The cornerstone: inclusions in enclaves and xenoliths
The use of MI for a better understanding of crustal melting has built primarily from the study of crustal enclaves hosted in the felsic peraluminous lavas of the Neogene Volcanic Province of SE Spain, and in particular from those hosted by the dacite of El Hoyazo, near Almería.
Figure 4. Andalusite with MI from a crustal xenolith in the Crd-bearing rhyolites of Lipari
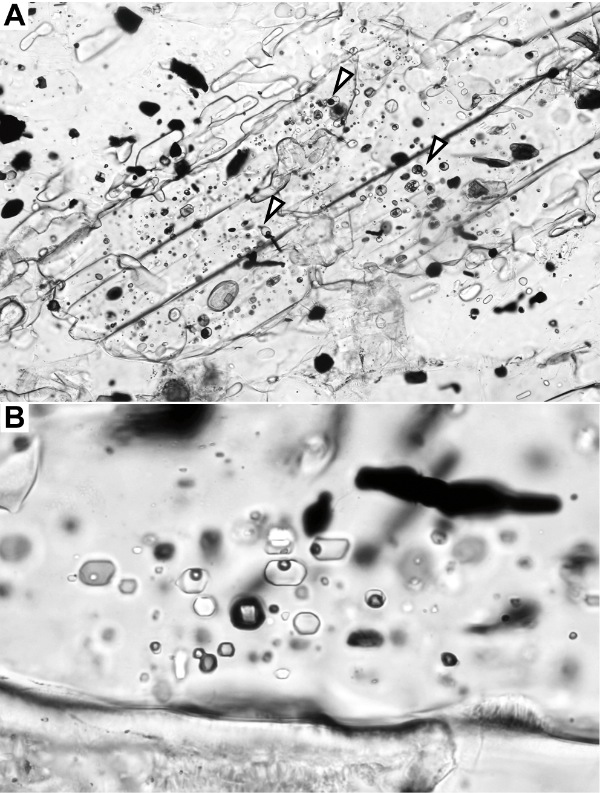
A: large scale view with arrows pointing at MI-rich zones. Width of view: 3.4 mm. B: close-up with detailed view of the glassy inclusions, each containing a shrinkage bubble. Width of view: 0.2 mm.
In this exceptional geological context, fragments of a partially melted crust situated at c. 20 km depth were rapidly brought to the Earth’s surface by magma uprise, and quenched from temperatures of c. 800°C during submarine eruption of the host volcanic rocks. This allowed the preservation of the mineralogical and textural features of an anatectic crystalline basement (Cesare et al., 1997) similar to experimental charges but in hand sample sizes. Along with microstructures from the Spanish rocks, extensively described in the literature (Acosta Vigil et al., 2010; Cesare, 2008 and references therein), here we also present new data from much smaller and less abundant crustal enclaves present in cordierite-bearing rhyolitic lavas from Lipari (S Italy, Barker, 1987), which display comparable features to those from SE Spain.
In the rocks from El Hoyazo, MI have been studied most extensively within garnet and plagioclase (e.g., Acosta Vigil et al., 2007, 2010), but they are also hosted by biotite, cordierite, hercynitic spinel, K-feldspar, quartz, ilmenite, zircon, monazite, apatite and corundum (Cesare, 2008).
In the lower-pressure enclaves from Mazarrón (SE Spain) and from Lipari MI are also hosted in andalusite (Figure 4), and such occurrence has provided important constraints for the P-T location of the Al2SiO5 triple point (Cesare et al., 2003a) as well as evidence that andalusite can coexist with a granitic melt (Clarke et al., 2005). The arrangement of MI in minerals from the studied samples is commonly indicative of a primary trapping: zonal arrangement dominates in garnet and spinel (Figure 5A), where inclusions are commonly located in the core of crystals. In zircon, inclusions are distributed in a very thin annulus separating a detrital core from the syn-anatectic overgrowth (Cesare et al., 2003b, 2009). In other hosts, especially plagioclase, MI occur throughout the mineral (Figure 5B, C) and may be so abundant to impart to the crystal a cloudy appearance. The occurrence of secondary MI, clearly associated with fractures postdating the host growth, is very rare (Cesare et al., 2007).
Figure 5. Microstructures of MI in Spanish enclaves
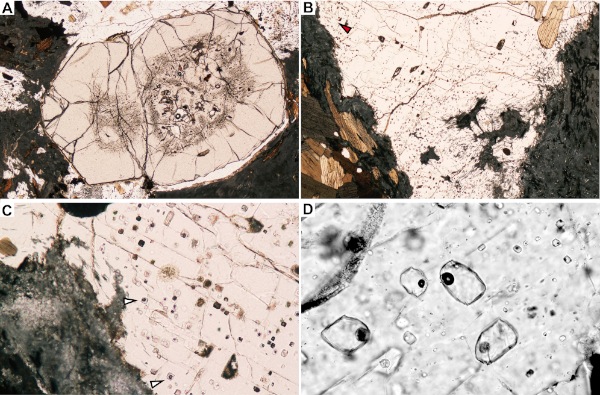
A: Zonal arrangement of MI in garnet from a residual enclave in the dacitic lavas of El Hoyazo, Spain. B: plagioclase containing MI throughout the crystal (El Hoyazo, Spain). Arrow indicates the area at the margin of plagioclase enlarged in (C). C: close-up of (B), showing that MI (negative crystals with shrinkage bubbles, arrows) are abundant also at the border of plagioclase. D: Large MI, with colorless, fresh glass and a shrinkage bubble, in plagioclase from an enclave (Mazarron, Spain). Widths of view: 8.4, 3.4, 0.85 and 0.35mm, respectively.
Figure 6. SEM microstructures of MI in spanish xenoliths
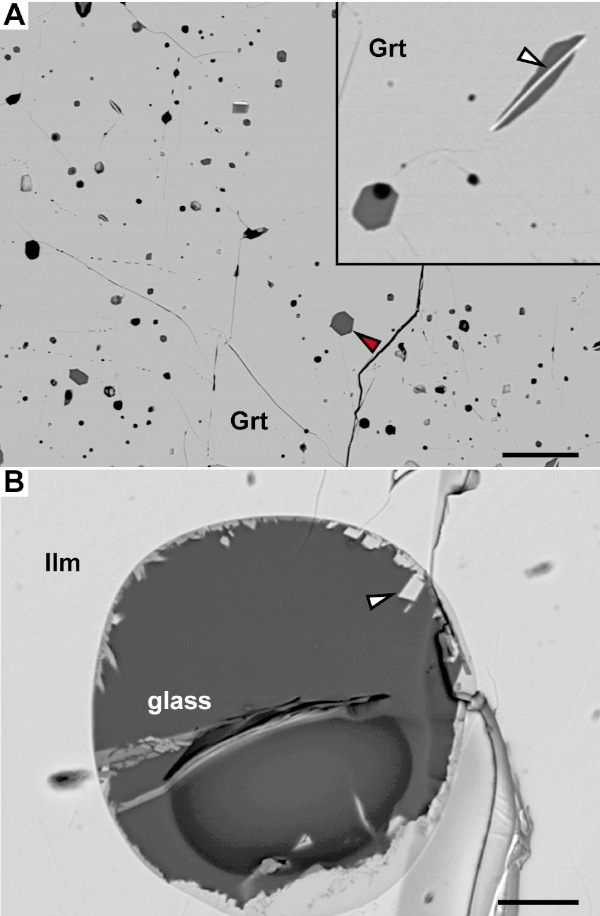
A: MI in garnet from an enclave of El Hoyazo. In the close-up of top-right two MI are enlarged: one with a well developed negative crystal shape, the other with an ilmenite needle (arrow) that is probably a trapped mineral. Scalebar: 50 µm. B: a large MI hosted in ilmenite in an enclave from El Hoyazo. The inclusion walls are coated by ilmenite daughter crystallites (arrow). Scalebar: 20 µm
The average size of MI is <30 µm, but exceptionally large inclusions may reach up to ˜100 µm in ilmenite, cordierite or plagioclase (Figures 5D and 6B). The shape is rarely irregular, more often rounded (zircon, ilmenite, cordierite) to negative crystal (garnet, spinel, plagioclase), or tubular (apatite), depending on the crystallographic control of the host mineral. The abundance of negative crystal shapes (Figure 6A) and the occurrence of "necking-down" phenomena (Acosta-Vigil et al., 2007) indicates that solution/precipitation processes were efficient in modifying the shape of inclusions in order to diminish the surface free energy (Roedder, 1984).
The inclusions very often contain fresh, colorless, isotropic and undevitrified glass, and a single shrinkage bubble (Figures 4-6) that may be empty or contain fluids exsolved from the melt (mostly H2O, Cesare et al., 2007). Owing to the very fast cooling during submarine eruption, crystallization of “daughter minerals” from the glass is very rare and limited to some overgrowths or crystallites of ilmenite in MI in ilmenite (Figure 6B), or of alkali feldspar in MI in plagioclase (Acosta-Vigil et al., 2007). While daughter minerals are rare, solid inclusions (or "trapped minerals", i.e., minerals that were already present during the formation of MI) may be widespread: the most common solid inclusion is graphite, particularly abundant in MI in plagioclase from the enclaves of El Hoyazo (Cesare and Maineri, 1999). Ilmenite (Figure 6A) and biotite are also observed as trapped minerals within MI in garnet. An origin as solid inclusions is attested by: (i) these minerals being partly enclosed in the host plagioclase or garnet; (ii) the fact that they occur only in part of the entire population of MI and in a non-systematic manner; (iii) the variable mineral/MI volume ratios; and (iv) the presence of these minerals also as single inclusions in the hosts. These observations support the hypothesis that, as frequently observed in previous studies of fluid and silicate melt inclusions, graphite, ilmenite or biotite lamellae acted as an imperfection at the growing crystal faces of the host mineral, and favoured the entrapment of MI.
The MI in enclaves and xenocrysts from felsic lavas described here are interpreted as primary, and therefore as indicating the coexistence of the host minerals with melt at the time of entrapment. These conditions were attained during partial melting of the metasedimentary protoliths, and hence the MI are interpreted as tiny droplets of the crustal anatectic melts as they were being produced.
Could the MI have formed by processes other than this, such as melting of solid inclusions already trapped within the host (Vernon, 2007)? If so, they would not indicate the growth of the host in the presence of melt, as melting could have occurred later in a geologically, and chronologically, separate event. The possibility that melting of solid inclusions (or “inclusion melting”) may produce MI with seeming primary origin has been discussed by Cesare (2008) with special reference to the MI hosted in garnet at El Hoyazo.
Figure 7. Two examples of nanogranite inclusions
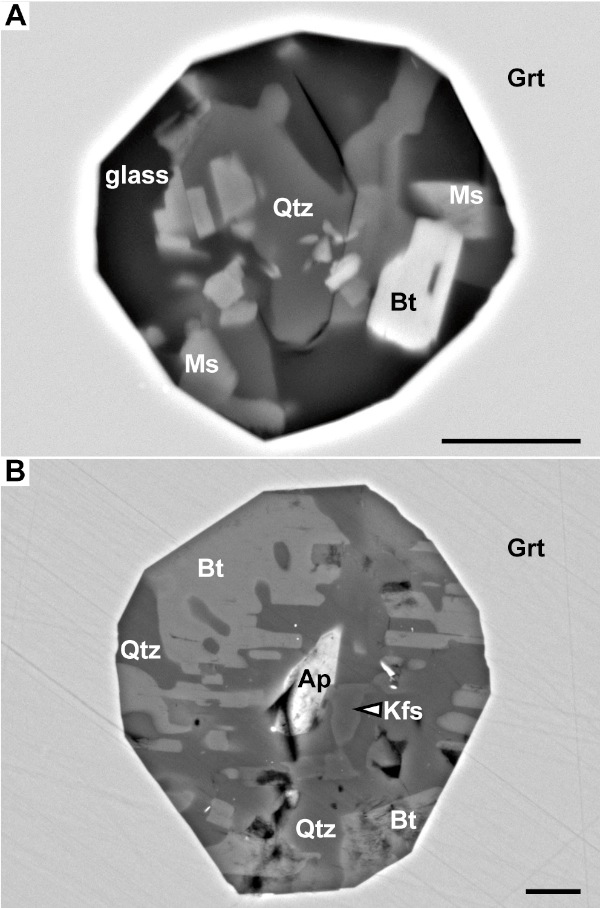
Two examples of nanogranite inclusion, hosted in garnet from the migmatites of Ronda (A) and the khondalites of the Kerala Khondalite Belt (B). Scalebars: 2 µm
It was shown that this process would be plausible only if all melt inclusions had been produced by the melting of an aggregate of solids and H2O, each of them with exactly the same proportions of reactant phases as to melt completely. Here we reiterate the conclusion that this is “…geologically unresonable…” (Cesare, 2008), but specify – based on our recent experience on inclusion remelting – that this process could work only if the inclusion trapped by the host was a nanogranite (see below). Nevertheless, the MI would have also originally contained a melt phase.
Owing to the combination of lack of crystallization and freshness of glass, these MI allow the geochemical characterization of natural crustal anatectic melts unaffected by retrograde or post-formation phenomena. Due to the exceptionally rare nature of these rocks, however, a thorough study of this genre could be undertaken for the first time only in the last decade (see below and Acosta-Vigil et al., 2010, and references therein).