Major implications and comparison with metasomatized mantle rocks from greater depths
The transition from spinel- to garnet-bearing assemblages in the Ulten peridotites enables to trace the evolution of this mantle from shallow regions towards deeper and cooler levels of the wedge flushed by subduction fluids (Fig. 15). Nimis and Morten (2000) and Scambelluri et al. (2006) proposed location of the starting spinel-peridotites in a relatively hot domain above an asthenospheric melt source. The Pb, U, Li enrichment of the Ulten Zone anhydrous spinel-peridotites can be suggestive of exchange with a (subduction-related?) melt phase enriched in crustal components. Scambelluri et al. (2006) proposed that the metasomatic imprint of the early spinel peridotites might have been acquired by interaction with melts produced during Variscan subduction (Fig. 15). However, this interpretation is hampered by a lack of age constraints for the pristine spinel-facies metasomatism, and it cannot be excluded that it was achieved during older (pre-Variscan?) events that caused the re-enrichment of this lithospheric mantle domain (Petrini and Morten, 1993).
Lateral corner-flow motion brought the spinel-peridotites towards the subducting slab into a P–T regime where fluids are released from slabs (Fig. 16) (Scambelluri and Philippot 2001; Manning 2004). Available studies on the Ulten Zone peridotites agree on the fact that garnet-facies crystallization was driven by aqueous subduction fluids sourced from the crustal rocks nearby (Rampone and Morten, 2001; Tumiati et al., 2003; Scambelluri et al., 2006; Sapienza et al., 2009). Sm–Nd ages of garnet-peridotites, country migmatites and eclogites indicate a common late Variscan (330 Ma) HP event of peridotite metasomatism and partial melting plus eclogitization of crustal rocks (Tumiati et al. 2003). The exact mechanism of peridotite metasomatism is still unclear. According to some authors, crystallization of the garnet-facies assemblage occurred when the spinel-facies rocks were tectonically sliced in the subducting slab (Nimis and Morten 2000; Rampone and Morten, 2001). Alternatively, Scambelluri et al. (2006) proposed that metasomatism occurred before engagement in the crust, when peridotites were still settled in the mantle above the slab (Fig. 16). At eclogite-facies, partial melting of the Ulten continental crust produced the migmatites locally in contact with the peridotite lenses. However, the metasomatic style of the (amphibole + garnet) peridotites indicates that the main agent was an aqueous fluid, not a siliceous melt, and lack of diffuse orthopyroxene overgrowths in these rocks provides compelling evidence that siliceous melt infiltration was not the dominant metasomatic process (Rampone and Morten, 2001). Rather, aqueous fluids have been individuated as dominant agent.
Two mechanisms have been proposed to explain the extraction of C-O-H fluids from the migmatized crustal slab. Rampone and Morten (2001) proposed that the C-O-H fluid responsible for amphibole and dolomite formation in the peridotites was released by the in situ crystallization of hydrous granitic melts produced by the associated crust. Another possible way of C-O-H fluid extraction from these melts requires their interaction with mantle peridotites, to produce pyroxenite reaction layers plus residual aqueous-carbonic fluid (Fig. 16; Scambelluri et al. 2006). Although pyroxenites are present in the Ulten Zone peridotites (Braga et al. 2007), reactive pyroxenite layers and siliceous melt/peridotite reaction zones with (ortho)pyroxene formation after olivine are not yet documented. Therefore there is no direct evidence of an orthopyroxene-rich reactive layer between crustal and mantle Ulten Zone rocks. Interaction between peridotite and siliceous subduction agents has been documented by studies on UHP rocks from Dabie-Shan (China; Malaspina et al., 2006). In this setting, reaction between mantle peridotites and siliceous fluids/melts formed garnet-orthopyroxenite layers and released incompatible element-rich aqueous fluids able to migrate and metasomatize the overlying mantle wedge. The reactive behaviour at the subducted crust–mantle interface may thus filter the slab fluids to produce aqueous fluids enriched in the most incompatible and volatile components (C included) that ultimately flush the mantle. Crystallization of hydrous phases as amphibole or phlogopite may shift the fluid toward CO2-rich compositions and enable carbonate crystallization in inner domains of the mantle wedge. In this second scenario, the reactive orthopyroxene horizon potentially occurring at the Ulten Zone has been tectonically deleted; in this case tectonic incorporation of peridotites into the country rock gneisses might have occurred after the major stage of metasomatism.
The Ulten orogenic mantle wedge peridotites are thus examples of fluid-mediated chemical exchange between subducted plates and overlying mantle. Comparison with other ultra-deep mantle wedge materials may help to clarify the extent of crust-mantle exchange during subduction. In the following we compare the Ulten rocks with metasomatized UHP garnet websterites in the locality of Bardane (Fjørtoft island, W Norway), that contain ortopyroxene, clinopyroxene, garnet, phlogopite, dolomite/magnesite and locally showing microdiamond inclusions (Van Roermund et al., 2002). The websterites are hosted by garnet peridotites which represent deep cratonic mantle formed in the Archean (Spengler et al., 2006): these mantle rocks have been part of a wedge involved in Caledonian orogeny and tectonically coupled with the Baltoscandian continental crust during ultradeep subduction to 200 km depth (Scambelluri et al., 2008; Spengler et al., 2009; Van Roermund 2009a). During subduction fluids released from the continental crust flushed and metasomatized the Bardane mantle (Brueckner et al., 2002, Van Roermund et al., 2002; Carswell and Van Roermund 2005; Scambelluri et al., 2008). Despite the difference in age, orogenic setting and crystallization depths, the mantle rocks from Bardane and Ulten share relevant geologic similarities as they: (1) are tectonically inserted in eclogitized HP and UHP continental rocks (Godard et al., 1996; Dobrzhinetskaya et al., 1995). (2) contain hydrates and carbonates in the HP and UHP parageneses, witnessing metasomatism due to influx of C-O-H fluids that reached carbonate saturation. Figure 17 reports the average trace element compositions of: (1) the Ulten HP clinopyroxene and amphibole, and (2) the Bardane UHP clinopyroxene and phlogopite. These represent the major trace element repositories in such rocks and the spidergram shows that clinopyroxenes from both settings have very similar patterns. The REE contents of the Bardane clinopyroxene are higher than those from Ulten: this can be explained by coexistence of the Ulten clinopyroxene with amphibole, which is a preferential LREE host and lowers the LREE budget of associated clinopyroxene. On the other hand, clinopyroxene in Bardane equilibrates with phlogopite which has low affinity for LREE: for this reason the Bardane clinopyroxene is the LREE sink. Clinopyroxene from Ulten and Bardane are similarly depleted in LILE compared with coexisting amphibole and phlogopite due to preferential LILE partitioning in the latter phases. The above discussed similarities in trace element patterns of clinopyroxenes suggest that the metasomatic agents infiltrating the mantle wedge rocks at Bardane and Ulten were compositionally similar.
Figure 16. General scenario for the Ulten peridotite evolution (after Nimis and Morten 2000).
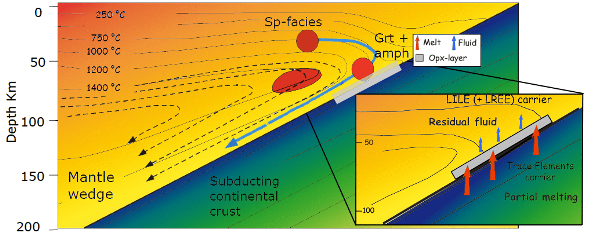
Equilibration in the spinel facies field (above a melting mantle region represented by the red area below the spinel-facies field) is followed by peridotite hydration and crystallization to garnet + amphibole assemblages in a wedge setting above the subducting crust and/or during engagement in the crust. These stages are followed by exhumation (redrawn from Scambelluri et al., 2006).
Figure 17. Comparison of the trace element compositions.
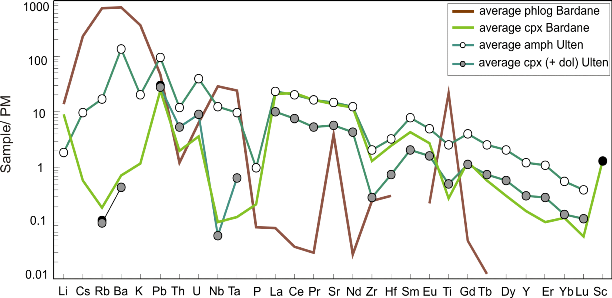
Comparison of the trace element compositions of the major trace element mineral repositories from the peak assemblages in Bardane (clinopyroxene and phlogopite, green and brown continuous lines, respectively) and Ulten Zone (clinopyroxene and amphibole, grey and white dots, respectively). Normalization as in \figure 5.
As shown in Figure 15, the Bardane peridotites exhibit lower ƒO2 values relative to the Ulten peridotites. As a consequence, such a high oxygen fugacity recorded by the Ulten peridotites inhibited carbon precipitation from the CO2-bearing metasomatic fluid (as evidenced by the location of the CCO equilibrium in Figure 15). More generally, the mantle wedge Ulten, Sulu and Bardane peridotites record a ststematic decrease of ƒO2 with depth: this trend is similar to the one defined by subcratonic mantle xenoliths (e.g. Central Asia, Lesotho and South Africa, see Fig. 15), although shifted towards higher fugacities. The above discussed similarities in metasomatic style recorded in the Ulten and Bardane peridotites, together with their relatively high ƒO2 values compared to subcratonic mantle equilibrated at similar P, suggest a relevant similarity in the oxidizing metasomatic agents infiltrating the mantle wedge rocks at both settings. This observation points that comparable metasomatic processes driven by C-O-H fluid phases have affected the mantle wedge over a large range of subduction depths, from ~ 80 km (Ulten Zone) to ~200 km (Bardane).