After the collisional phase a relative high heat flow rate was maintained in the lower and middle crust (until ca. 560 Ma; Söllner et al. 2000; Pedrosa Soares et al., 2001). From 560 to 535 Ma there seems to have occurred a tectonic relaxation along the entire belt, save for some local movements along ductile shear zones and slight refolding of regional fold. This tectonic break is also followed by a break in the magmatic activity. A new magma influx started around 535 Ma and lasted until 480 Ma defining the G5 late post-collisional suite.
What kind of geological mechanism could have caused such an interruption and then its continuation as a prominent bimodal magmatic activity? This section summarizes the literature aimed at answering this question.
G5 is comprised of several complexly zoned plutons (unit 10 in Figure 2) intruded high-grade gneisses at high angles to the foliation of the enclosing rocks (Figure 15). This suite is characterized by compositions varying from orthopyroxene-gabbro to granite and corresponds to the latest magmatic event of the orogen. One of the most outstanding features of these plutons is the widespread evidence of magma mingling. The deep erosion levels in the region, together with vertical exposures of over 500 m reveal the internal structure of the plutons.
Figure 15. Várzea Alegre and Santa Angélica Intrusive Complex
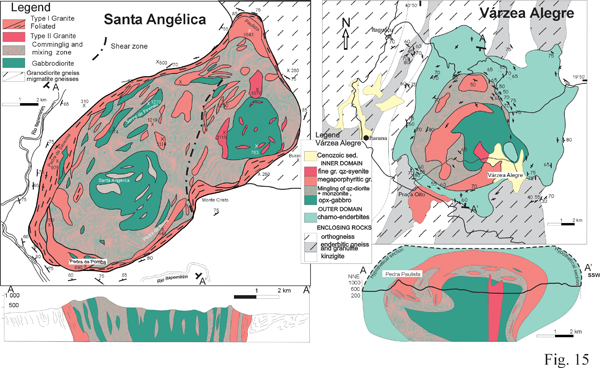
Várzea Alegre and Santa Angélica Intrusive Complex. Geology of VAIC by Medeiros; Mendes and Wiedemann. Geology of enclosing rocks of VAIC from Tuller (1993). Geology of SAIC from Bayer et al. (1987).
The Várzea Alegre Intrusive Complex (VAIC) is an almost circular pluton of approximately 150 km2 (Figure 15). It shows an inversely zoned structure, with gabbro and diorite in the center, surrounded by coarse-grained granite and an outer domain of megaporphyritic dark green charnockitic rocks towards the borders [Mendes et al., 1997, 1999; Medeiros et al, 2000, 2001, 2003]. Country rocks are G2 hypersthene-garnet-plagioclase granulites and Paraíba do Sul (Ubu series) cordierite-garnet-biotite metasedimentary gneisses with marked NE-SW striking gneissic foliation.
The outer domain of the intrusion consists of apatite-zircon-allanite-magnetite-hypersthene-mesoperthite quartz-diorite, granodiorite and quartz-monzonite. The domain varies in width from hundred meters, at the southern and western borders, to almost 4 km, at the eastern and northern borders, forming an expressive topography of 'sugar loaves'. When exposed, the contact to the country rocks is sharp. By contrast, the contact between the charnockites and the internal gabbro/dioritic/granitic domain is ductile and interfingered (Figure 16). The internal magmatic foliation is steeply to moderately dipping (Figure 15).
Figure 16. Várzea Alegre Intrusive Complex
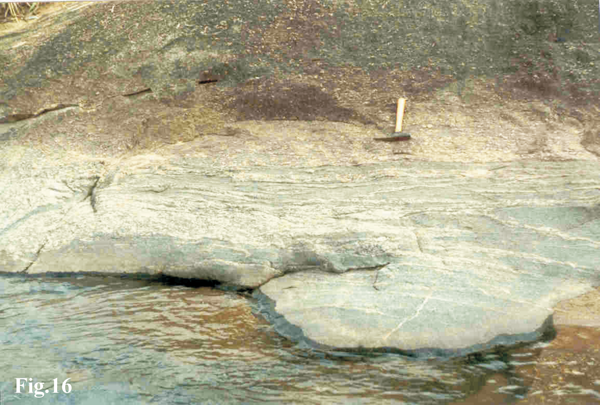
Várzea Alegre Intrusive Complex: interfingered and ductile contact between the charnockite and the internal gabbro/dioritic/granitic domain.
The internal domain consists of a preferentially eroded dark gray apatite-zircon-clinopyroxene (salite-augite)-hypersthene-K-feldspar-plagioclase (An50-65) gabbro/monzogabbro, surrounded by diorite/quartzdiorite-monzodiorite and light megaporphyritic granite (Figure 17). A late stock of sphene-bearing syenogranite cross cuts the orthopyroxene gabbro [Medeiros et al., 2001]. A mingled/mixed zone, consisting of quartz-diorite and quartz-monzodiorite, is characteristic of the contact between the megaporphyritic granite and diorite.
Figure 17. Foliated coarse grained granite from Várzea Alegre Complex
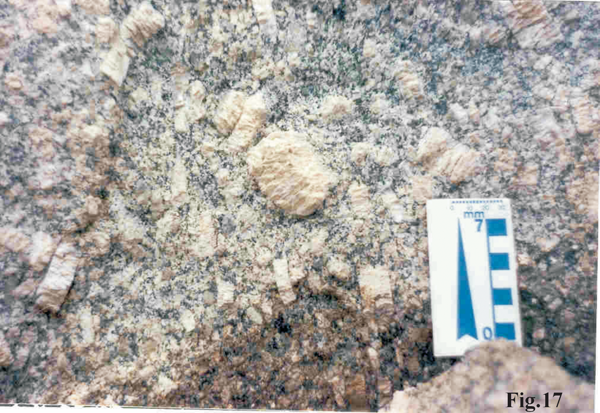
Foliated coarse grained granite from Várzea Alegre Complex. K-feldspar grains up to 6 cm.
The Santa Angélica Intrusive Complex (SAIC, Figure 15) is one of the most interesting examples of the post-collisional plutons in this mobile belt [Wiedemann et al., 1986; Bayer et al., 1987] and 5 Outcrops are described in detail in Appendix 1: Field Guide. The SAIC covers about 200 km2 and is an elliptical-shaped intrusion composed of several roughly concentric lens-shaped granitic layers, elongated lenses of gabbro-diorite and tightly packed heterogeneous enclave swarms. The complex intrudes an antiformal structure with a northwest plunging hinge, in contrast to other major fold hinges that plunge northeast. The country rocks are high-grade biotite-garnet-sillimanite gneisses (Paraíba do Sul gneiss) and biotite-hornblende granodioritic to tonalitic gneisses (G1 suite). These are locally migmatized, showing sub-vertical concordant foliation to the borders of the intrusive complex, which dip inwards towards the intrusion. The intensity of migmatization is stronger close to the pluton contact, where the gneisses exhibit nebulitic fabrics consistent with partial melting (Figure 18).
Figure 18. Schematic drawing of the contact between the Santa Angélica Intrusive Complex and the enclosing gneisses
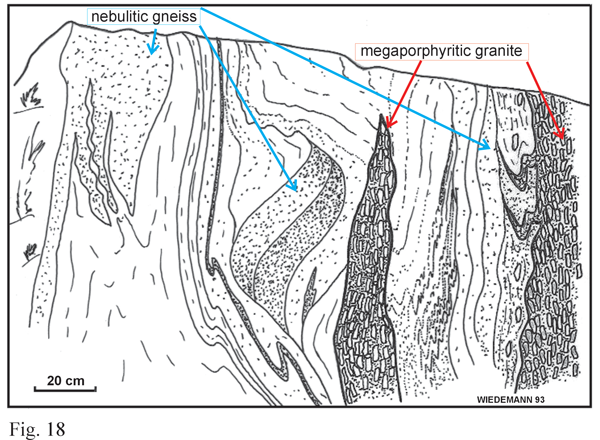
Schematic drawing of the contact between the Santa Angélica Intrusive Complex and the enclosing gneisses. The intensity of migmatization is stronger close to the pluton contact, where the enclosing gneisses exhibit nebulitic fabrics consistent with partial melting. Redrawn after Schmidt-Thomé (1987).
This intrusion has a general inverse concentric zonation, with more acidic rocks (Type I granite) at the margins, varying to a twin core of more basic rocks. Type II granite is fine-grained and intruded type I granite through brittle fractures (Figure 19). Magma mingling between monzogabbros and granites can be observed throughout the intrusion (Schmidt-Thomé & Weber-Diefenbach, 1987; Bayer et al., 1987) forming large mingled zones of enclave swarms in different degrees of hybridization with the granite (Figure 20 and 21). A main NE-SW shear zone caused more intensive mingling, producing a fine-banded rock consisting of gabbrodioritic and granitic layers (Figure 22). This NE-SW shear zone separates the two gabbroic nucleii. The foliation inside the intrusive complex trends NE and is normally sub-vertical and, at map scale, the whole magmatic body appears boudinaged.
Figure 19. Type-I granite intruded by type-II granite at the northwest border of the SAIC
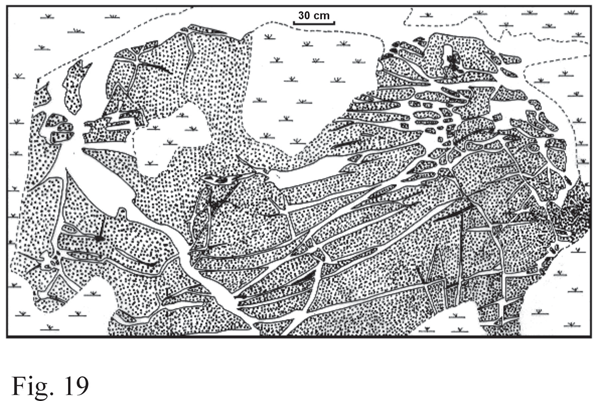
Type-I granite intruded by type-II granite at the northwest border of the SAIC. Drawing after photography.
Figure 20. Large mingled zones
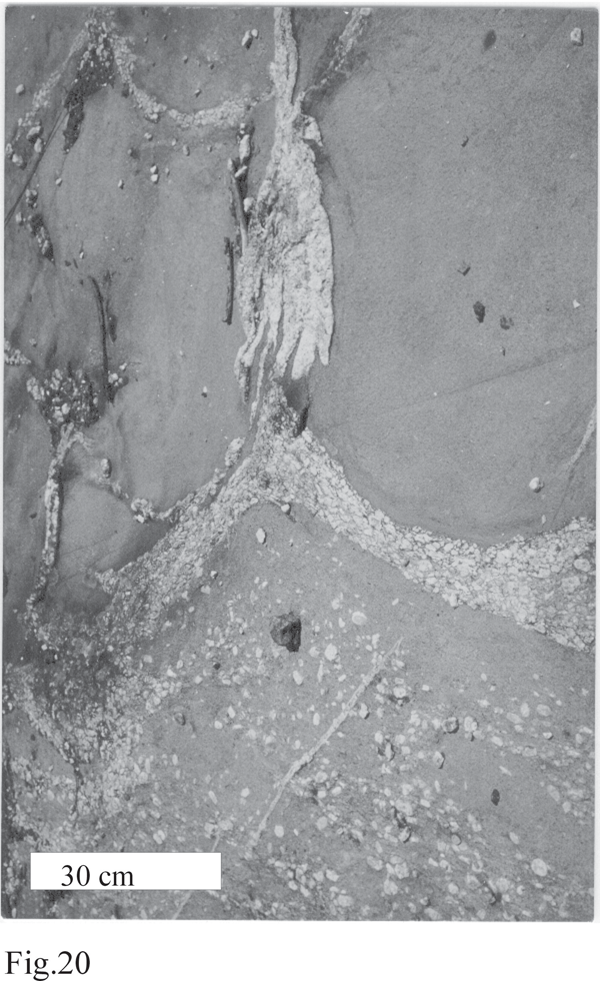
Large mingled zones formed by enclave swarms in different degrees of hybridization with the granite (SAIC).
Figure 22. chematic representation of a hybrid zone in SAIC
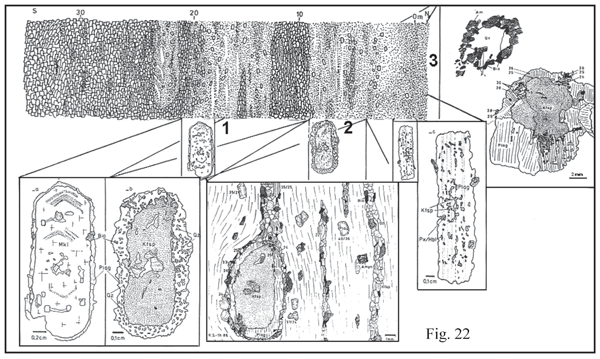
Schematic representation of a hybrid zone in SAIC (Lambari Frio region). Fine-banded rock, consisting of gabbrodioritic and granitic layers, cropping out along this main NE-SW shear zone. Schlieren of coarse-grained allanite-granite-I interfingered with dioritic magma. In the hybrid zone of granodioritic composition, three main types of mantled feldspars are found: 1) soft corroded with microcline cores and oligoclase borders; 2) orthoclase cores and plagioclase borders. Small numbers are anorthite-contents of border and matrix plagioclase; 3) corroded plagioclase from the dioritic layers. Associated with this third type there are quartz xenocrysts mantled with mafic minerals, such us amphibole and biotite. Feldspar aggregates are also common in this zone. Schematic drawing after Schmidt-Thomé (1987).
Structural studies along the contact with country rocks [Bayer, 1987; Wiedemann et al., 1997] point towards a possible ascent as a diapir, partly controlled by shear zones [Weinberg et al., 2004]. A possible explanation for the lack of a marginal syncline related to downward movement of country rocks responding to an upward rising of a diapir, could be the contemporaneous migmatization of a previously isoclinally folded crust during magma ascent. However, local small scale synclines have been observed along the contacts as shown on Figure 18.
The Castelo Complex (Figure 23) is an elliptical intrusion ~ 100 km2 with a dioritic center and a granitic border. The outermost plutonic unit is over 2 km wide and consists of interlayering between two granite types: a megaporphyritic monzogranite and a fine-grained monzogranite showing well-defined foliation. The fine-grained granite is also porphyritic and sphene-bearing. It is very similar to the type-II granite from Santa Angélica. Micaceous schlieren and ghost structures, from the partially melted or assimilated country gneisses, are frequently observed in this granite. Intrusive contatcts with the border gneiss are lit par lit sills or discordant, as dykes of the fine-grained granite intrude ductile shear zones (Figure 23). The contacts usually show evidence of a stoping mechanism and in all produces an agmatic structure generally with sharp discordant contacts with the enclosing rocks. However, at the regional scale the foliation in the gneissic country rock is broadly concordant with the pluton shape.
Figure 23. Geological map of Castelo Complex
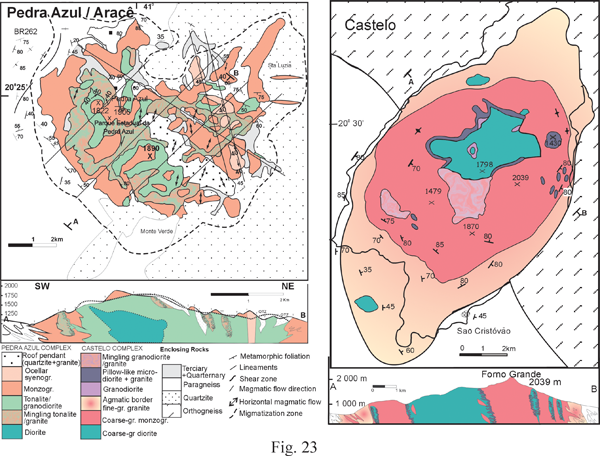
Geological map of Castelo Complex (by Weinberg, Ludka, Mendes, Horn and Wiedemann. Pedra Azul Complex (by Costa Nascimento, Costa-de-Moura and Wiedemann). References in text.
The core of the intrusion consists of a coarse-grained diorite. From the border towards the core there is a decrease in intensity of magmatic foliation. Joints filled with granitic veins is typical of the core region and suggests fracturing and dyking of an early consolidated dioritic body, possibly due to shrinkage during solidification (Wiedemann et al., 1997). The contact between granite and diorite is a narrow zone, ~ 100 m wide, formed by microgranular enclave swarms mixed to different degrees with granite (Figure 24). In this zone quartz xenocrysts with hornblende and/or biotite rims (quartz occelli), such as those found in Santa Angélica (Figure 25), feldspars showing antirapakivi- and rapakivi-type mantling are also common. In contrast to the Santa Angelica Complex, mingling in Castelo was between granite and diorite instead of gabbro. Another typical attribute for the hybrid zone in Castelo are spotted textures (Hibbard, 1995) and the presence of granitic schlieren in the microdiorite.
Figure 24. In the Castelo Complex
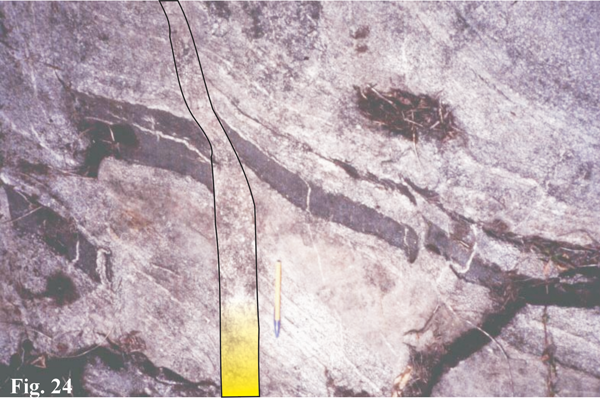
In the Castelo Complex, type-II, 10cm-wide, granite dyke (obliquely N-S in the figure) intrudes the border gneiss, along a ductile shear zone seen displacing and shearing a mafic band in the gneiss (obliquely E-W). Granite dyke is highlighted with yellow and black contour.
Figure 25. Contact between granite and diorite is a narrow zone
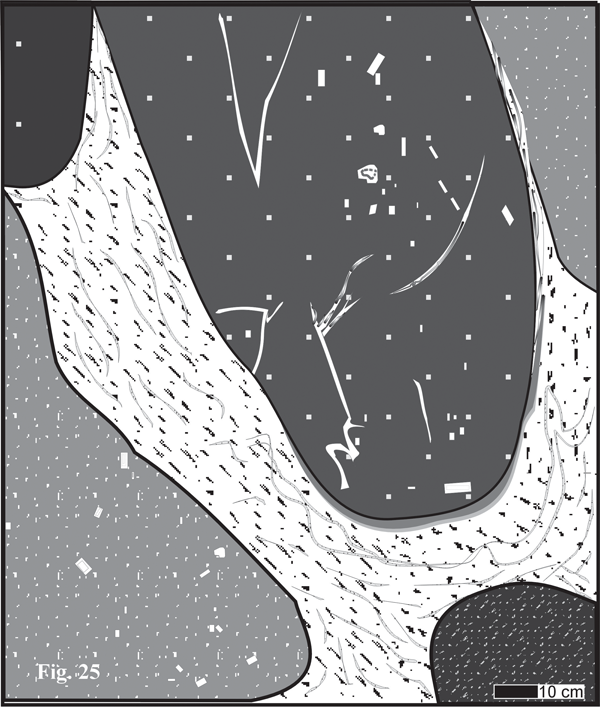
In the Castelo Complex, the contact between granite and diorite is a narrow zone ~ 100m wide, consisting of microgranular enclave swarms in different mingling degrees with the granite. Different grey patterns represent mafic pillows of different compositions, resulting from different mixing degrees with granite. Notice mantled feldspar phenocrysts in the mafic pillows. The white lines in the pillow on the upper side of the figure represent local granitic melt segregations, while the gray lines in the granitic matrix indicate the main flow directions. Drawing after a photograph.
Compared to the Santa Angelica Complex, the Castelo Complex shows less plastic strain, which, together with the evidence for stoping along the margins, might indicate different emplacement regimes or different intrusion levels.
The Pedra Azul Complex (PAC, Costa-de-Moura et al., 1999) is an irregular intrusion covering close to 200 km2. It is formed by mingling of contrasting magmas, grading from diorite to a medium-grained syenogranite (Figure 23). A general NW-SE and NE-SW fracturing is observed along the whole pluton and its sillimanite quartzites and aluminous gneisses wall rocks. The igneous lithotypes are separated from the enclosing rocks by a migmatized zone, which shows stoping, lit-par-lit intrusion or nebulitic contacts, with widespread partial melting of the metamorphic units. Megaporphyritic or coarse-grained facies, such as those found in most other plutons are absent in this intrusion. A medium-grained monzogranite forms the highest peaks in the center and lower border regions, constituting the outermost magmatic envelope of the structure [Wiedemann et al., 2002]. Domains of tonalitic to granodioritic compositions crop out in the center of the pluton. The contact between the monzogranite and the tonalitic to granodioritic domains is marked by mingled zones, where granitic schlieren, in contact with more mafic- and finer-grained rocks, originate pillow-like and net-veined structures. A small region of dioritic composition is exposed over 6 km2, at the northern border, close to the city of Aracê. The igneous flow in the PAC is marked by magmatic lineation and by the alignment of enclaves as indicated in the map in Figure 23 (Costa-de-Moura et al., 1999).
Abundant host rocks slabs and smaller xenoliths of sillimanite quartzite and garnet-sillimanite-biotite gneiss define an agmatitic mixture of granitic veins and xenoliths, cropping out over some 15 km, in a 1 km-wide zone in the interior of the pluton. This zone follows a SE-NW lineament (fracture or fault zone), crosses almost the entire pluton and is interpreted as an assimilated roof pendant (see "Roof pendant" in Figure 23), from the uppermost part of the structure. Several bands of country rocks swarms in the granite domain have been described mostly parallel to the NW border.
The last intrusive event in the PAC was the emplacement of several stocks of ocellar syenogranite, elongated along a NW-SE fracture zone. The ocellar texture of this late syenogranite is a peculiar one and is formed by hololeucocratic (quartz and K-feldspar), titanite-rich spots, wrapped by a biotite-magnetite-ilmenite-rich mesocratic matrix, in which allanite and titanite are present.
The magnitude of the magnetic anomaly (Tuller, 1993), corresponding to the intrusion of Pedra Azul, is comparable to that of Santa Angélica. However, the small amount of Outcropping basic rocks, together with the presence of a large sillimanite-quartzite roof pendant, indicate shallower erosion and/or lower intrusion levels at Pedra Azul. Compared to the other intrusive complexes, Pedra Azul has the largest volume of hybrid rocks.
The Mimoso do Sul Complex consists of two separate intrusions: a predominantly monzonitic unit (Torre Pluton) and a gabbroic unit (Jacutinga Pluton) (Figure 26). The Torre Pluton has three concentric layers: 1) a center of dioritic /monzodioritic composition; 2) a ring of mesoperthitic monzonite; and 3) an outer ring of microcline-quartz-monzonite and granite. The contact between the pluton and the enclosing rock is sharp. Inside the pluton, the gradual change from microcline quartzmonzonite towards the dioritic/monzodioritic center could only be traced by detailed petrographic mapping (Ludka, 1991). In the Torre Pluton the magmatic foliation is steep to sub-vertical. Associated sills or dykes of peralkaline apatite-biotite-Fe-augite pyroxenites crosscut the outer border and the center of the intrusion.
Figure 26. Geological map of the Mimoso do Sul Complex
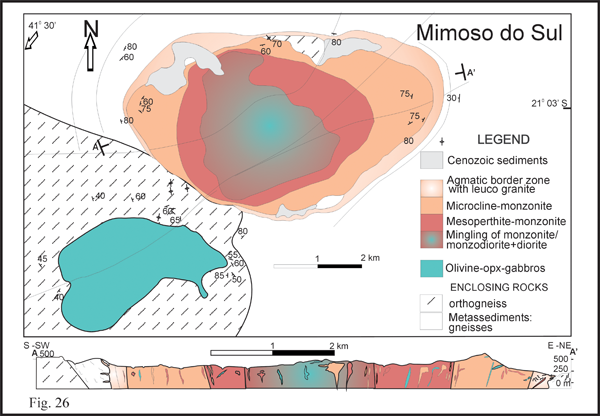
Geological map of the Mimoso do Sul Complex, by Ludka, and Wiedemann. References in text.
The gabbroic body of the Jacutinga Pluton consists of gabbro-noritic rocks with compositions grading from fine-grained olivine-orthopyroxene(opx)-clinopyroxene(cpx) melagabbros up to coarse-grained opx-cpx leucogabbros. Fine igneous layering (Figure 27) is characteristic for most Jacutinga Outcrops. Coronitic textures, consisting of complex olivine-(and/or pyroxene)-plagioclase overgrowths or intergrowths, are also common (Ludka, 1991; Wiedemann et al., 1995; Ludka, 1997).
Figure 27. Fine rhythmic layering in the monzodiorite from the Torre Pluton
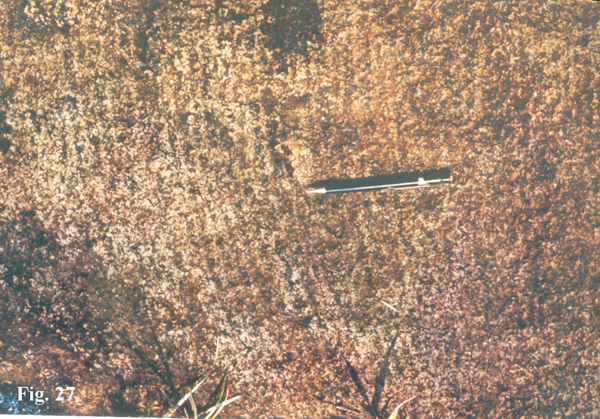
Fine rhythmic layering in the monzodiorite from the Torre Pluton, consisting of primary mesoperthite+plagioclase+clinopyroxene+biotite+apatite. This feature is also characteristic for most Jacutinga Pluton Outcrops, in Mimoso do Sul.
Three distinct magmatic suites have been recognized in the G5 group (Figure 10b and 11b; Wiedemann et al, 1986; Horn & Weber-Diefenbach, 1987; Offman, 1990; Ludka, 1991; Wiedemann, 1993; Mendes et al, 1997; Medeiros et al., 2001; Wiedemann et al., 2002): a) tholeiitic (Jacutinga and Itaoca), b) high-K calc-alkaline (Santa Angélica, Castelo, Iconha, Pedra Azul, Várzea Alegre), and c) a very high-K alkaline (Torre/Mimoso do Sul, Venda Nova and Conceição de Muqui). The use of shoshonite as a classification for the very high-K suite has been avoided because this term has been proposed for a volcanic rock, a trachyandesite (Iddings, 1982). The granitoids focused here are usually coarse-grained and very rich in feldspar. In this case very high-K contents could be the result of feldspar accumulation processes. Examples of whole-rock geochemical analyses of the G5 group are presented in Tables 2 and Table 3. The high-K calc-alkaline rocks (suite b) above) are the most significant group (almost 90% of all plutons, by area and volume) while suites a) and c) are restricted (Fig. 2).
G5 high-K calc-alkaline granitoids plot in the R1R2-diagram (Fig. 9c,d; Batchelor & Bowden, 1985) from field 2 (pre-plate collision) and field 6 (syn-collision and anatetic melts) towards field 3 (post-collision uplift). Very high-K granitoids (suite c) plot mainly in the late-orogenic field 4. Comparision between G1, G2, G3 and G5 (Fig. 9a,b), suggests a gradational change in composition with time, typical for younger orogenic belts (Bonin, 2004). While G5 granitoids plot mostly in fields 3 and 4 for late orogenic plutons, with less evolved compositions in field 2, for pre-plate collision magmas, the older G1 and G2 - G3 suites tend to concentrate in fields 2 and 1+2, respectively.
Most granitoids are abnormally enriched in incompatible elements, mainly LILE, such as K, Ba, Sr and LREE (Figure 12b and 28). High contents of HFSE, Ti, Y, Nb, P and Zr (Horn & Weber-Diefenbach, 1987; Tables 2 and 3) are also characteristic. REE diagrams from several G5 intrusions are shown in Figure 28. Almost all units from Iconha (not described here) and Venda Nova Plutons/Complexes show similar variations in chondrite-normalized REE distributions, with steep slopes from LREE to HREE (Figure 28c). The more evolved granite II have lower LREE/HREE ratios (Figure 28c,d), commonly observed in high-silicate magmas. This may be attributed to fractionation of accessory phases (e.g. zircon, monazite, allanite - DePaolo, 1988). Regarding the least evolved rocks, Jacutinga gabbro-norites show gentle slopes (Figure 28a) while the monzogabbros at Venda Nova and monzodiorites at Iconha (Figure 28b,c,d) have much steeper patterns. When comparing the least evolved rock associations from these plutons, it is evident that, although Jacutinga rocks show strong enrichment in incompatible elements (Figure 28a) those from Venda Nova (Figure 28b,c) are more enriched. Jacutinga gabbro-norites contain hypersthene and no alkaline minerals, not even in the CIPW-norm. Olivine is resctricted to a local occurrence. The rocks from Torre and Venda Nova bodies, on the other hand, have much higher K2O-contents for lower SiO2-values (Figs 9c,d and 11B). This results in the absence of hypersthene in most lithotypes, crystallization of Fe-salite instead, presence of leucite in the CIPW-norm and agpaitic index > 1 (Ludka, 1997). Therefore the Torre Pluton and Venda Nova Complex are considered to be examples of the onset of a late-orogenic alkaline magmatism. This is a gradual process with the coeval intrusion of peraluminous calc-alkaline granites from crustal remelting (Figure 10B), which are usually found associated with the alkaline/peralkaline lithotypes (monzonites, monzodiorites and associated pyroxenite/biotitite of Figs 9D and 26).
Figure 28. Rare Earth Element abundance normalized to chondrite
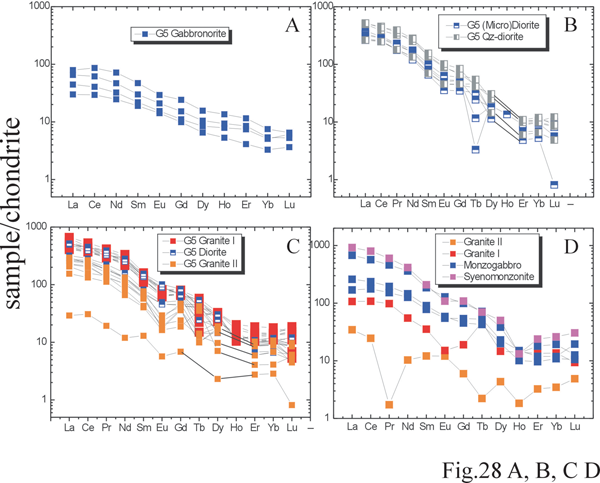
Rare Earth Element abundance normalized to chondrite (after McDonough and Sun, 1995) plotted against REE atomic numbers. A: G5 gabbronorite from Jacutinga (data from Ludka, 1997). B: G5 microdiorite and quartz-diorite from Iconha (data from Offman, 1990). C: G5 granite I, -granite II and diorite from Iconha (data from Offman, 1990); D) G5 very high-K rocks from Venda Nova (data from Offman, 1990). Discussion in text.
Migmatization of country rocks during emplacement could account for similarities between REE patterns of enclosing gneisses and G5 granites (granite II; Figure 13 and 28). However, the Nd values for Santa Angelica (Figure 14) show a discrepancy and point towards an older continental source for these granites. The dominant granite I is SiO2 oversatured. This granite shows normative corundum and typically high P, Ti, Zr, K, Ba and Sr (Figure 12b). Less enriched REE-patterns however similar to those from gabbro-dioritic lithotypes are compatible with a long lasting melt interaction between less and more evolved melts (Figure 28b,c,d). LREE contents (Figure 28c,d), Rb, Sr and Rb/Sr values are yet typical of the upper crust (Tables 1 and 3) and indicative of a hybrid origin.
Zircon ages constrain the magmatic crystallization of the late plutons to between 530 and 480 Ma (Table 1; Söllner et al., 2000) confirming their late post-collisional emplacement. Units from the Santa Angélica and Mimoso do Sul Complexes yielded the following U-Pb ages: 513±8 Ma, for the Santa Angélica megaporphyritic granite, 492±15 Ma for the Santa Angélica type-II titanite granite and 480±4 Ma for the Mimoso do Sul coarse grained syenomonzonite. Rb-Sr data from the Várzea Alegre megaporphyritic granite yield a whole-rock isochron age of 508±12 Ma (Medeiros et al., 2000). Zircons of the charnockitic rocks from Várzea Alegre yielded an U-Pb age of 499±5 Ma, implying that both charnockite and porphyritic granite crystallized simultaneously (Medeiros et al., 2003). Whole-rock Rb-Sr isotopic data from the Pedra Azul granite (Platzer, 1997) indicate a crystallization age of 536 31 Ma.
Isotopic data from metabasic rocks from the rifting stage of the orogen point towards a depleted mantle source (Sm-Nd whole-rock isochron based on five samples yielded an age of 816 ± 72 Ma, Nd(t)= +3.4 to + 4.6; Pedrosa-Soares et al., 1998). During the subduction phase of the orogen, repetitive episodes of crustal contamination of the asthenospheric mantle wedge must have occurred to contribute for the enrichment patterns of the intermediate and basic mantle-derived melts. However Nd(t) values below -20 for the Santa Angélica basic rocks (Table 1) together with extremely high Ba and Sr values (Figure 12a,b) have been considered to be due to a locally abnormal mantle enrichment (Wiedemann et al., 1995; Ludka et al., 1998).
Figure 14 shows the initial Nd and Sr isotopic compositions for magmatic and metamorphic rocks from the area, time-corrected for the mean crystallization age of the G5 suite (510 Ma). We distinguish two groups, both of which plot in the enriched mantle field. The first group is represented by gabbro, granite and charnockite from Várzea Alegre, by 560 to 580 Ma old metaplutonic rocks of G1 and G2 suites and by the enclosing metasedimentary rocks (>613 Ma kinzingite). This group of samples is characterized by a relatively uniform Nd isotopic composition followed by extreme variations in Sr isotopes. Thereby, the metasedimentary rocks represent the most enriched member. We tentatively interpret this systematics as reflecting contamination of a mantle-derived basaltic melt, now most closely approached by the Várzea Alegre gabbro, due to assimilation of melts derived from a Neoproterozoic crust. In such a model, a hyperbolic mixing curve would start in a more depleted mantle field.
A second group is represented by only two magmatic samples from Santa Angélica, with almost identical Sr-Nd isotopes. U-Pb dating of zircons from these samples indicate zircon cores older than 2000 Ma, possibly Archean (Söllner et al., 1991). Accordingly, the Nd isotopes indicate assimilation of a much older crust than in the case of Várzea Alegre or melt generation from an anomalous enriched mantle source (Horn & Weber-Diefenbach, 1987; Offman, 1990; Ludka, 1997). Taking into account the reduced number of measured samples, this comparison demonstrates that different plutons may have different magmatic sources. We speculate that the granitic melt from Santa Angélica could have been originated from the melting of an Archean crustal segment. This granitic melt mixed with a mantle derived basaltic melt to produce a hybrid monzogabbro with the same isotopic signature. The smaller spreading of data points between granite and monzogabbro samples, in the Santa Angélica Intrusive Complex, in comparison to Várzea Alegre, is consistent with field and petrographic evidence of a more effective mixing between gabbro and granite in this intrusion. However, high K2O-values of most basic and intermediate rocks, and compositional gaps on geochemical trends reflect heterogeneous mingling and restricted mixing. An alternative could be that mixing with a granitic magma may have occurred at depth and high temperature, before plagioclase crystallization, followed by mingling during emplacement, as demonstrated by van Westrenen (1997), using trace element partitioning between plagioclase and less evolved melts from the Pedra Azul Complex.
Magma mingling/mixing, fractional crystallization and assimilation of country rocks are the main differentiation processes during the evolution of these igneous sequences (Wiedemann, 1993; Horn & Weber-Diefenbach, 1989; Mendes et al., 1997, 1999; Platzer, 1995; Mendes et al., 1997; Ludka et al., 1998; Pedrosa-Soares et al., 2001; Medeiros et al., 2000, 2001; Wiedemann et al., 2002). The late post-collisional magmatism is a product of a bimodal system consisting of mantelic and crustal-derived magmas, which are thought to be the end-members of the initial bimodal system. The ascent of mantelic magmas, probably along ductile shear zones, increasing the geothermal gradient, seems to have induced crustal melting. Physical and chemical interaction between these melts at source, separate geochemical evolution in a later stage and restricted hybridization during emplacement could explain the formation of the differently complex zoned plutons. The differences in exposed rock types and in the intensity of magma mingling/mixing processes in the area have been considered to be due to different sources, emplacement conditions and erosional levels (Wiedemann et al., 2002).
The observations summarized here confirm the classical model of generation of granitic and intermediate magmas, induced by underplating of basaltic magma under the continental crust during late orogenic stages (Batchelor & Bowden, 1985; Hall, 1987; Huppert & Sparks, 1988; Bonin, 2004; Perugini et al., 2004).