Carbon-cycling through subduction beneath the Italian region
Mantle structure
Peridotite xenolith suites testify for a depleted mantle lithosphere, refertilized in orogenic regions by conspicuous metasomatic processes induced by hydrous silicate ± carbonate melts of subductive origin. At greater pressures, the most prominent feature evidenced by geophysical data is the occurrence of a low-velocity layer from about 70 to 120 km depth (1.8-4.0 GPa) that extends from the south-eastern Provence to the south-eastern Tyrrhenian Sea areas (Fig. 3). This coincides with the zone of slab migration from Oligocene to present and it is associated with orogenic magmatism of the same age, which migrated east- and southeast-ward as an effect of slab rollback (Panza et al., 2007a). In this view, the low-velocity layer represents a sort of tail of mantle anomalies left behind by the retreating slab. According to Panza et al. (2007a), the upraise to shallow depths of this layer beneath the Campanian area is related to the eastward mantle flow above the retreating slab. Peccerillo et al. (2008) explain this low-velocity layer as the effect of geochemical anomalies generated by the subducting slab during rollback. An important piece of evidence in support of this possibility is the absence of such a layer in zones which were not affected by recent subduction (i.e., continental France and Spain, and Southern Sicily). In the latter, where the well-known ephemeral volcano of Ferdinandea (Graham) island was formed in 1831, the thin low-velocity layer observed offshore, west and south-west Sicily, likely reveals local zones of melting, possibly due to lithospheric decompression along strike-slip faults.
A main question is the identification of the process that generated this layer, i.e. of which components were released from the slab to modify the upper mantle. Also, the occurrence of a low-velocity layer in areas that are not affected by active or recent magmatism, such as the central Tyrrhenian area, needs to be understood. Low VS waves might be induced by basaltic melts, generated by partial mantle melting processes at elevated temperatures (e.g., Goes and Van der Lee, 2002; Cammarano et al., 2003). Alternatively, the observed low VS might correspond to a level of C-O-H volatile enrichment (i.e. H2O bound in mantle mineral’s lattice or at grain-boundaries ± carbonates, and/or to free H2O, CO2-rich fluids or melts) which may induce composition and density variation in the mantle (Gaetani and Grove, 1998; Jung and Karato, 2001; Presnall and Gudfinnsson, 2005; Dasgupta and Hirschmann, 2006).
The presence of a low velocity layer between 60-70 km to 120-130 km of depth (1.8-4.0 GPa) may be explained by the presence of C-O-H fluid phases (free or mineral-bound) generated by the subducted slab, during the rollback of the west-directed Adriatic-Ionian subduction. It is unlikely that the decrease, with increasing depth, of seismic wave velocities indicates the presence of a 60-km-thick layer with low fractions of basaltic melts, since a continuous supply of more than 1-4% in volume of basaltic melt is required to allow migration through the whole mantle zone (Hyndman and Shearer, 1989). Similar amounts of basaltic melts are highly improbable, since magmatism is not any more active at present. In addition, it would necessarily require considerably high temperatures in the mantle, which are not compatible with the heat flux along the overall trajectory of section 1 (Fig. 3).
CO2 mantle degassing processes
Frezzotti et al. (2009) proposed that the low velocity layer observed between 70 km and 130 km of depth may represent a low viscosity wedge beneath the western Mediterranean, induced by the presence of carbonate-rich melts. The confinement of mantle physical modifications at P > 2 GPa suggests a major role of CO2 than of H2O. In the peridotite-CO2 systems (i.e. CO2 fluids, carbonate melts, and carbonates), 2 – 2.5 GPa corresponds to the pressure at which the solidus of carbonated-peridotite is considerably depressed (Fig. 5; Dalton and Presnall, 1998; Gudfinnson and Presnall, 2005): peridotite melting would begin generating low fractions of carbonate-rich melts.
Figure 5. Proposed evolution for lithosphere - asthenosphere degassing beneath the Western Mediterranean, modified from Frezzotti et al. (2009).
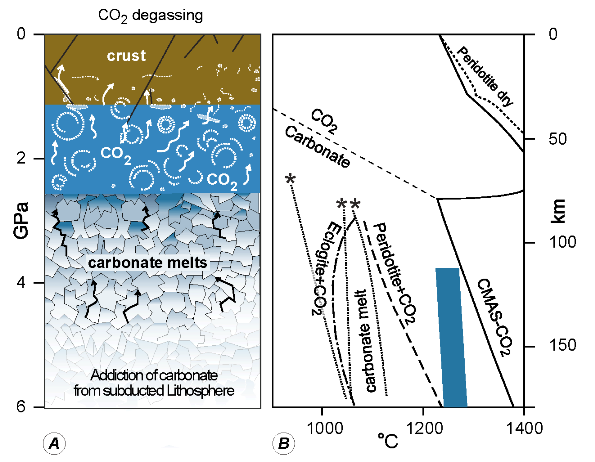
a) Pressure-temperature diagram showing the effects of CO2 on the solidus of carbonated lithologies in the mantle. Two different estimates of the peridotite - CO2 solidus are reported: CMSA - CO2 after Dalton and Presnall (1998), and Gudfinnsson and Presnall (2005), and peridotite - CO2 (2.5 wt %) from Dasgupta and Hirschmann (2006). Dry peridotite solidus in the CMAS system is from Gudfinnsson and Presnall (2005). Eclogite - CO2 solidus (dry eclogite + 5 wt % CO2) from Dasgupta et al. (2004). Asterisks (*) corresponds to the KNCFMASH - CO2 solidus (carbonated pelite + 1.1 wt.% H2O + 4.8 wt.% CO2) from Thomson and Schmidt (2008). The effect of carbonates on the composition of melts generated at increasing temperature is reported as wt% CO2, based on the CMSA – CO2 system. Gray area = estimated present-day mantle temperatures at the inferred pressures (Carminati et al., 2005). b) Application of the experimentally determined melting relationships for carbonated peridotite, and crustal lithologies to illustrate present mantle processes and metasomatism beneath the Western Mediterranean. Melting of sediments and/or continental crust of the subducted Adriatic-Ionian (African) lithosphere, generates carbonate-rich (hydrous-silicate) melts at pressure > 4 GPa (130 km) and T > 1260°C. Due to their low density and viscosity, such melts can migrate upward through the mantle, forming a 70 km thick carbonated partially molten CO2-rich mantle layer recorded by tomographic images. Upwelling of carbonate-rich melts to depths less than 60 - 70 km, induces massive outgassing of CO2 in the lithospheric mantle.. Buoyancy forces, probably favored by fluid overpressures, and tectonics might allow further CO2 upwelling to the Moho and the lower crust, and, ultimately, outgassing at the surface.
At pressures higher than 2 GPa, carbonates and carbonate-rich melts are stable; at lower pressures, they decompose releasing CO2 fluids (Canil, 1990; Yaxley and Green, 1996). These properties of CO2 at depth satisfy the variations of the physical properties of the mantle from 2 GPa to 4 GPa: carbonate-rich melts have very low interfacial energies with respect to mantle minerals. Dihedral angles (θ) are in the range of 25°–30° and allow carbonate melts to rise through and react with mantle minerals, which may well account for the low VS detected at P > 2 GPa (Hunter and McKenzie, 1989; Watson and Brenan, 1987; Watson et al., 1990). Conversely, the dihedral angles (θ) between CO2 fluids and mantle minerals are greater than 60° and inhibit the formation of an interconnected fluid network with cessation of VS reduction (Watson and Brenan, 1987): CO2 pooling should occur associated to CO2 outgassing of carbonate melts in the mantle below 2 GPa.
The addition of a carbonate component into the western Mediterranean mantle might have been induced by subduction processes of sediment-bearing old oceanic and/or continental Adria lithosphere either by decarbonation, or by melting reactions. However, decarbonation reactions during subduction represent an uncommon process, since carbonates are very stable phases to very high pressures and temperatures (Kerrick and Connolly, 2001a and b; Connolly, 2005). Fluid-absent partial melting of carbonated-hydrated pelites necessitates of even higher temperatures to generate carbonate ± silicate melts (T=1100 °C- P=2 -5 GPa; Dasgupta etal., 2004; Thomsen and Schmidt, 2008).
Foregoing processes require temperatures that are unrealistic for active subduction mantle geotherms (van Keken et al., 2002). According to Frezzotti et al. (2009) decarbonation or melting processes of the Adria lithosphere should have been induced successively, during a progressive rise of mantle temperatures, induced by the combined effect of the strong extensional tectonics affecting this sector of the Tyrrhenian basin and of the eastward mantle flow (Panza et al. 2007a), facilitated by the eastward retreating Adriatic-Ionian subducting plates. This hypothesis implies that, in this area, the crust of the retreating subducted slab remained heterogeneously trapped in the mantle at a depth of about 130 km and more.
Lithospheric CO2 degassing path
Based on a similar model, high amounts of CO2 can be transported above the deep subducted slab from a depth of about 130 km, in a timescale of a few hundreds of ky. Upwelling of carbonate-rich melts from upper mantle depths is geologically fast, due to their low viscosity and density, and can reach values of the order of tens of cm/y (Hammouda and Laporte, 2000).
At lithospheric depths ≤ 70 km, above the carbonate stability field, massive CO2 fluxes are produced by outgassing of carbonate melts (Fig. 5). At similar mantle depths, CO2 fluids have very high density (1.15 – 1.2 g/cm3 from 60 km to 80 km, at 1000°C; Frezzotti and Peccerillo, 2007), and are very compressible, but virtually immobile (e.g. θ > 60°). Released CO2 fluids will tend to pool above this pressure threshold to form diffuse and/or concentrated gas-rich regions in the upper mantle (e.g., isolated small pockets confined at mineral grain boundaries). We cannot, however, exclude that CO2 fluids may coalesce to form larger overpressurized reservoirs, which could facilitate earthquakes.
In Italy, non-volcanic CO2 degassing is associated with the main crustal geological features, and active tectonics has been proposed as the driving mechanism for CO2 release from crustal depths (e.g., Miller et al., 2004). The CO2 migration path from the lithospheric mantle is more complicated. Deep faults, such as the 41° N parallel line, could allow fast upward CO2 fluxes, and accumulation in the lower crust (Fig. 5; see Mofete d’Ansanto in Table 1). A similar process, however, cannot account for CO2 mantle rise beneath the Apennines or Tuscany.
The very recent GPS measurements and the unusual subcrustal seismicity distribution have revealed the role of buoyancy forces with respect to the ongoing slow and complex lithospheric deformations in the uppermost mantle along central Italy (Audia et al.,2007; Panza and Raykova, 2008; Ismail-Zadeh et al., 2010) . These authors proposed that buoyancy forces, resulting from the heterogeneous density distribution in the lithosphere govern the present-day deformation within Central Italy. Upper mantle buoyancy may explain the upwelling, towards the base of the crust, of otherwise immobile CO2.
Etna CO2 emission
CO2-magma degassing is a normal feature of many OIB-type volcanoes worldwide. However, Etna emits about 1-2 orders of magnitude more CO2 than most measured OIB volcanoes (see Table 2). Interaction between magma and carbonate wall rocks (Grasso, 2001) seems to be a minor process at mount Etna (Marty et al., 1994), where the anomalous CO2 emission appears to be mantle-related (Allard et al., 1997; D’Alessandro et al., 1997).
Geochemical studies of present magmatic activity have highlighted the presence of a subduction fluid-component in Etna magmas, testified by enrichments in K, Rb and variation in Sr, B, and Ne-isotope ratios (Condomines et al., 1995; Tanguy et al., 1996; Nakai et al., 1997; Schiano et al 2001; Tonarini et al., 2001; Allard et al., 2006). Gvirtzman and Nur (1999) and Doglioni et al. (2001) pointed out that Etna is located along a main lithospheric fault zone running from Lipari and Vulcano (Aeolian arc) to the Malta escarpment. According to these Authors, magmatism is generated at a window along this fault, at the boundary between the border of the African plate and the southeastward retreating Ionian slab. This would allow contemporaneous OIB-type mantle decompression and melting, and CO2-rich fluid inflow from the undergoing slab in the Etna magmatic system.
An alternative explanation for the origin of deep CO2 is that the enriched mantle source is represented by the carbonate sequences of the subducted Iblean foreland. The low dipping angle of the Iblean foreland (Doglioni et al., 2001, and references therein) and the absence of deep seismicity beneath the western Aeolian arc suggest sinking and foundering of Iblean slab within the upper mantle, which could release large amounts of CO2 when conditions of carbonate stability have been reached.