples, spinel-peridotites included, display positive anomalies in LILE, particularly Cs, Pb and U. This occurs irrespective of the depletion in Al2O3 and CaO and the degree of enrichment is significant if compared with the DMM (e.g. Rb, Ba, Th, P in spinel peridotites). The (garnet + amphibole) peridotites display higher Rb, Ba, Th than the spinel peridotites. Lithium is appreciably enriched (about 2 x PM) in the majority of the peridotite samples analysed (Scambelluri et al., 2006). The selected samples are affected by minor serpentinization and by formation of small modal amounts of retrograde tremolitic amphibole; hence some incompatible element enrichment (e.g. Li, Cs, Ba, Rb) can be in principle attributed to retrograde metamorphism. Some of the above mentioned incompatible elements display positive correlations with the modal amphibole contents (estimates from Rampone and Morten 2001). As an example, the bulk-rock Pb and Sr versus modal amphibole diagrams (Fig. 11) illustrate the coupled increase in such elements and water in the amphibole-bearing peridotites. Positive correlation trends are also defined by the incompatible element pairs Pb–Sr and Pb–U (Fig. 8). This indicates that the transition from dry spinel lherzolites to wet (amphibole ± garnet) peridotites was assisted by the influx of an aqueous fluid carrying incompatible trace elements. The early timing of this event (peak eclogitic or retrograde) can be fixed by measuring the trace element compositions of specific mineral assemblages.
Mineral compositions
The average compositions of minerals from the spinel-peridotites are shown in the PM-normalized diagrams of Figure 12. Clino- and ortho-pyroxene from these rocks have convex-upwards REE patterns, depleted in MREE and variably enriched in LREE. Both ortho- and clinopyroxene enriched in LILE with respect to high field strength elements (HFSE), with normalized Pb/Nb ratio in clinopyroxene (representing the LILE/HFSE fractionation) ranging from 16 to 90. The LREE- and LILE-enriched trace element signature of spinel facies pyroxenes indicates that the spinel peridotites experienced a criptic metasomatism by chromatographic porous flow (Bodinier et al., 1990) that likely predated subduction zone metasomatism and fluid infiltration during garnet-facies recrystallization and coupling with the crust (Scambelluri et al., 2006). This metasomatism has been ascribed by Scambelluri et al. (2006) to high-temperature interaction with subduction-related siliceous melts when these mantle rocks were at relatively shallow (spinel-facies) lithospheric depths.
Figure 12. Rare earth element and trace element compositions
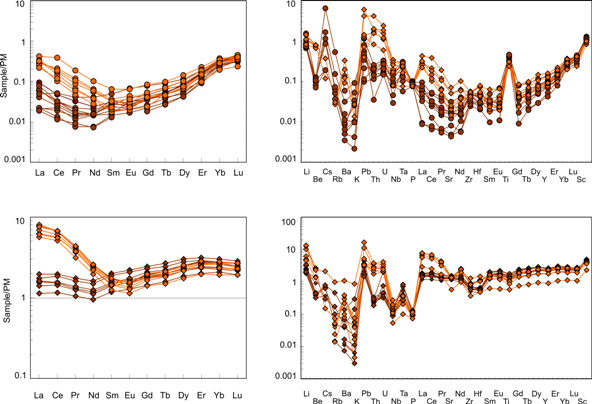
Rare earth element and trace element compositions of orthopyroxene (A and B) and of clinopyroxene (C and D) from two spinel peridotite samples, reported with two different colours (brown and orange) in the figure. Normalization as in Figure 5.
The compositions of the main rock-forming minerals in the garnet peridotites are shown in Fig. 13. These rocks contain garnet, clinopyroxene, orthopyroxene, olivine, amphibole (± phlogopite, carbonate and apatite) in textural equilibrium and represent highly deformed, hydrated and locally carbonated mantle rocks. The LREE content of the garnet-facies clinopyroxene is up to 10 x PM (Fig. 13); the coexisting orthopyroxene is slightly enriched in LREE. Compared with the spinel-facies pyroxenes, they display much lower HREE, between 0.1 and 0.01 x PM, monitoring full equilibration with garnet at eclogite-facies. Amphibole in textural equilibrium with garnet has LREE-enriched patterns (La up to 10–30 PM) and low HREE contents (0.3–0.5 PM). More generally, the garnet-facies amphibole has flat HREE, about 2 x PM: this suggests partial HREE re-equilibration of amphibole during retrograde garnet breakdown. Clinopyroxene in the (amphibole + garnet) peridotites is enriched in Li, Be, Cs, Ba, Pb, U. It also displays high PbN/NbN (330–615) and low CeN/PbN (1.39–2.66) ratios, compared with the spinel-facies clinopyroxenes. Olivine has Li and P between 3–4.5 ppm and 40–70 ppm, respectively. Dolomite has LREE/HREE >> 1 (LaN/YbN up to 317); the PM-normalized incompatible multi-element diagram shows enrichments in Ba, Pb and Sr over U, Th, REE and HFSE; the latter are often below detection limits or they occur in very low concentrations (e.g. Hf < 0.01 ppm, Nb < 0.085 ppm, Ta < 0.01 ppm).
HREE depleted amphibole coexisting with garnet (see Fig. 13) indicates that peridotite hydration occurred at eclogite-facies conditions. This demonstrates that water-rich fluids entered the anhydrous spinel-facies peridotites during subduction-related high-pressure metamorphism. Relevant features of the (garnet + amphibole) peridotites are the bulk-rock positive anomalies in Cs, Ba, Pb, U and Li (twice the PM; Fig. 13). The LILE and LREE budget is nearly entirely hosted by amphibole and clearly reflects addition of crust-derived components to the mantle rocks. Therefore, the coupled increase of modal amphibole content (see Fig. 11) and bulk Sr, Pb, U was achieved during fluid-mediated eclogitization of the mantle wedge Ulten Zone peridotite.
Amphibole in the Ulten garnet peridotites exhibits high LILE and relatively low HFSE content (Fig. 13). Amphibole and phlogopite are common minerals in metasomatized mantle rocks and provide important information about the nature and composition of metasomatic agents. Amphibole related to alkali-rich silicate melts is generally LILE- and HFSE-enriched and does not display fractionation between these elements (Ionov et al., 1993). Selective enrichment in LILE with respect to HFSE, as shown by the garnet-bearing Ulten Zone peridotites, is documented in mantle xenoliths affected by carbonatite metasomatism and in orogenic peridotites infiltrated by crust-derived metasomatic agents (Zanetti et al., 1999; Malaspina et al., 2006; Scambelluri et al., 2008). In the Finero peridotite, for instance, LILE-enriched and HFSE-depleted amphibole has been linked to mantle wedge metasomatism by Si-rich hydrous melts (Zanetti et al., 1999). Comparable geochemical fingerprints in amphibole from sub-arc peridotite xenoliths have been either ascribed to hydrous fluids (Vidal et al., 1989; Maury et al., 1992), or to hydrous melts (Schiano et al., 1995). Based on the above arguments, on bulk-rock compositions and on the increasing modal amount of amphibole at the expense of pyroxene, the garnet-facies Ulten Zone amphibole has been attributed to infiltration of crust-derived aqueous fluids (Rampone and Morten, 2001; Scambelluri et al., 2006).
The presence of dolomite in some high-pressure Ulten garnet-peridotites is known since the early work of Morten and Obata (1987) and witnesses the presence and local saturation of CO2 in the metasomatic fluid (Rampone and Morten, 2001). Sapienza et al. (2009) have shown that the Ulten Zone carbonated garnet peridotites display stable dolomite + apatite in HP garnet-bearing subduction assemblage, as shown by the texture and by the HREE depleted composition of dolomite (Fig. 13). Similarly to the associated clinopyroxene and amphibole, dolomite is also enriched in MREE to LREE. It thus appears that amphibole and apatite play a major role as trace element repositories, although dolomite is a major host for Sr, Ba, Pb and, subordinately, LREE. As shown by Sapienza et al. (2009) the trace element compositions of the garnet-facies clinopyroxenes and amphiboles from dolomite-free and dolomite-bearing Ulten Zone rocks largely overlap. Since these peridotite varieties constitute the same (or nearby) outcrops and have been closely associated in space and time, they record infiltration of a common metasomatic CO2-H2O fluid with low CO2/H2O. Water consumption from the fluid during amphibole crystallization led to CO2 saturation in the fluid and to dolomite formation (Rampone and Morten 2001). Low CO2/H2O ratio in the metasomatic Ulten Zone fluid is also suggested by the experimental constraints on fluid phase mobility in the mantle. Watson et al. (1990) determined low wetting angles (60°) for CO2-rich fluids at mantle depths, which favour their stagnation in isolated pockets and prevent their mobility along interconnected networks. On the other hand, aqueous fluid may occur as an interconnected mobile phase at P = 3 GPa and T approaching the solidus.
Peridotite reaction with carbonate-rich melts can produce metasomatic effects (Ionov and Harmer 2002) comparable with the ones described for the Ulten dolomite peridotites. However, several evidence are inconsistent with a melt-related origin of the Ulten Zone carbonate-bearing assemblages. Sapienza et al. (2009) have shown that during metamorphism of the Ulten Zone peridotites, carbonate melts are unstable and should crystallize and/or quench, thus producing carbonate pockets and veins as described in mantle xenoliths (e.g. Ducea et al. 2005; Ionov et al. 1996). Reaction of such melts with peridotites also produces abundant clinopyroxene. These features are not observed in the Ulten Zone peridotites, where modal clinopyroxene decreases as the result of progressive addition of water-rich fluids (Morten and Obata, 1987; Rampone and Morten, 2001). Based on these evidence, Sapienza et al. (2009) proposed that the dominant metasomatic phase affecting both the dolomite-free and the dolomite-bearing Ulten peridotites was a water-rich, CO2-bearing fluid.
Figure 13. Rare earth element and trace element compositions
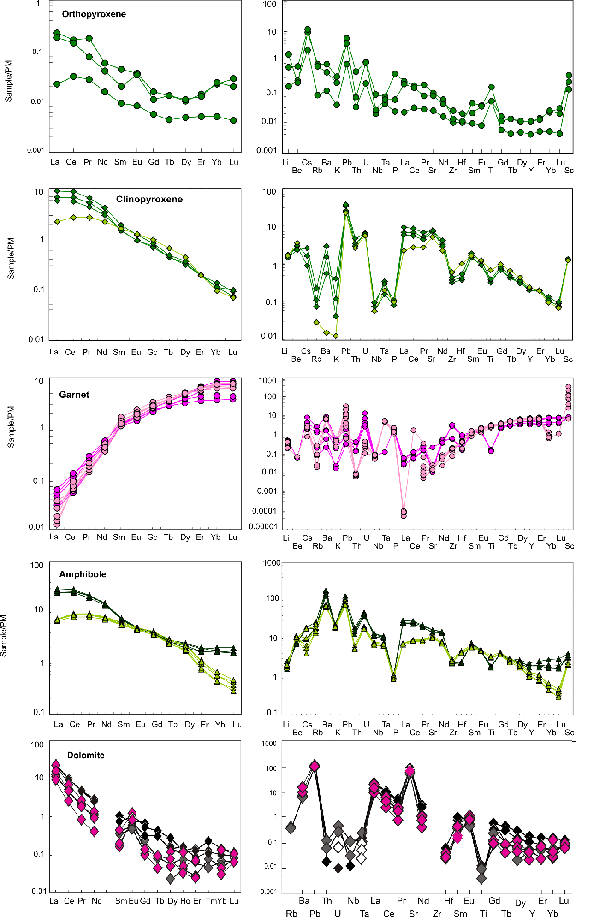
Rare earth element and trace element compositions of minerals of the (amphibole + garnet + dolomite) peridotite. Normalization as in Figure 5.
The pressure–temperature conditions attained during peak eclogite-facies metamorphism by the Ulten Zone dolomite-bearing peridotites are essentially the same as those recorded by the dolomite-free (garnet + amphibole) peridotites (Sapienza et al., 2009). Based on experiments on the peridotite–CO2–H2O system (Wallace and Green, 1988), Sapienza et al. (2009) proposed that crystallization of the Ulten(garnet + dolomite) peridotites occurred at subsolidus conditions (1.8-2.5 GPa and T < 950°C) in the presence of H2O and C-bearing fluid phases. This estimate is consistent with: (1) the 2.5 GPa P estimate based on the Na2O contents of amphiboles in the HP assemblage (up to 2.5 wt% Na2O; Obata and Morten 1987; Niida and Green 1999) and (2) the amphibole stability in peridotite–H2O systems (Fumagalli and Poli, 2005). Carbonatite melts coexisting with (amphibole + garnet) peridotites form above 950 °C in a 2.1 - 3.1 GPa range, whereas C-bearing silicate melts form at higher temperature (Wallace and Green 1988). The Ulten Zone (garnet + amphibole + dolomite) peridotites can be viewed as potential sources of carbonic melts in a subduction setting: their protracted subduction to higher P-T conditions is a possible mechanism leading these rocks to partial melting and release of carbonatite and/or carbon-bearing silicate melts to the mantle wedge (Sapienza et al., 2009). Carbonated and hydrated (phlogopite-bearing) garnet peridotites like the ones from Ulten can represent metasomatized mantle potentially able to generate ultrapotassic magmas like those erupted in the Roman and Lucanian Magmatic Italian Provinces. An increasing number of papers has recently related formation of these magmas to partial melting of lithospehric upper mantle metasomatised by sedimentary-carbonate components derived form the subducted slab. (Conticelli et al. 2002; D'Orazio et al. 2007; Avanzinelli et al. 2008, 2009; Frezzotti et al., 2009)