Petrology of the Ulten Zone peridotites
The Ulten Zone peridotites outcrop as lenses (up to 10 m thick, tens to hundreds meters long) of harzburgite, lherzolite and minor dunite intruded by pyroxenite veins (Fig. 8A). They form a discontinuous horizon broadly located between underlying weakly migmatized foliated garnet + kyanite- bearing gneisses, and overlying migmatites (Godard et al., 1996). When present, the peridotite banding and/or foliation is roughly concordant to the main planar anisotropy of the host rocks. The contacts between peridotite bodies and country gneiss are generally poorly exposed and often obscured by young faults; when exposed they are generally sharp and are locally marked by the development of hybrid Phl ± Amph ± Chl-bearing layers (Godard et al., 1996; Tumiati et al., 2003, 2007; Marocchi et al., 2007, 2010). The petrographic features of peridotites and pyroxenites have been described by Morten and Obata (1983) and Obata and Morten (1987); further details have been reported by Godard et al. (1996), Nimis and Morten (2000), Godard and Martin (2000), Rampone and Morten (2001), Tumiati et al. (2003), Tumiati and Martin (2003), Morten and Trommsdorff (2003), Tumiati et al. (2007), Scambelluri et al. (2006), Sapienza et al. (2009). Here we present a summary of major textural characteristics of these rocks.
The Ulten Zone peridotites record a polyphase metamorphic history that is documented by the transformation of coarse (up to a few cm-sized crystals) spinel-bearing lherzolites (coarse-type) to fine-grained (0.2-1 mm-sized crystals) mylonitic peridotite varieties dominated by garnet + amphibole-bearing assemblages (fine-type). The latter largely prevails over the coarse-type. The garnet + amphibole peridotites are locally associated with garnet + amphibole + dolomite-peridotites: carbonate-bearing and carbonate-free rocks occur in the same and/or in nearby outcrops (Sapienza et al., 2009). Pyroxenite veins record a similar evolution from very coarse (≤6 cm) clinopyroxenites to fine-grained garnet + amphibole ± olivine- bearing websterites (Morten and Obata, 1983; Nimis and Morten, 2000).
Coarse spinel-facies peridotites with coronitic garnet
The coarse spinel peridotites are restricted to weakly deformed domains within some peridotite bodies. They preserve protogranular textures (Fig. 8B) and display olivine, ortho, clinopyroxene and spinel showing equilibrium textures. Amphibole is absent within the above spinel-facies assemblage and occurs as secondary phase along the pyroxene grain boundaries. Thermobarometry of the spinel lherzolites yields ~1200°C and 1.3-1.6 GPa. T ≥ 1400°C and comparable pressures have been obtained for the early crystallization of the associated pyroxenites (Nimis and Morten, 2000). In the coarse spinel-bearing peridotites, garnet occurs in reaction coronas around spinel (Fig. 8B, C) and in granoblastic aggregates in equilibrium with neoblastic pyroxene and olivine. Coarse ortho- and clino-pyroxene in these rock varieties show along cleavages complex exsolutions and/or replacement textures made of pyroxene, garnet, amphibole and subordinated spinel along cleavages (Godard et al., 1996). In some samples, neoblastic edenitic-pargasitic amphibole occurs in the fine-grained granoblastic garnet-bearing domains; these domains can additionally contain dolomite and apatite, to form a stable assemblage with olivine, orthopyroxene, amphibole and garnet. The garnet assemblages developed by cooling to ~850°C and P increase to ~2.7 GPa of the spinel peridotites (Fig. 6; Nimis and Morten, 2000). Maximum pressure and temperature estimates are also provided by the presence of amphibole and dolomite in the garnet bearing assemblage. These two minerals in peridotite systems can survive up to maximum pressures of 3 GPa (for amphibole, Fumagalli and Poli, 2005) and 2 GPa for dolomite at temperatures of 900 °C (Tumiati et al., 2009). The coexistence of amphibole and dolomite has been experimentally determined for KNCFMAS + C-O-H systems up to 1.8 GPa and 900-1000 °C (Tumiati et al., 2009). Maximum temperatures for dolomite stability are provided by the carbonate solidus located at 930-1080 °C and a pressure range of 2.1-3 Gpa for hawaian pyrolite compositions (Wallace and Green, 1988) or at 1060 and 2.6 GPa for KNCFMAS + C-O-H systems (Tumiati et al., 2009). Using the experimental data of Wallace and Green (1988), Sapienza et al. (2009) determined maximum 2.5 GPa and 850 °C, for crystallization of the garnet + amphibole + dolomite assemblage in the carbonated garnet peridotites of the Ulten Zone.
Figure 8. Meso- and micro-structures of coarse spinel-facies peridotites with coronitic garnet.
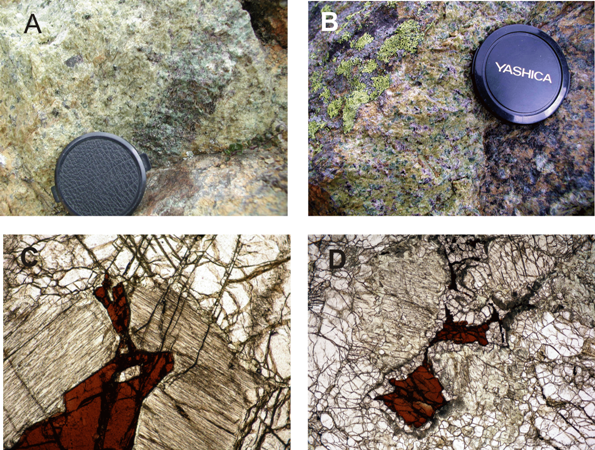
A: pyroxenite vein inside the spinel peridotite. B: spinel peridotite with coronitic garnet. The black crystals in the peridotite are spinel grains, pink garnet forms clear corona textures around spinel. C: transmitted light photomicrograph of a spinel peridotite with fresh unreacted spinel grains (brown; about 1 mm long) showing equilibrium texture with exsolved orthopyroxene. The coarse white mineral at the top right corner is olivine. D: transmitted light photomicrograph of spinel grains (brown; 0.5 mm long) with garnet coronas. They are surrounded by coarse exsolved clinopyroxene (pale green), orthopyoroxene and olivine (white coarse mineral in the lower half of the picture).
Figure 9. Meso and microstructures of mylonitic (garnet + amphibole ± dolomite) peridotites.
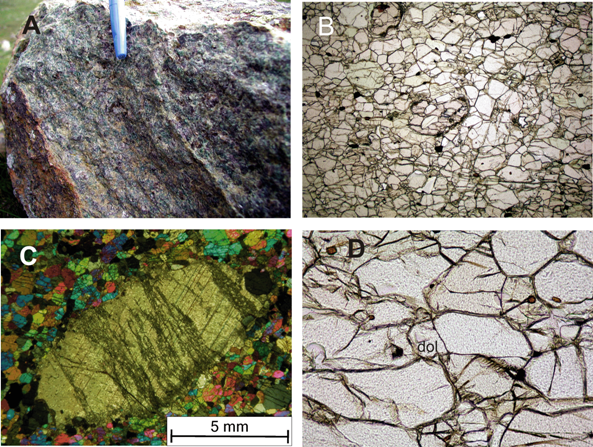
A: mylonitic (garnet + amphibole) peridotite. The main foliation dips from left to right side of the photograph. It consists of amphibole and finegrained garnet associated with olivine, clino and orthopyroxene. B: transmitted light photomicrograph of the mylonitic foliation, with stable garnet (pink crystal at the centre of the picture), amphibole (pale green), olivine (white), clino and orthopyroxene. Image width is 5 mm. C: transmitted light photomicrograph (crossed nicols) of a dolomite porphyroclast surrounded by apatite grains and showing internal recrystallization along microfractures. The porphyroclast is set in a fine-grained matrix of (garnet + amphibole)–harzburgite composition. D: transmitted light photomicrograph of a dolomite neoblast in the matrix. Foliation is defined by the preferred orientation of elongated amphibole.
Mylonitic garnet + amphibole ± dolomite peridotites
Widespread shearing, hydration and sinkinematic recrystallization during the high-pressure stage produced fine-grained peridotites characterized by dominant tabular to mosaic granular textures (Fig. 9). These rocks mostly consist of granoblastic olivine + orthopyroxene + garnet + amphibole aggregates, with minor or no clinopyroxene and rare dolomite, phlogopite and apatite (Obata and Morten, 1987). Transition from coarse to fine types is testified by porphyroclastic garnet-bearing samples. In the fine-type, amphibole is modally abundant (13-23 vol %) and ranges in composition from pargasite-edenite cores with up to 1 wt% K2O to Mg-hornblende rims (Obata and Morten, 1987; Nimis and Morten, 2000; Rampone and Morten, 2001; Tumiati, 2002). Increase in modal amphibole is associated with progressive disappearance of clinopyroxene and, eventually, garnet as a response to increasing hydration (Obata and Morten, 1987). Hydration was locally accompanied by development of centimetre-sized veinlets of euhedral garnet plus pargasite-edenite, corresponding to zones of fluid influx (Tumiati et al., 2003). The mylonitic garnet + amphibole peridotite varieties are locally associated with dolomite-bearing peridotites. The carbonated peridotites contain millimetre- to centimetre-sized prophyroclasts of dolomite, garnet (enclosing relic spinel) and weakly zoned amphibole set in a finer-grained mylonitic matrix (< 1 mm sized). Dolomite porphyroclasts are about 1 cm in size (Fig. 9c): they display fine-scale internal recrystallization along microfractures and are surrounded by apatite grains (up to 1.3 mm). A second generation of HP minerals occurs in the foliated matrix, which consists of olivine, orthopyroxene, elongated amphibole, garnet, dolomite and clinopyroxene (Fig. 9d). Modal estimates by Obata and Morten (1987) indicate that these samples may contain 72% olivine, 16% orthopyroxene, 3.4% amphibole, 7.9% garnet; clinopyroxene, dolomite and apatite are present in subordinate amounts. The occurrence of dolomite and apatite in the fine-grained garnet peridotite domains suggests their equilibrium crystallization at garnet-facies conditions. Mineral compositions and semiquantitative thermobarometry suggest that the mylonitic garnet + amphibole peridotites developed at roughly the same conditions as the coronitic garnet assemblages in the coarse peridotites (Obata and Morten, 1987; Tumiati et al., 2003).
Retrogression of the garnet-bearing mineral assemblages is recorded by (i) mineral zoning (Nimis and Morten, 2000; Tumiati et al., 2003), (ii) formation of symplectites (orthopyroxene + clinpyroxene + spinel and orthopyroxene + amphibole + spinel) after garnet (Godard and Martin, 2000), and (iii) complete garnet and clinopyroxene replacement of amphibole + spinel (Susini and Martin, 1996; Scambelluri et al., 2006; Marocchi et al., 2007). The retrograded peridotites rarely preserve relicts of garnet and pyroxene and generally consist of amphibole + orthopyroxene + olivine + spinel ± phlogopite (modal amphibole content up to 20 vol%). Occasionally, a new REE(La)-epidote (dissakisite) occurs in fine-grained spinel + amphibole- bearing peridotite deriving from retrogression of former garnet-bearing peridotite (Tumiati et al., 2005). Further retrogression produces olivine + tremolite + cummingtonite + chlorite + talc and widespread serpentinization. Peridotite lenses locally display reaction rims towards the contact with the host migmatized gneiss. These derive from peridotite-gneiss interaction either during exhumation of the crust-mantle association (Marocchi et al., 2010) or, alternatively, during subduction (Tumiati et al., 2007). These reaction zones are dm-thick bands, well exposed in the Mt. Hochwart area (northeastern Ulten Zone), which display a layered distribution of metasomatic minerals. From migmatite to peridotite the following zones can be distinguished (Marocchi et al., 2010): phlogopitite ± tremolite ± apatite ± zircon (zone 1), tremolite + phlogopite + anthophyllite (zone 2) and anthophyllite ± chlorite ± talc (zone 3). Locally, Mg-hornblende-rich domains with accessory Cl-rich Fe-kinoshitalite, a rare Ba-rich mica (Tumiati et al., 2007). These hybrid reaction layers display very high contents of fluid-mobile elements. whereas Li is preferentially hosted by anthophyllite. The nature of the metasomatic aqueous fluids responsible for the crystallization of the metasomatic bands is debated. According to Marocchi et al. (2010) the reaction zone has been produced by infiltration of hydrous fluids at T ≈ 660–700 °C and P < 1.2 GPa, during exhumation of coupled continental crust and mantle peridotites. Conversely, based on the occurrence of Cl-rich apatite and Cl-rich Fe-kinoshitalite in the reactions bands, Tumiati et al. (2007) proposed that metasomatic reactions were enhance by deep infiltration of ocean water in the subduction channel.