Hypothesis 2 (megacrysts grow after all or most crystallization)
A recent paper by Johnson and Glazner [2010] marks a return to an old idea, that K-feldspar megacrysts form late in granitoids — too late for enough free liquid to be available for them to grow large and for any movement and concentration of megacrysts to occur. The mechanism (’thermal recycling’) proposed by Johnson and Glazer [2010] is that the K-feldspar megacrysts grow very late, after dissolution of smaller already crystallized K-feldspar grains, in response to multiple episodes of reheating and fluid fluxing caused by arrivals of later magma batches. They wrote that “coarsening may occur during thermal fluctuations with small amounts of melt remaining, during small-degree partial remelting, or in the presence of a fluid phase.”
Johnson and Glazner [2010] and Glazner and Boudreau [2010, p. 327] have asserted that K-feldspar megacrysts are "almost universally interpreted as early-forming crystals". On the contrary, Vernon [1986, p. 52] and Vernon and Paterson [2008b] have emphasized that experiments indicate that K-feldspar nucleates late in the sequence of mineral appearances, referring to the experimental results of Piwinskii [1968], Piwinskii and Wyllie [1968], Whitney [1975] and Clemens and Wall [1981]. However, the important point is that the magma can have 60-70% liquid when the K-feldspar crystallizes— sufficient for it to grow large crystals capable of moving into flow alignment and forming physical accumulations [Clemens and Wall, 1981, p. 116; Winkler and Schultes, 1982, pp. 560-561]. In contrast, the assertion of Johnson and Glazner [2010] that insufficient liquid is available for growth and movement of megacrysts does not agree with the experimental results. Hypothesis 2 encounters the following ten problems.
(1) Abundance of liquid when megacrysts grow: The availability of abundant liquid is verified by the excellent evidence of unimpeded growth of a K-feldspar megacryst shown in Figure 2, which depicts a porphyritic rock that was chilled (forming a fine-grained quartzofeldspathic groundmass), containing large, euhedral K-feldspar megacrysts surrounded by former liquid, as well as much smaller euhedral phenocrysts of plagioclase, quartz and biotite. The rapid cooling has preserved evidence of unimpeded growth of K-feldspar in liquid, either later than or at the same time as plagioclase, biotite and quartz. The K-feldspar grew relatively rapidly from few nuclei and had plenty of space in which to grow freely and so develop crystal faces. This rock shows unequivocally that K-feldspar megacrysts can grow in liquid as normal euhedral phenocrysts. Other illustrations of rapidly cooled felsic rocks with independent K-feldspar megacrysts separated by abundant groundmass have been presented by Vernon [2004, figure 3.13] and McDonnell et al. [2004, figure 2]. In contrast, Figure 14 shows a large oikocryst of K-feldspar, with numerous, small, predominantly euhedral inclusions of plagioclase, biotite and hornblende, in a synplutonic microgranitoid dyke. Though the K-feldspar in this rock also grew from few nuclei and developed very large grains, the proportion of already crystallized minerals evidently was too large to permit the K-feldspar to grow as a euhedral megacryst.
Figure 21. Mixing of K-feldspar megacrysts into mafic replenishment
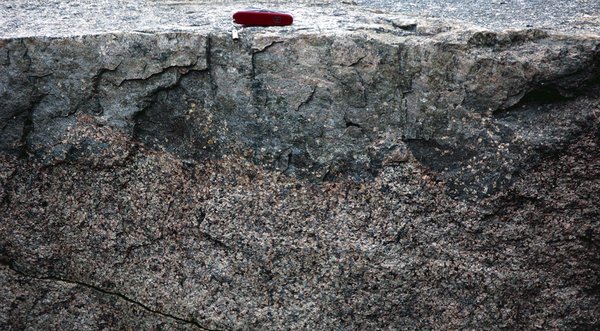
Outcrop view of arrested mixing and dispersal of K-feldspar megacrysts from porphyritic granitoid cumulate into overlying mafic sheet, Cadillac Mountain pluton, Mount Desert Island, Maine, USA. Knife 9 cm long.
(2) Space problem for late growth: The hypothesis of Johnson and Glazner [2010] encounters the same problem that its authors ascribe to the normal phenocryst hypothesis, namely that space has to be provided for the megacrysts, which would be especially difficult where most of the crystallization of the rock has already occurred. The authors do not explain how sufficient room could be made for the development of megacrysts if only small amounts of melt were present, or how the scattered pockets of liquid produced by the putative melting of small K-feldspar crystals coalesce to form pools large enough to accommodate megacrysts. The deficiency of small K-feldspar crystals in the presence of large ones, observed by Johnson and Glazner [2010], can be explained by megacryst growth as normal phenocrysts. It is much easier to make space where 60 to 70% of the liquid is available, and so it is more reasonable to accept that K-feldspar megacrysts grow as normal phenocrysts, and that sufficient liquid remains for them to undergo some movement and physical accumulation in granitic magmas.
(3) Overgrowths on megacrysts: Once nucleated, K-feldspar megacrysts grow rapidly, compared with growth rates of the other minerals present, as indicated by their size. Fenn [1977] and Swanson [1977] observed experimental growth rates (several millimetres in several days) that are fast enough to produce, in a reasonable time, feldspar crystals as large as any found in granitoids. Evidently enough time is available for megacrysts to reach large sizes before space restrictions force the megacrysts to grow in an interstitial manner, commonly forming crystallographically continuous off-shoots (overgrowths) from the rims of the megacrysts between adjacent grains. These overgrowths are consistent with continued magmatic growth after euhedral megacrystic growth ceased, the overgrowths being impeded mainly by simultaneously crystallizing quartz and feldspar grains [Anderson, 1934; Bateman et al., 1963, p. D16; Exley and Stone, 1964, pp. 135, 159; Booth, 1968, p. 1036: Emmermann, 1969, p. 292: Wilshire, 1969, p. 243; Higgins and Kawachi, 1977, p. 275; Kawachi and Sato, 1978; Vernon, 1986a, p. 5; Higgins, 1999; Vernon and Paterson, 2008]. However, Johnson and Glazner [2010] regarded these interstitial ‘tendrils’ as “remnant pathways by which interstitial K-feldspar migrated toward the megacrysts.” This speculation cannot explain how each independently originating ‘tendril’ (joining others only where they reach the edge of the megacryst) could acquire the same crystallographic orientation.
(4) Sizes of inclusions in megacrysts: The sizes of inclusions in K-feldspar megacrysts are usually inferred to reflect the sizes of crystals of the included minerals at the time the megacrysts incorporated them [Kerrick 1969; Mehnert and Büsch, 1981; Vernon, 1986; Vernon and Paterson, 2008a]. This interpretation is confirmed by the small crystals of plagioclase, quartz and biotite in the groundmass around the megacryst in Figure 2. However, Johnson and Glazner [2010] suggested that the inclusions are small because any larger crystals would accumulate (presumably by being pushed aside) at the edges of the growing megacryst — an intuitive idea that is unsupported by microstructural evidence. The common presence of euhedral plagioclase inclusions with normal zoning indicates that the plagioclase crystals were growing independently in the liquid before being incorporated in the K-feldspar megacrysts. As Swanson [1977, figure 3b] found experimentally for a granodioritic composition, the growth rate of plagioclase is much lower than that of alkali feldspar at the degree of undercooling at which alkali feldspar reaches its maximum growth rate. Therefore, a growing megacryst has a good chance of encountering and enclosing plagioclase grains much smaller than itself. In contrast, K-feldspar inclusions in plagioclase are rare, as they are generally too large, though small inclusions of K-feldspar in plagioclase phenocrysts have been described [e.g., Boone, 1962, figure 4].
(5) Megacrysts involved in magma mixing: Another problem with the Johnson and Glazner [2010] hypothesis is the common incorporation of K-feldspar megacrysts into mafic magma replenishments in granitic magma chambers [e.g., Vernon, 1983, 1984, 1986, 1990, 1991; Reid et al., 1983; Wiebe, 1993, 1994, 1996; Wiebe et al., 2002; Collins et al., 2006], as shown in Figures 21, 22, 23 and 24. This situation requires that the megacrysts exist independently in enough liquid to mix with the more mafic magma, which would be very difficult or impossible if the megacrysts formed late in largely solid rock.
Figure 22. K-feldspar megacrysts in microgranitoid enclave
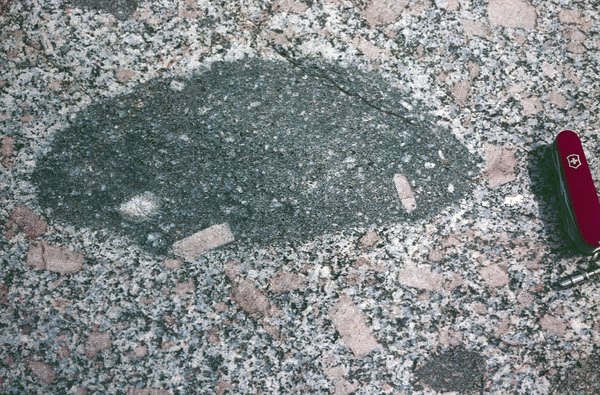
Microgranitoid enclave with megacrysts of K-feldspar derived from the host magma, Bungulla Adamellite, northern New England Batholith, New South Wales, Australia. Knife 9 cm long.
Figure 23. Microgranitoid enclave with megacrysts
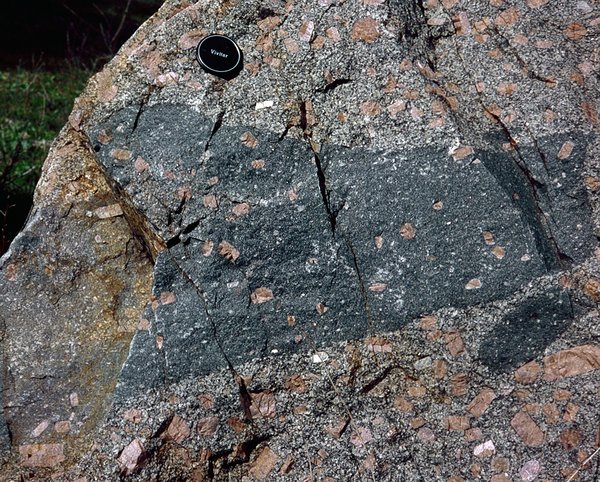
Microgranitoid enclave with abundant megacrysts of K-feldspar derived from the host magma, Wuluuman pluton, Wellington, New South Wales, Australia.
Figure 24. Megacrysts mixed into enclave magma
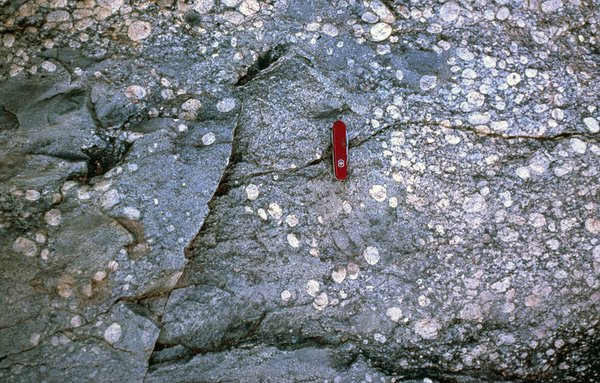
K-feldspar megacrysts (some with plagioclase rims, forming rapakivi stucture) from the surrounding megacrystic granitoid variably dispersed through more mafic magma, Yundurbulu Batholith, Mount Stafford, central Australia. Knife 9 cm long.
(6) Supposed ‘idiomorphic tendency’ of K-feldspar, but not quartz: Johnson and Glazner (2010) stated that “selective coarsening of K-feldspar relative to other phases is governed by the idiomorphic tendencies of K-feldspar …. In contrast, quartz also crystallizes late but rarely forms euhedral crystals in granites, presumably because it is less idiomorphic.” On the contrary, both quartz and K-feldspar develop crystal faces when growing in liquid, as is well known from felsic volcanic rocks, porphyritic microgranitoids (Figures 2, 3, 15) and some granitoids (Figures 18, 25). Euhedral growth of both K-feldspar and quartz is confirmed by euhedral oscillatory zoning patterns (Figures 6, 18, 19), though external boundaries of these minerals in granitoids are commonly anhedral (Figures 6, 19), owing to impingement with adjacent growing grains or minor grain-boundary migration during weak deformation.
Figure 25. Early euhedral quartz
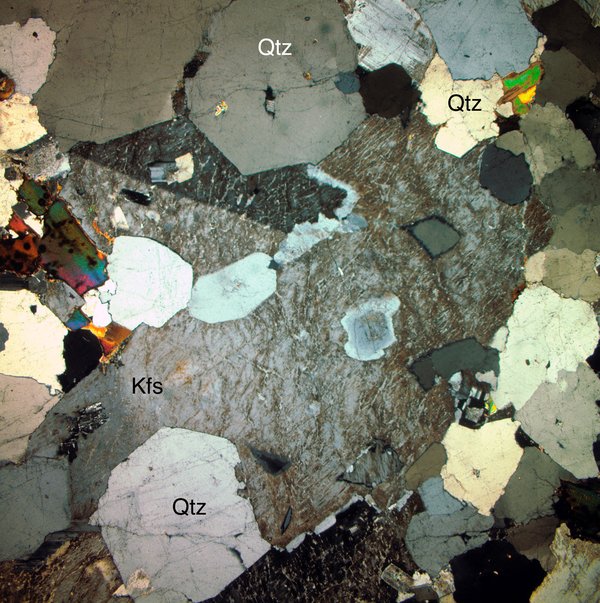
Euhedral crystals of quartz (Qtz) projecting into K-feldspar (Kfs) in granite. Crossed polars; base of photo 7 mm.
(7) K-feldspar concentrations in granitoids: Concentrations of euhedral K-feldspar megacrysts in granitoids [Gilbert, 1906; Cloos, 1936; Phillips, 1968, p. 180; Wilshire, 1969, p. 244; Wahrhaftig, 1979; Barrière, 1981; Vernon, 1986, pp. 7-8; Abbott, 1989; Reid et al., 1993; Tobisch et al., 1997; Clarke and Clarke, 1998; Weinberg et al., 2001; Paterson et al., 2005; Vernon and Paterson, 2008a], as shown in Figures 26 and 27, are usually ascribed to physical accumulation. The common magmatic flow alignment of megacrysts [e.g, Gilbert, 1906; Vernon, 1986a; Clarke and Clarke, 1998; Paterson et al., 2005; Vernon and Peterson, 2008a] indicates that the megacrysts are able to rotate in liquid without deforming plastically. The alternative explanation of Higgins [1999] and Johnson and Glazner [2010] for K-feldspar megacryst concentrations is that solution and regrowth of K-feldspar are concentrated at selected places. However, as noted by Vernon [2004, pp. 64-65], this hypothesis fails to explain why (a) only K-feldspar was precipitated, without quartz, plagioclase and biotite (with which the inferred percolating felsic liquid should also have been saturated), and (b) the concentrated K-feldspar megacrysts typically do not interpenetrate, even where in contact, but tend to remain as separate solids in the concentrating process, whereas simultaneous growth would be expected to produce moulding of one crystal around another. Mechanical concentration is a simpler explanation, which avoids remelting and/or K metasomatism to concentrate the K-feldspar.
Figure 26. Megacryst accumulation
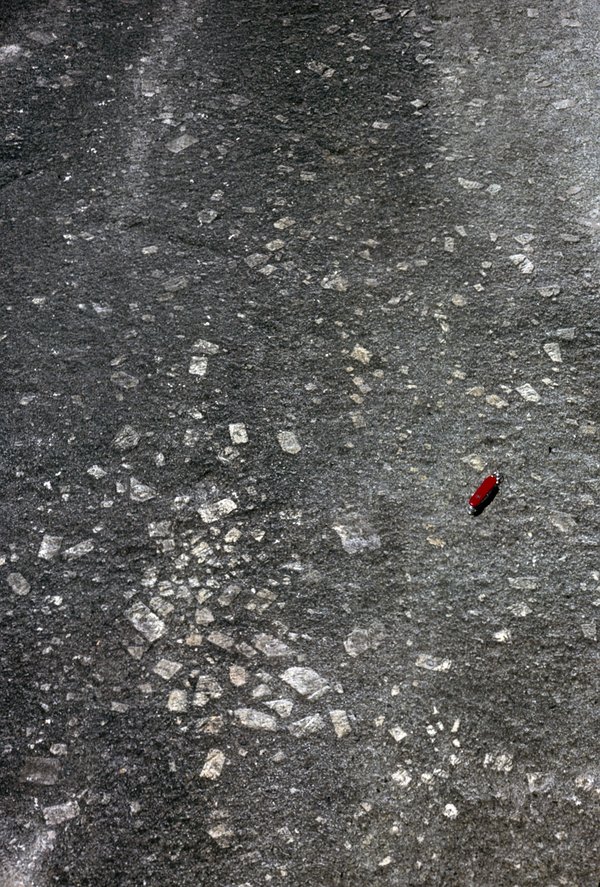
Patch rich in euhedral K-feldspar megacrysts, Cathedral Peak pluton, Tuolumne Batholith, California, USA. The distribution appears to be the result of accumulation, not in situ growth, because the proportion of K-feldspar is much higher than in the bulk of the pluton and is too high for a local magma composition. Knife 9 cm long.
Figure 27. K-feldspar-rich granitoid cumulate
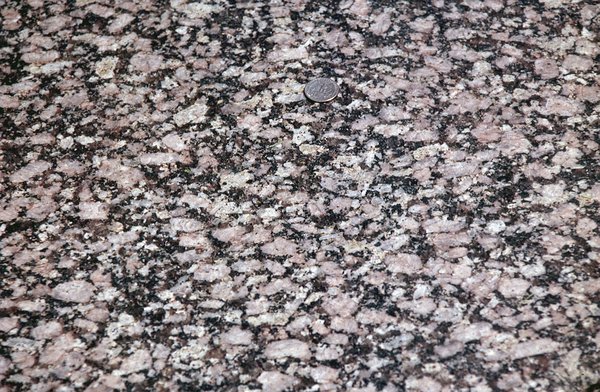
Megacrystic granitoid inferred to have become enriched in megacrysts by physical accumulation, removal of liquid, or both. The strong alignment of the megacrysts reflects magmatic flow, probably involving rotation of the crystals during compaction. United States quarter dollar for scale.
(8) Rarity of megacrysts in felsic volcanic rocks: Johnson and Glazner [2010] claimed that because K-feldspar phenocrysts commonly do not occur in dacitic volcanic rocks, they cannot move and accumulate in clusters in magma chambers. However, intuitive assertion is a poor substitute for evidence, and the presence of trough structures with graded layers, as well as local megacryst-rich intrusions [e.g., Weinberg et al., 2001; Paterson et al., 2005; Vernon and Paterson, 2008a] should not be ignored. Many granitoids show evidence of crystal accumulation, by mechanical movement of crystals or crystal aggregates [Paterson et al., 2005; Vernon and Paterson, 2008a], removal of liquid [McMurry, 2001; Vernon and Collins, 2011], or both. Some megacryst-rich accumulations could occur by extraction of the residual liquid commonly inferred to be responsible for many felsic volcanic and tuffaceous rocks. An example is the Bodocó quartz-monzonite pluton, Brazil, which shows no isotopic variation, but considerable modal variation, including K-feldspar megacryst enrichment, which can be attributed to in situ crystal accumulation consequent on loss of interstitial felsic liquid [McMurry, 2001]. The common inability of megacryst-rich magmas to erupt as such to the surface could reflect loss of interstitial liquid or a relatively low volatile content of the magma, even at the stage when megacrysts are mobile, and so need have no bearing on the previous ability or inability of megacrysts to move, relative to liquid, in plutons.
(9) Consequence of repeated replenishment: Johnson and Glazner [2010] asserted that “granitic plutons are emplaced incrementally and … megacrystic textures are evidence of such an emplacement process” (that is, ‘thermal recycling’ occurs as a result of replenishment), but that “some compositionally indistinguishable granitic plutons contain K-feldspar megacrysts whereas others do not.” These statements combined raise a problem for the Johnson and Glazner [2010] hypothesis, because the repeated replenishment the authors infer to be responsible for the formation of all granitoid plutons, should cause ‘thermal recycling’ and hence growth of megacrysts in all of them.
(10) Rapakivi structure and K-feldspar antecrysts: K-feldspar megacrysts with plagioclase rims (‘rapakivi structure’) dispersed through non-mantled megacrysts (Figures 28, 29) imply that crystals that previously had reacted with liquid (because of changed P-T-XH2O conditions or magma mixing) were derived from another magma or a different part of the same magma, and then mixed with non-reacted crystals precipitated from the host magma. Though foreign, such ‘antecrysts’ are nevertheless magmatic. Moreover, because of their evidence of reaction and replacement and/or mantling, these crystals grew early enough for magmatic reaction or later mixing to occur.
Figure 28. Rapakivi antecrysts
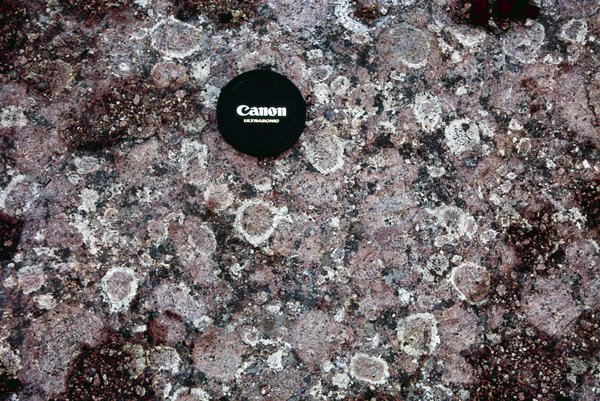
Rapakivi granite, southern Finland, showing K-feldspar megacrysts with light-coloured plagioclase rims (antecrysts) dispersed through non-mantled megacrysts. Despite the presence of lichen on the outcrop, the contrast between mantled and non-mantled megacrysts is clearly shown.
Figure 29. Antecrysts in rapakivi granite
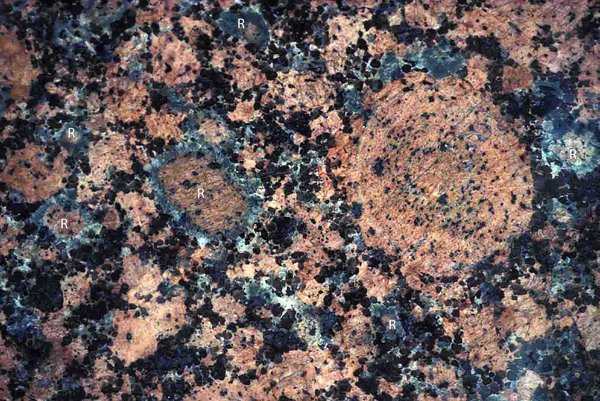
Sawn slab of rapakivi granite, southern Finland, showing K-feldspar megacrysts (R) showing grey plagioclase rims (antecrysts), labelled “R”, dispersed through non-mantled megacrysts. Base of photo c. 30 cm.
General inference about late K-feldspar in granitoids: The foregoing discussion confirms that, though K-feldspar typically nucleates late in the crystallization history, it generally has plenty of liquid volume in which to develop large crystals, which consequently can move, relative to liquid, as witnessed by numerous examples of flow-aligned megacrysts and megacryst-rich cumulates.