The Italian Mantle
It is generally agreed that the bulk of the CO2 degassing in Italy should reflect deep mantle processes, with crustal contributions being significant only at a few active or recent volcanoes (Allard et al., 1997; Chiodini et al., 2000; Minissale, 2004; Gaeta et al., 2009; Iacono Marziano et al., 2009). Therefore, the clue to understand present-day massive Earth’s CO2 degassing in Italy ultimately resides in a careful knowledge of mantle processes and of their geodynamics.
The evolution of the Italian region and of the entire Western-Central Mediterranean area has been the effect of the Oligocene to Present convergence between the African and Eurasian plates. This led to the opening of the Ligurian-Provençal, Algerian, and Valencia and Tyrrhenian basins, the formation of the Apennine chain and the emplacement of a wide variety of both orogenic and anorogenic magmas (see Alagna and Peccerillo, this issue; Bianchini et al., this issue; Carminati et al. this issue).
Orogenic magmatism started in Provence, Balearic sea and Sardinia (Oligo-Miocene) and migrated eastward in the Tyrrhenian Sea floor, in the Italian peninsula and Aeolian arc (Miocene to present). Compositions are mainly calcalkaline but potassic ultrapotassic rocks dominate in the Italian peninsula (e.g. Francalanci et al., 1993; Peccerillo, 2003; Alagna and Peccerillo, this issue). Eastward migration of orogenic magmatism is related to slab rollback in the same direction (Carminati et al., 1998; Doglioni et al., 1999, and references therein). Anorogenic magmatism around the Tyrrhenian Sea is also variable in composition, from tholeiitic to Na-alkaline, but does not show any apparent regional migration with time, although in single areas (e.g. Sardinia) it follows orogenic activity (Savelli and Gasparotto, 1994; Lustrino et al. 2004, 2007a, for a review).
Peridotite xenoliths
Peridotite xenolith suites are present in Pliocene-Quaternary orogenic volcanics in continental Italy at Monte Vulture (0.2 M.y.), and at Torre Alfina (0.9 M.y.), and in the Miocene-Quaternary anorogenic mafic volcanics of Monti Iblei in Sicily, and in Sardinia (Fig. 1; e.g., Conticelli and Peccerillo, 1990; Conticelli, 1998; Jones et al., 2000; Sapienza and Scribano, 2000; Downes, 2001; Beccaluva et la., 2001; Downes et al., 2002, and references therein).
Peridotite suites consist mainly of spinel lherzolites, harzburgites, and dunites, and may contain variable amounts of metasomatic phlogopite and amphibole (Table 3). Overall, geochemical and petrological features of peridotite xenolith suites indicate a depleted mantle lithosphere beneath the Italian region resulting from ancient melting events, variably enriched by subsequent fluxes of metasomatic melts and/or fluids. In orogenic mantle settings, carbonate and hydrous-silicate melts have been proposed as the main mantle metasomatic agents by various authors (Conticelli and Peccerillo, 1990; Conticelli, 1998; Jones et al., 2000; Downes et al., 2002; Rosatelli et al., 2007).
At Monte Vulture volcano, spinel peridotites consist of lherzolites and harzburgites, with subordinate dunites, and wehrlites, and pyroxenites (Fig. 2a), which may contain metasomatic phlogopite, amphibole, and carbonate. Peridotites equilibrated at pressures of 1.8 - 2.2 GPa (about 60-80 km of depth), and temperatures of 990 – 1150°C in the lower part of the spinel stability field (Table 3). Metasomatic enrichment processes in peridotites are testified by presence of mica, carbonates, and silicate glass in melt inclusions and microveins (Fig. 2b), and by LILE and LREE enrichments in clinopyroxene (Downes et al., 2002; Rosatelli et al. 2007). According to Downes et al. (2002), peridotites show 87Sr/86Sr ratios higher than in most of the European continental lithosphere (87Sr/86Sr = 0.7042–0.7058), suggesting that metasomatic melts could have been subduction related.
Figure 2. Photomicrographs of xenolith spinel peridotites from Vulture and Torre Alfina volcanoes.
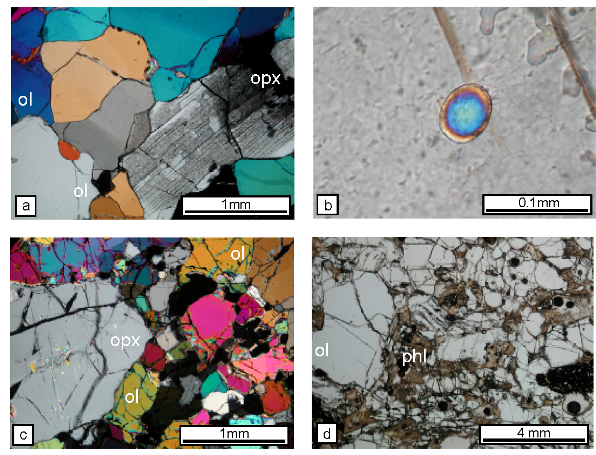
a) harzburgite from Vulture. Olivine porphyroclasts show undulouse extinction, crossed polars (CP). b) Isolate carbonate inclusion in olivine neoblast from Vulture dunite. Rounded contours of the carbonate inclusion suggest trapping as melt phase, CP. c) Harzburgite from Torre Alfina. Two generations of olivine are recognized: large olivine porphyroclasts surrounded by medium-grained neoblasts, CP. d) Phlogopite from dunite, Torre Alfina. Phlogopite is mainly interstitial, but locally crosses single grains of anhydrous minerals. thick section, plane-polarized light.
In the orogenic lamproitic lavas of Torre Alfina volcano (Tuscany), spinel peridotite suites consist of dunites, harzburgites, and minor spinel lherzolites that may contain metasomatic phlogopite (Fig. 2c and d). Peridotites represent samples of a “hot” lithosphere, close to the asthenosphere-lithosphere boundary: recent geothermobarometric studies indicate equilibrium pressures of about 1.6 GPa, corresponding to a depth of 50 to 60 km, and temperatures of 950 – 1080°C (Table 3; Pera et al., 2003).
In Torre Alfina peridotite xenoliths, metasomatic phlogopite may be present in traces, disseminated through the rock, or may constitute up to 10% of the rock by mode, distributed along short alignments (Fig. 2d). Phlogopite-rich xenoliths have been interpreted as remnants of pervasive metasomatic events in the upper mantle (Conticelli and Peccerillo, 1990). These have Sr-Nd (87Sr/86Sr ~ 0.716 - 0.717; 143Nd/144Nd ~ 0.5121) isotopic signatures close to those of the host ultrapotassic rocks and have been suggested to represent the metasomatic veins, whose melting gave ultrapotassic magmas (Conticelli, 1998).
Table 3. Peridotite xenolith suites in Italy
Location | Rock | Ol | Mg# | Opx | Mg# | Cpx | Spinel | Amph | Phlog | Temperature | Pressure | Citation |
---|---|---|---|---|---|---|---|---|---|---|---|---|
vol. % | vol. % | vol. % | vol. % | vol. % | vol. % | C | GPa | |||||
Torre Alfina | Harzburgite Dunite Lherzolite | 80-95 | 0.90-0.92 | 4-12 | 0.91-0.93 | <1-8 | 1-2 | <1 -10 | 950-1080 | 1.6 ± 0.2 | Peccerillo & Conticelli 1990; Conticelli 1998; Pera et al. 2003 | |
Vulture | Cpx-poor Lherzolite Harzburgite | 60-86 | 0.90-0.91 | 8-30 | 0.91-0.93 | 1-10 | 1-2 | 1 | <1 | 992-1150 | 1.8 ± 0.3 | Jones et al. 2000; Downes et al. 2002; Rosatelli et al. 2007 |
Sardinia | Cpx-poor Lherzolite Harzburgite | 60-80 | 0.89-0.91 | 10-30 | 0.90-0.92 | 4-16 | 1-3 | 950-1050 | 1.4 ± 0.2 | Beccaluva et al. 2001; unpublished data | ||
Iblei | Harzburgite Lherzolite | 60-77 | 0.90-0.92 | 10-30 | 0.87-0.91 | 3-10 | 1-4 | <1 | <1 | 950-1050 | 1.1 | Sapienza et al. 2005; Cristofolini et al. 2009 |
Ol= Olivine; Opx= Orthopyroxene; Cpx= Clinopyroxene; Amph= Amphibole; Phlog= Phlogopite; Mg#= Mg/(Mg/Fetot) |
VS tomography
S-wave velocity tomography in the western Mediterranean and the Thyrrenian sea area is illustrated in Fig. 3. All reported data have been recently published in Panza et al. (2007a, and b), Boyadzhiev et al. (2008), and Peccerillo et al. ( 2008), where detailed information about the data, path coverage, resolution, etc. can be found. Updated information is given by Brandmayr et al., this issue. Section 1 (Fig. 3a) goes from Provence to Central Sardinia and to the Campanian area, running along the 41° Parallel Line in its central and eastern segments (Panza et al., 2007a). Section 2 (Fig. 3b) runs from offshore southern Sardinia to the Aeolian arc and Calabria following an E-W and then NW-SE direction (Boyadzhiev et al., 2008; Peccerillo et al., 2008). Section 3 (Fig. 3b) is located in the central-northern Tyrrhenian Sea and goes through the Tuscany and Roman magmatic provinces (Panza et al., 2007b).
Section 1 running from Provence to Sardinia, central Tyrrhenian Sea, up to the Campanian volcanoes (Vesuvio, Campi Flegrei) (Fig. 3) shows variable features in the lithosphere-asthenosphere system. The upper mantle beneath continental France shows rather constant S-wave velocities (VS ~ 4.3-4.5 km/s) down to 250 km of depth. Starting from off-coast Provence, the upper mantle structure changes significantly because of the presence of a layer with relatively low S-wave velocities (VS = 4.0-4.1 km/s) at a depth of about 70-120 km.
Figure 3. VS models of the lithosphere-asthenosphere system along the TRANSMED III geotraverse
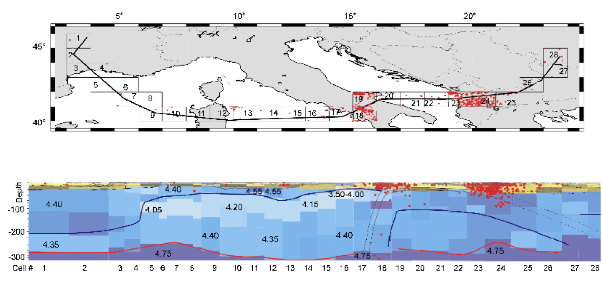
Modified from Panza et al. (2007a); the geometry of the base of the lithosphere is indicated by the blue line; the limit between upper and lower asthenosphere is indicated (red line).
Figure 4. Sections 2 and 3, built from the cellular VS model of the Tyrrhenian Sea and surroundings (Panza, 2007a).
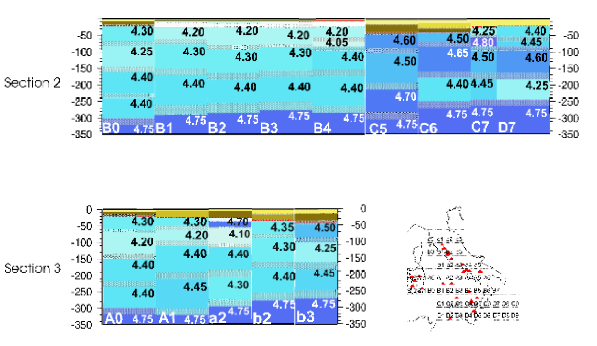
In each labeled cell, the hatched zone stands for the thickness variability, while, to avoid crowding of numbers, only the average shear velocity is reported. The VS ranges of variability are given in Panza et al. (2007a and b). Red triangles indicate recent and active volcanoes.
This low velocity layer is delimited, at the top and at the bottom, by high-velocity material (VS ~ 4.30-4.55 km/s) and it extends eastward from offshore Provence to the Magnaghi and Vavilov basins, with almost constant thickness. From the Pontine Island to the Campanian area, the low velocity layer raises to a shallower level, with a decrease of VS that can be as low as about 3.4 km/s. A low velocity layer beneath the Western Mediterranean area was also detected by P-wave tomography (Hoernle et al. 1995) and was interpreted as an isolated portion of a large scale mantle up-welling affecting the Western Atlantic and Central-Southern Europe.
In section 2 (Fig. 4), which goes from Sardinia to the southeast, crossing the Aeolian Arc and Calabria, the low velocity layer is much shallower being detected at 30 km to 70 km of depth and rising to 0-30 km beneath the eastern active Aeolian volcanoes. An almost vertical seismically active high-velocity body with VS from 4.50 to 4.80 km/s likely representing the descending Ionian slab (Panza et al., 2003), interrupts the low velocity layer beneath Calabria.
Finally, section 3 (Fig. 4) goes across the central Tyrrhenian Sea, the Tuscany and Roman magmatic province and the Apennines. In its western segment, it is characterized by a low velocity layer with VS = 4.1-4.2 km/s at about 60-120 km of depth that becomes shallower in the Roman Province (about 30-50 km).