Textural and Metamorphic Evolution of the Frido Unit Metadolerite Dikes
Metadolerite dikes crosscutting serpentinites in the Frido Unit ophiolites are characterized by a wide range of textures going from well-preserved igneous intersertal/integranular and blastophitic textures to cataclastic and mylonitic. The microstructural and metamorphic evolution of the investigated metadolerites is summarized in Table 2. In the least deformed metadolerite samples showing intersertal/intergranular and blastophitic texture, plagioclase, clinopyroxene and opaque minerals represent the relict igneous phases (“Igneous” stage in Table 2). Clinopyroxene is replaced by brown amphibole (mainly Mg-hornblende and Mg-hastingsite) rimmed by green amphibole with similar compositions. The brown and green (or blue-green) amphiboles are often rimmed by uncoloured actinolite. We interpret these Ca-amphiboles as being related to oceanic metamorphism in the Jurassic Tethys Ocean that occurred under amphibolite to greenschists facies conditions (e.g. Liou et al., 1974; Spear, 1981; stage “Oceanic 1” in Table 2). Brown and red/brown amphiboles have been described in oceanic gabbros (e.g. Prichard and Cann, 1982; Mével, 1988; Coogan et al., 2001) and ophiolitic gabbros (e.g. Mével et al., 1978; Tribuzio et al., 1995, 1999) and attributed to either magmatic or late magmatic crystallization (e.g. Tribuzio et al., 2000) or to HT-oceanic alteration (e.g. Gaggero and Cortesogno, 1997) or both (e.g. Coogan et al., 2001), depending on textural features and composition of trace elements. In the Northern Apennine ophiolite, brown amphibole associated with green and blue-green amphiboles has been found in dolerites and attributed to oceanic metamorphism (Cortesogno and Oliveri, 1974). Green and pale-green Ca-amphiboles replacing clinopyroxene and veins filled with actinolite, actinolitic hornblende, and Mg-hornblende have been found in dolerites drilled in the modern oceanic crust from the Pacific ocean (lower sheeted dike complex; Laverne et al., 1995; Tartarotti et al., 1995; Alt et al., 1996; Vanko et al., 1996) indicating relatively high temperatures (>400°C), typical of greenschists to amphibolite facies conditions.
Plagioclase phenocrysts in the Frido metadolerites are replaced by pseudomorphs of sericite or saussurite, pumpellyite, prehnite, chlorite, and epidote. These metamorphic minerals can be interpreted either as oceanic (stage “Oceanic 2” in Table 2) or as orogenic (see Table 2), since minerals of the prehnite-pumpellyite facies as well as of the greenschists (or sub-greenschists) facies have been described in both the present-day oceanic crust (e.g. Alt et al., 1986, 1996, 2010 and refs. therein) and in orogenic environments (e.g. in the Apennines; Cortesogno and Oliveri, 1974; Cortesogno, 1980; Beccaluva et al., 1982). As far as pumpellyite, its textural features and the high Al2O3 content suggest that it probably crystallized under blueschists or lawsonite-albite facies conditions (Cortesogno et al., 1984, Sansone and Rizzo, 2012), supporting the hypothesis of an orogenic (subduction-related) origin.
Blue amphibole (glaucophane and Mg-riebekite), that commonly grows at the rim of Ca-amphiboles replacing clinopyroxene in the Frido metadolerites, is here interpreted as being of orogenic origin (stage “Subduction-related” in Table 2): it crystallized during subduction under HP-LT conditions in the Apennine accretionary wedge, as already discussed in Spadea (1994) and Sansone et al. (2011). Ca-Na amphibole (winchite) at the contact between green and blue amphiboles might be interpreted as a reaction product formed during the initial stage of the subduction phase (“Win” in Table 2). In fact, winchite has been described in eclogites from the Western Alps (Gran Paradiso and Sesia-Lanzo unit; Ungaretti et al., 1983; Benciolini et al., 1994). Lawsonite in our samples commonly fills veins. It is also interpreted as being a subduction-related mineral (Table 2). White mica filling veins has a phengitic composition and can be also ascribed to a subduction-related stage (Bousquet et al., 2002).
In textural domains where the suite “brown- green- and blue-amphibole” occurs, we also observed the association of chlorite+aegirin-augite (see Figs. 7a, 7b). These two minerals are interpreted as being crystallized under blueschist facies (or epidote-blueschist facies as defined by Evans, 1990) conditions. Aegirin-augite has been observed in blueschists facies rocks from the North Cascades and from New Zealand (e.g. Brown, 1974). However, secondary aegirin-augite of oceanic origin has been also described in basalts from the Pacific Ocean (Laverne, 1987); consequently, an oceanic origin for this pyroxene cannot be completely ruled out.
Additional metamorphic minerals found in the studied samples also include quartz, albite, apatite, titanite, and minor calcite and chrysotile (see also Sansone et al., 2011). These minerals occur either as pseudomorphs (or replacive) crystals after igneous phases or more often as vein infilling. Mineral associations within veins may be various; in fact, within a vein, mineral assemblages typical of the prehnite-pumpellyite as well as of the lawsonite-glaucophane facies may occur together, as already stated by Sansone et al. (2011) and Sansone and Rizzo (2012). However, since veins cut through all the previous textures of the rock, they are interpreted as being formed during the entire orogenic evolution of the Frido ophiolite within the Apennine accretionary wedge, from subduction to late orogenic stages (see Table 2). Similar metamorphic mineral assemblages have been described in the Franciscan subduction complex (California), where mafic blocks in the mélanges are mostly composed of LT-blueschist facies minerals including albite, chlorite, pumpellyite, lawsonite, Na-pyroxene, quartz, sphene (and no garnet; e.g. Cloos, 1985 and refs. therein). This is different from most of the lawsonite-bearing rocks occurring at active margins, being lawsonite eclogites (e.g. Tsujimori et al., 2006; Zucali & Spalla, 2011).
Summing up, we may infer that the studied metadolerites have been affected by rock-seawater (or seawater-derived fluids) interaction in the Jurassic Tethys Ocean, producing oceanic alteration under amphibolite to greenschists and facies conditions. Oceanic hydrothermal alteration may have induced element mobility (e.g. LREE), although the studied rocks have all an N-MORB affinity (Sansone et al., 2011). These oceanic rocks have been subsequently involved in the Apennine accretionary prism, as attested by the occurrence of blueschist facies minerals and by late prehnite-pumpellyite facies mineral assemblages (see Table 2). A qualitative P-T path for the investigated rocks is illustrated in Figure 8. The oceanic evolution of the metadolerite dikes is constrained by the crystallization of amphiboles under amphibolite and greenschist facies conditions (steps 1 and 2 in Fig. 8) at low pressure. The early orogenic evolution is marked by crystallization of (LT-) blueschist facies minerals, namely lawsonite and glaucophane related to subduction (“subduction-related” stage in Table 2). Pressure and temperature are difficult to determine however, as the glaucophane stability field (“Gln in” curve in Fig. 8) constrains the upper T boundary (Maresch, 1977), while the occurrence of lawsonite in the studied samples suggests minimum pressures of 3 kbar (Thompson, 1971; Liou, 1971). Lawsonite in the metadolerite dikes occurs inside veins and is mostly associated with LT-blueschist facies minerals. Consequently, the reaction curve Lws+Gln = Pmp+Chl+Ab+Qtz+fluid by Liou et al. (1985) was chosen to constrain the upper pressure boundary for the studied samples (curve 2 in Fig. 8). The presence of albite instead of jadeite and quartz (curve 1 in Fig. 8) suggests a maximum pressure for the subduction-related metamorphism of 8 to 10 kbar in the temperature range of 100 to 250°C (Newton & Smith, 1967). Late orogenic minerals observed in our samples (“late orogenic stage” in Table 2) suggest a decompressional retrograde path toward the prehnite-pumpellyite facies (stage 4 in Fig. 8).
Figure 8. P-T diagram showing the metamorphic evolution of the Frido Unit metadolerites.
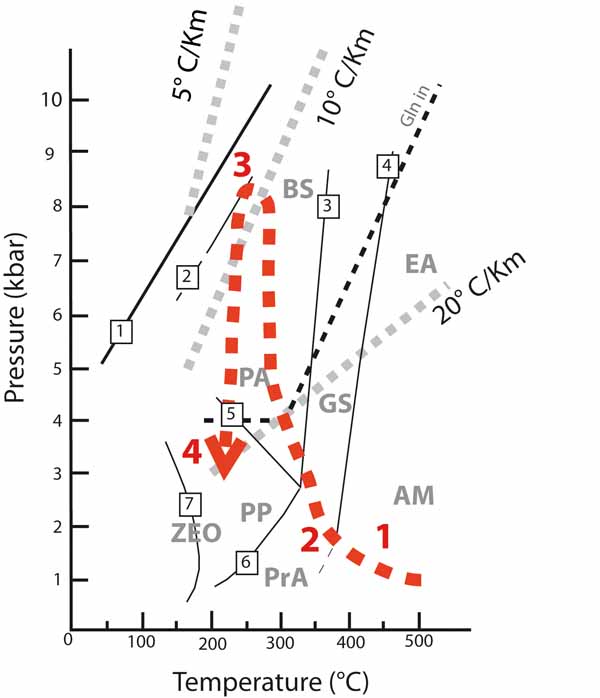
Metamorphic evolution of the Frido unit metadolerites plot in a P-T diagram showing petrogenetic grid for low-grade metamorphic facies in a basaltic system (redrawn after Liou et al., 1985 and after Maresch, 1977 for the glaucophane stability field). A proposed qualitative P-T path is illustrated (red arrowed-trajectory). Red numbers (1 through 4) refers to Oceanic 1, Oceanic 2, Subduction-related, and Late orogenic stages, as defined in Table 2, respectively (see text and Table 2 for comments). Numbered lines are the following univariant lines (according to Liou et al., 1985): (1) Jd+Qtz = Ab; (2) Lws+Gln = Pmp+Chl+Ab+Qtz+fluid; (3) Pmp+Chl+Qtz = Zo+Tr+fluid; (4) Zo+Chl+Tr+Qtz = Hbl+fluid; (5) Pmp+Tr+Qtz = Prh+Chl+fluid, (6) Pmp+Qtz = Zo+Prh+Chl+fluid; (7) Ab+fluid = Anl+Qtz. “Gln in”: upper stability field for glaucophane according to Maresch (1977). Metamorphic facies: PP=prehnite-pumpellyite; PrA=prehnite-actinolite; GS=greenschist; BS=blueschist; EA=epidote-amphibolite; AM=amphibolite. Geothermal gradients of 5°C/Km, 10°C/Km, and 20°C/Km are also shown. Mineral abbreviations according to Siivola and Schmid (2007).
The retrograde path did not necessarily take place at a lower temperature than the prograde one during the subduction; thus the downward and upward paths could have been almost identical. However, we suggest that the retrograde path did not proceed under a higher temperature than the prograde path, since relatively high temperatures would have led to the lawsonite breakdown. Consequently, the inferred qualitative P-T-path follows a counter-clockwise trajectory. Counter-clockwise trajectories for subducted terranes are commonly attributed by numerical modeling to early subduction stages in an accretionary prism (e.g. Cloos, 1982; Gerya et al., 2002; Gerya, 2011), such as the Franciscan Complex (Cloos, 1985). Well-developed counter-clockwise paths have been proposed for fast subduction by Roda et al. (2010), where burial flow dominates over the upwelling flow. Additional field and analytical studies are needed to better constrain and quantify the metamorphic evolution of the Frido unit.