Melt inclusions in minerals
Melt inclusions (MI) are small droplets of silicate liquid trapped in minerals called hosts, that either quenched as glass or crystallized to a polycrystalline aggregate upon cooling of the system. Pioneered by Sorby (1858), their microstructural study has developed under the more comprehensive framework of “fluid inclusions”, a category that encompasses all types of fluids regardless of their composition and density (Hollister & Crawford, 1981; Roedder, 1984). From a genetic viewpoint, a distinction can be made between inclusions that are trapped during (termed primary) or after (termed secondary) the crystallization of the host mineral. Much of the microstructural investigation of fluid inclusions (see criteria in Roedder, 1984, or Goldstein, 2003) is aimed at evaluating this important difference. Primary inclusions can provide much more petrological information, because the presence of a particular fluid can be linked to that of a particular mineral or assemblage. Here we essentially describe and discuss primary inclusions, where such origin has been constrained on the basis of one of the most robust textural criteria, named the “zonal arrangement” (Sobolev & Kostyuk, 1975): inclusions are distributed in the core of, or in annuli within, the host (Figure 1). When inclusions present systematically (i.e., in a comparatively large, coherent number of occurrences) a zonal arrangement, one can conclude that they most likely formed during the growth of their host. Considering MI, primary entrapment indicates the growth of a crystal in the presence of a melt phase. One of the most common processes for this to happen is the crystallization of magma, and this is the reason why MI are so important for, and so extensively studied in, igneous petrology (Clocchiatti, 1975; Frezzotti, 2001; Schiano, 2003; Webster, 2006). Melt inclusion-rich phenocrysts in lavas are typical examples of this mode of occurrence, where the host is crystallizing from the melt that it is being entrapped (Figure 2). A second major, and perhaps less recognized process by which MI can form, more relevant to crustal anatexis, is when the mineral host and melt form at the same time, with the host being able to trap the melt that it is growing with.
This circumstance takes place during incongruent melting reactions, where peritectic solid phases are
Figure 1. Microstructures indicating primary entrapment of MI
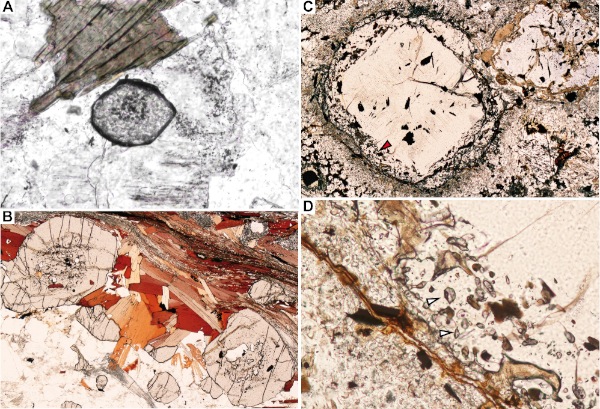
Zonal arrangement of primary MI in garnet from migmatites of Ronda (A), from the kinzigites of the Ivrea Zone (B) and from a residual enclave in the Crd-bearing rhyolites of Lipari (C and area indicated by red arrow enlarged in D, where arrows point to glassy inclusions). Widths of view: 0.35, 8.4, 3.4 and 0.42 mm, respectively.
formed together with an anatectic melt. For example, during the partial melting of metasedimentary protoliths at mid- to deep-crustal depths (0.5 - 1 GPa), the largest volumes of melt are produced by the incongruent breakdown of biotite to form peritectic garnet. Under these conditions, primary MI can be trapped in a peritectic host such as garnet (or orthopyroxene in Al2SiO5-free rocks).
There is a fundamental difference between the two processes of primary MI entrapment described above (i.e., melt crystallization vs. incongruent melting) , that can be visualized in the schematic representation of Figure 3, where melting and crystallization are considered essentially a function of temperature. Assuming the evolution of a partially melted system as a heating-cooling path, it can be recognized that most of the melt production by incongruent melting reactions occurs during the prograde up-T part, whereas most of melt consumption (by magma crystallization or by retrograde reactions) occurs during the down-T section. As a consequence, we
Figure 2. Primary MI in a plagioclase glomerocryst in a Crd-bearing rhyolite from San Vincenzo, Italy
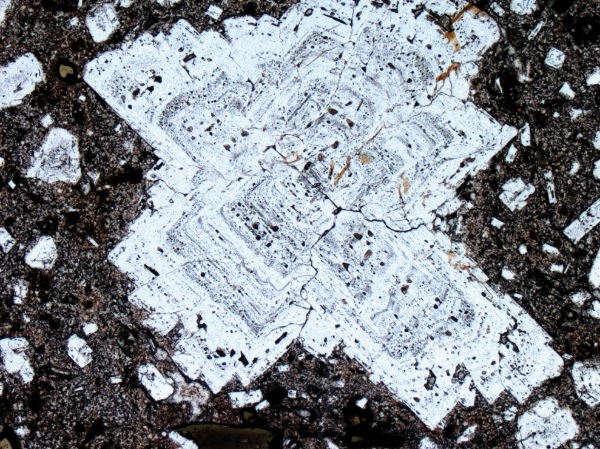
The MI, arranged in concentric zones attesting to the progressive growth of the host, contain the melt that was evolving during magma crystallization. Width of view: 3.4 mm.
expect a quasi-systematic distribution of MI associated with each of these two modes of entrapment, so that MI formed during melt/magma crystallization are generally trapped during the cooling path (1 in Figure 3) and will be hosted in minerals from leucosomes or igneous rocks, whereas MI related to the melting process are mostly formed during the heating path and will be hosted in peritectic minerals (2 in Figure 3).
Figure 3. Schematic temperature (T)-time (t) diagram
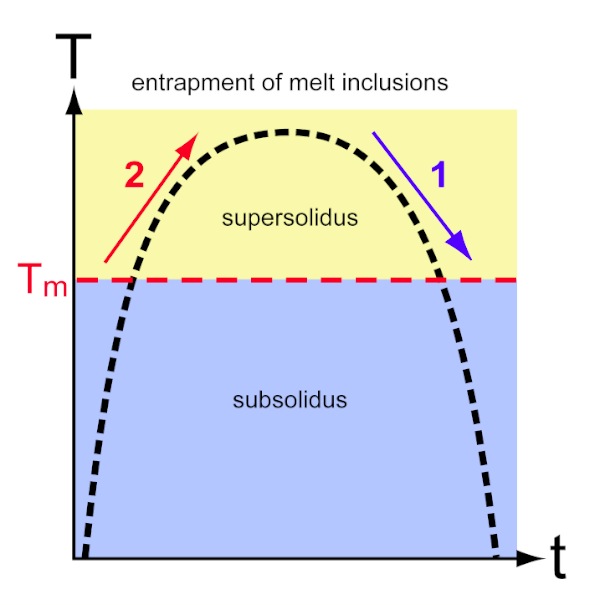
Schematic temperature (T)-time (t) diagram illustrating the two main modes of entrapment of MI during crustal anatexis and melt production (path 2), and magma crystallization (path 1). The former occurs mostly during he heating path of the rock, whereas the latter occurs dooring cooling. Only inclusions trapped along path 2 may contain primary compositions of anatectic melts.
In other words, the two modes are linked to two different parts of the evolution of an anatectic/igneous system. From a geochemical point of view this behaviour has one major consequence in that while MI in igneous rocks (including leucosomes in migmatites) contain evolved compositions, those in peritectic minerals in migmatites and granulites should display the primary compositions of anatectic melts. Although the scenario simplified in Figure 3 does not reflect the complexity of the natural processes, where pressure and composition changes may also play a major role, the consequences and the conclusions that are reached here maintain a first-order validity.