The development: nanogranites in migmatites
If anatectic melt is preserved as, and found in MI from unusual settings such as crustal enclaves in peraluminuous felsic lavas, why have MI not been found in the more representative and common rocks that are produced by anatexis, i.e., migmatites and granulites? This question prompted the recent efforts of the writers, who started to (re)investigate rock samples from migmatite terrains worldwide looking for MI in peritectic minerals, in particular within garnet, thought to be one of the most promising hosts as it is formed by the incongruent melting of biotite. Of course, given the slow cooling (some millions of years compared with some minutes or days) that regionally metamorphosed and partially melted rocks have undergone, one should not expect to find glass anymore within MI, but rather a polyphase cryptocrystalline aggregate resulting from melt crystallization, as commonly observed in plutonic rocks (Bodnar and Student, 2006). This search led to the discovery (Cesare et al., 2009) of "nanogranites" in garnets from the melanosomes of migmatitic granulites in the Kerala Khondalite Belt (KKB, India).
These are inclusions containing a granitic assemblage (quartz, feldspars and micas) made of crystals with micrometric or sub-micrometric grain-size (Figure 7). Despite the small size, the polycrystalline nature of these inclusions can easily be detected with a (good) optical microscope. For the sake of completeness, it should be noted that the inclusions in garnet previously described by Hartel et al. (1990) seem to be very similar to nanogranites, but this early report was not followed by detailed study. After the recent occurrence in the KKB, nanogranite inclusions have been also found in garnet from migmatites at Ronda (Spain), the Ivrea Zone and Ulten Zone (Italy) and the Himalayas (Nepal), and in ilmenite from Ronda. We also believe that the inclusions reported by Henriquez & Darling (2009) in the Adirondacks (USA) should be nanogranites.
Although inclusions of nanogranite are rare in samples from the KKB and constitute scattered small clusters within the large garnet porphyroblasts, in most other occurrences they are arranged zonally in the cores of crystals (e.g., Figure 1A, B). An excellent example is provided by the migmatites of Ronda, where, regardless of the small crystal size (<0.2 mm), all the garnet cores are clouded with inclusions (Figures 1A and 8). Nanogranite inclusions are smaller than MI in the Spanish enclaves: the statistics on 244 measurements of nanogranites in the garnets from the KKB provided a mean and maximum diameter of 13 and 25 µm, respectively (Cesare et al., 2009). In the garnets of Ronda inclusions are even smaller, often around 5 µm in diameter (Figure 9). Nanogranite inclusions in garnet are typically facetted, with a dodecahedral (Figure 10) negative crystal shape; more rarely they are round or tubular.
Figure 10. Examples of negative crystals in MI in garnet from the KKB (A) and the Ivrea Zone (B)
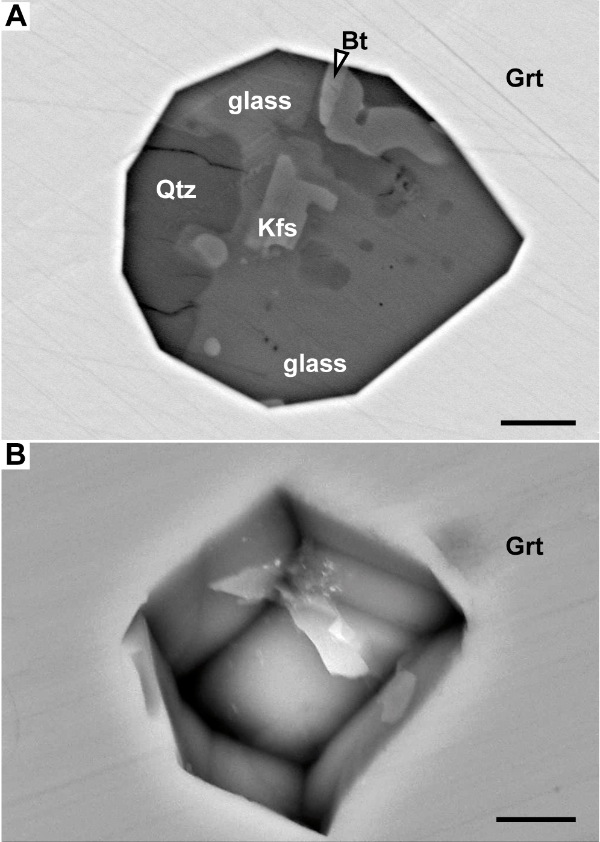
In the latter image, the MI has been emptied by mechanical removal during sample preparation, leaving a facetted rhombododecahedral pit in the garnet. Scalebars: 2 µm
Inclusions contain aggregates of crystals with equigranular, hypidiomorphic to allotriomorphic texture. Sometimes granophyric to nano- to microgranophyric intergrowths of quartz and feldspars are also present (Figure 11A). Crystal grain-size ranges from hundreds of nm to a few µm, and minerals – especially micas - often appear to have grown from the inclusion walls, which represent preferred nucleation sites. Owing to these microstructural and compositional features (see below), the cryptocrystalline aggregate found within inclusions was named “nanogranite” (Cesare et al., 2009). SEM investigation of polished nanogranite inclusions exposed at the surface of host garnet suggests highly variable phase abundances and ratios. For example Figure 7b, showing a high proportion of biotite, would suggest that the trapped melt that crystallized wasn't an anatectic leucogranite, or that some peritectic biotite was entrained together with the melt in the inclusion. Since we were able to remelt completely the nanogranite inclusions (see below), since the composition of remelted glasses is homogeneous, and since the only unmelted crystals preserved in inclusions are zircon, apatite and ilmenite, we conclude that, owing to cut effects, the modal information that can be gained from SEM images are apparent and can be misleading, and that two-dimensional modal analysis of these small inclusions is unreliable. Other minerals, such as apatite, graphite, ilmenite, rutile and zircon, are also present in the nanogranites (e.g., Figures 11A to C); in most cases, these phases are interpreted as solid inclusions that were present at the mineral-melt interface during MI entrapment. This is particularly evident for the case of rutile needles associated with nanogranites in the garnets of Ronda (Figure 11B).
Figure 11. SEM microstructures of MI in migmatites
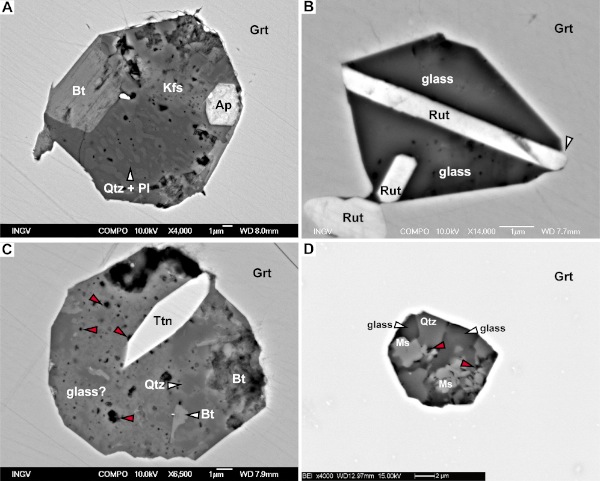
A: micrographic texture of finely intergrown quartz and plagioclase (arrow) in a nanogranite inclusion from the KKB. B: in some migmatites from Ronda rutile is the most common trapped mineral in the MI. The bottom right termination of the larger rutile needle (arrow) is partially enclosed in the host garnet, supporting the inference that rutile was trapped together with a melt droplet by the growing host. C, D: micro-to nano-porosity (red arrows) is often visible in nanogranites in samples from both KKB (C) and Ronda (D).
Nanogranite inclusions display a variable micro- to nano-porosity (Figures 11 C and D), that is commonly greater in the samples from Ronda. Although microcavities in inclusions exposed on the sample surface prepared for EMP or SEM investigation might be an artifact due to polishing, we have verified by Raman spectroscopy the presence of fluid-filled pores in nanogranite inclusions below the sample surface. These observations support the inference made by Cesare et al. (2009) that porosity forms by melt crystallization due to the higher density of solids with respect to the trapped melt. In the case of hydrous melts, the porosity is likely to contain exsolved fluids, in particular H2O.
Diametrically opposite decrepitation tails often occur where the host shows evidence of deformation and microcracking (Figure 12). These inclusions and samples should be avoided as decrepitation may have induced or facilitated alteration of the original microstructural and compositional features of the inclusion.
Figure 12. Diametrically opposite decrepitation tails in MI in garnet
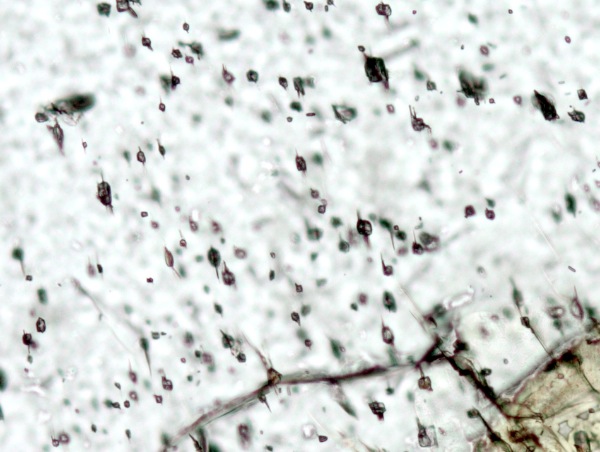
The orientation of microcracks is parallel to the major set of cracks in the host garnet. Width of view: 0.3 mm.
Microstructural features of the nanogranites that indicate crystallization from a melt are: (i) euhedral mineral shapes, in particular of biotite; (ii) high interfacial energy boundaries between minerals; (iii) nanogranophyric intergrowths, commonly recognized in plutonic rocks; and (iv) cuspate, thin elongate pockets or films of an unknow phase (possibly amorphous, still requires TEM investigation) that strongly resemble melt pseudomorphs, although 2-3 orders of magnitude thinner (Figure 13) than those previously described (Holness and Sawyer, 2008).
Nevertheless, the most convincing evidence that these inclusions represent trapped anatectic melts is that the melt phase, quenched as glass, can still be found within them (see below).
Figure 13. SEM microstructures of MI in migmatites
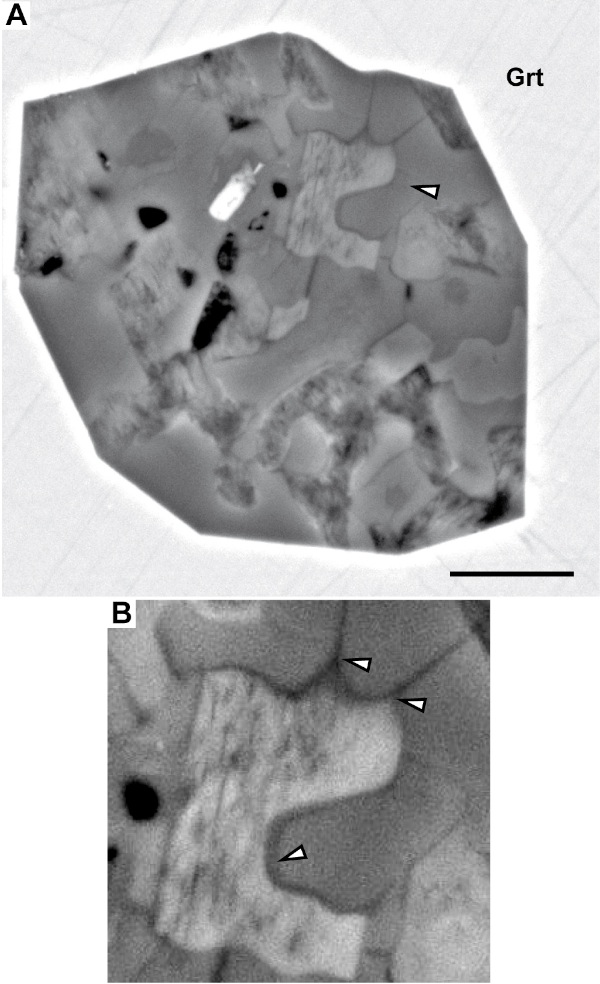
A) a nanogranite inclusion in garnet from the KKB. Arrow points to area enlarged in B. Scalebar: 2 µm. B) cuspate-lobate thin layers (arrows) of an undetermined phase define a microstructure that resemble melt pseudomorphs (reprinted from Cesare et al., 2009).