Hypothesis 3 (granites are effectively metamorphic rocks)
Many petrologists have assumed, generally without presenting evidence, that extensive subsolidus grain-shape changes occur as granitoids cool [e.g., Spencer, 1937; Drescher-Kaden, 1948; Augustithis, 1973; Pitcher, 1979; Hughes, 1982, p. 162; Hibbard, 1995, p. 228; Pitcher, 1997 p. 69; Bindeman, 2003, p. 367; Bachmann et al., 2007, p. 7; Kemp et al., 2008, p. 337]. For example, Tuttle [1952] and Tuttle and Bowen [1958] speculated that independent grains of K-feldspar and plagioclase in some granitoids are formed by extreme solid-state exsolution from a single feldspar, and Luth [1976, p. 336] stated that “most granitic rocks exhibit textural and mineralogical features which are less related to the ultimate magmatic origin and early history than to subsequent sub-solidus recrystallization.” O’Brient [1986, p. 608] referred to “complete subsolidus recrystallization that affects all but the shallowest and most rapidly quenched intrusions” and asserted that “plutonic rocks only indirectly preserve primary magmatic features.” Bartley et al. [2004] and Coleman et al. [2005] suggested that recrystallization (by which they presumably implied grain growth, rather than strain-induced recrystallization) in granitoids can obscure or even obliterate major structures, such as internal contacts in plutons. Similarly, Glazner and Bartley [2006] stated (also without presenting evidence) that “recrystallization is a well-established mechanism for rearranging crystal boundaries and obliterating contacts between lithologically identical rocks” in granitic intrusions. In addition, Coleman et al. [2005, p. 18] stated that the structures shown by K-feldspar in the Tuolumne Batholith, California, USA, “are not clearly igneous … and have been significantly modified by late-stage processes,” again without presenting evidence.
Perhaps the broadest assertions of this kind have been made by Coleman et al. [2005, p. 1], who stated that “thermal models indicate that slow incremental growth can maintain much of a pluton at temperatures corresponding to the amphibolite facies of metamorphism for extended time periods” promoting “subsolidus textural modification that is likely to further obscure any primary intrusive contacts between intrusive increments,” and McBirney [2009, p. 4], who wrote that “the textures of most coarse-grained igneous rocks are essentially metamorphic… As they cool, the minerals pass through a range of conditions in which a metamorphic petrologist would normally expect there to be conspicuous changes, including both compositional and textural re-equilibration of the kind seen in other rocks under comparable conditions.”
The foregoing assertions about grain-shape changes during slow cooling in granitoids evidently spring from the inferred long cooling times of large felsic plutons, leading to understandable speculations that they must therefore change their microstructures to resemble high-grade metamorphic rocks. However, Vernon and Paterson [2008c] presented detailed structural evidence that extensive grain-shape changes typically do not occur in cooling granitoids, in the absence of tectonic deformation or metamorphism, though minor grain-boundary adjustments can occur locally, and intragranular changes (such as exsolution and deuteric alteration) are common. Hypothesis 3 encounters the following four main problems.
(1) Contrast with mafic rocks: Grain-shape adjustments evidently do occur in mafic-ultramafic cumulate rocks that cool slowly from very high temperatures, because many such rocks have grain shapes indicative of solid-state grain-boundary adjustment [e.g., Voll, 1960; Weedon, 1965; Vernon, 1970, 1976, 2004, pp. 224-229; Hulbert and von Gruenenwaldt, 1985; Reynolds, 1985; Mathison, 1987; Hunter, 1987, 1996; Higgins, 1991, 1998; Waters & Boudreau, 1996; Duchesne, 1999; Holness, 2005, 2010; Holness et al., 2005; O’Driscoll et al., 2010], as shown in Figures 30, 31, 32, 33 and 34. Vernon [1970, 2004] found that some gabbros and ultramafic cumulates are essentially polygonal aggregates (Figure 34, 35) that resemble granulite facies metamorphic aggregates (Figure 36), except that they commonly preserve some residual elongate igneous shapes without crystal faces (Figure 37). On the other hand, many gabbros preserve abundant elongate plagioclase crystal shapes and euhedral plagioclase inclusions in pyroxene, and hence have an overall igneous appearance (Figures 38, 39). Vernon [1970] found that dihedral angles in some mafic/ultramafic cumulates are similar to those in typical granulite facies compositional equivalents, but Holness [2005 and this volume] and Holness et al. [2005] measured variable dihedral angles in aggregates in some individual oikocrysts, suggesting that equilibration of interfacial angles and change of igneous microstructure are limited, compared with aggregates of the same minerals in granulite facies metamorphic rocks. Evidently, mafic and ultramafic plutonic rocks are capable of undergoing extensive subsolidus grain-boundary changes in the absence of imposed metamorphism and deformation, but minimum-energy grain and inclusion shapes are not always achieved.
Figure 30. Subsolidus grain-shape adjustment in gabbro
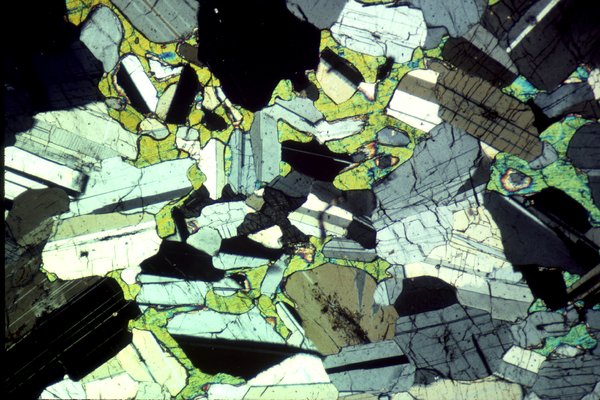
Gabbro from the Bushveld mafic-ultramafic igneous complex, South Africa, showing moderately aligned, elongate grains of twinned plagioclase, with clinopyroxene containing inclusions of plagioclase in ophitic to subophitic intergrowths. The inclusions of plagioclase are smaller than the plagioclase grains outside the clinopyroxene, reflecting the shorter growth period of the included plagioclase. The rounded corners of the plagioclase inclusions, together with the clinopyroxene versus plagioclase/plagioclase dihedral angles indicate that solid-state adjustment of grain boundaries occurred during slow cooling. Crossed polars; base of photo 4.4 mm. From Vernon (2004, figure 3.46).
Adcumulates typically have polygonal grain shapes (Figures 33, 34, 35, 37), whereas non-cumulate ophitic gabbros commonly show weak or no evidence of grain-shape changes with cooling (Figure 39). This difference could be due to the tendency for ‘sintering’ to occur in cumulate aggregates in liquid (leading to polygonal grain shapes), whereas simultaneously crystallizing plagioclase and pyroxene in ophitic gabbros and dolerites produce liquid-free intergrowths that would not show sintering tendencies.
Figure 31. Subsolidus changes in gabbro
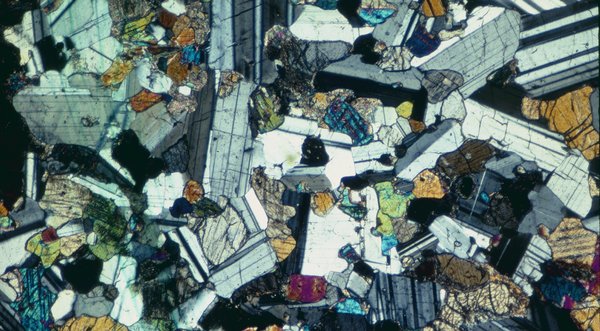
Gabbro, Days Creek, New England, New South Wales, Australia, with abundance of curved, irrational grain boundaries, polygonal grain shapes, and dihedral angles between plagioclase and pyroxene, though retaining many elongate plagioclase shapes reflecting a magmatic origin. Crossed polars; base of photo 4.4 mm.
Figure 32. Polygonal grain shapes in gabbro
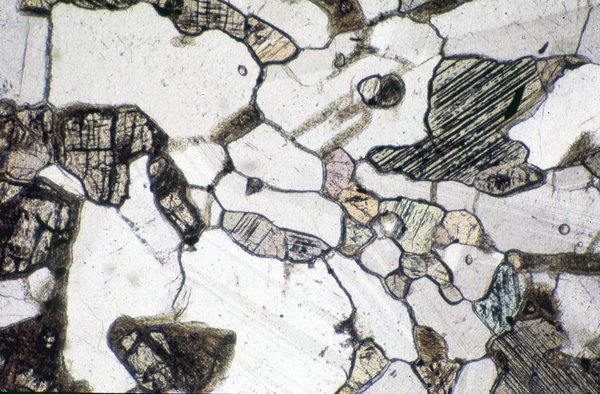
Gabbro dominated by polygonal grain shapes, rounded inclusion shapes, and curved grain boundaries, reflecting extensive subsolidus grain-shape adjustment. Plane-polarized light; base of photo c. 2 mm.
Hotter intrusions and deeper, more slowly cooled intrusions would be expected to undergo the most extensive grain-shape changes during cooling (Marian Holness, personal communication), other factors (such as deformation) being uninvolved. However, the gabbro illustrated in Figure 31 shows almost no residual crystal faces, though many of the plagioclase grains are elongate, reflecting approximate original shapes and sizes. The intrusion is small, occurs in low-grade rocks, and does not appear to have been metamorphosed or deformed. The reasons for the grain-shape changes in this rock are unclear, but could be connected with an original cumulate origin, which promoted some high-temperature ‘sintering’.
Figure 33. Polygonal grain shapes in troctolite
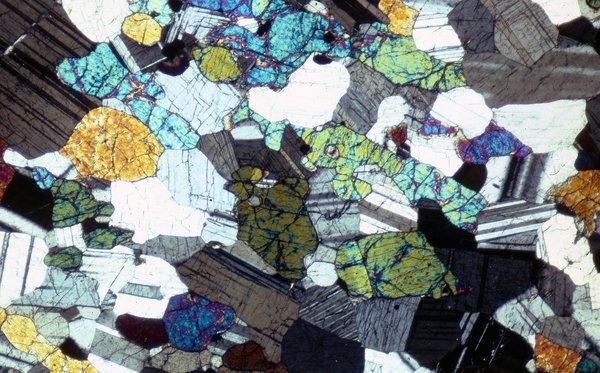
Troctolite, Rhum layered igneous complex, Scotland, dominated by polygonal olivine and plagioclase grain shapes formed by grain-boundary movement during slow cooling from high temperature. Crossed polars; base of photo 4.4 mm.
Figure 34. Polygonal olivine in dunite
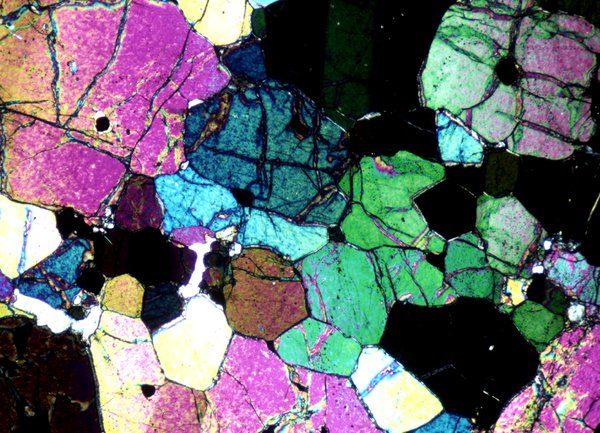
Ultramafic cumulate (dunite) showing polygonal olivine grain shapes formed by grain-boundary movement during slow cooling from high temperature, Rhum layered igneous complex, Scotland. Crossed polars; base of photo 4.4 mm.
Figure 35. Polygonal plagioclase in anorthosite
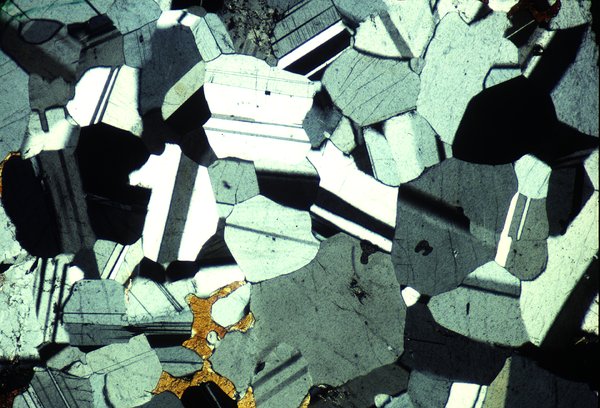
Polygonal aggregate of calcic plagioclase in an anorthosite cumulate from the Bushveld Complex, South Africa. Crossed polars; base of photo 4.4 mm. From Vernon (2004, figure 4.41A).
Figure 36. Granulite facies mafic microstructure
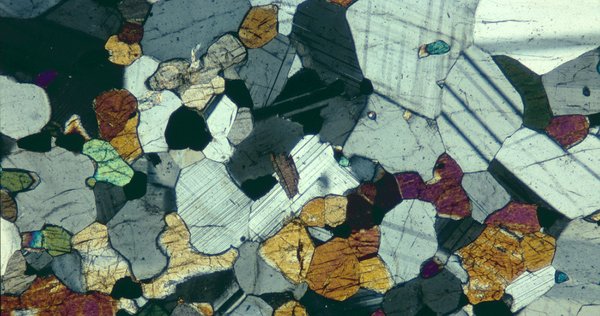
Polygonal grain shapes of plagioclase and pyroxene that are typical of the microstructure of granulite facies mafic metamorphic rocks. Crossed polars; base of photo 4 mm.
Figure 37. Anorthosite with residual elongate grain shapes
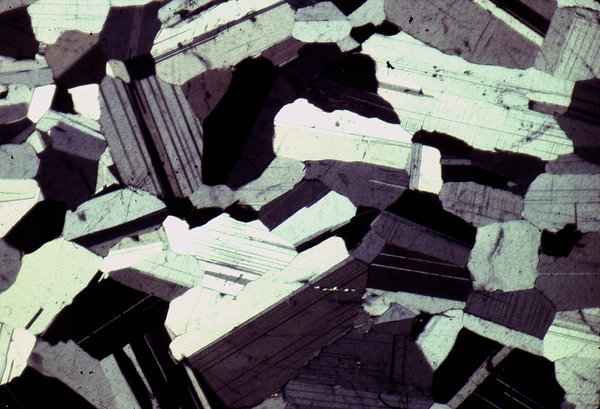
Polygonal aggregate of calcic plagioclase, in a section perpendicular to that shown in Figure 35. Despite the solid-state grain-boundary adjustment, elongate magmatic grain shapes aligned by magmatic flow have been preserved. Crossed polars; base of photo 4.4 mm. From Vernon (2004, figure 4.41B).
Figure 38. Gabbro microstructure
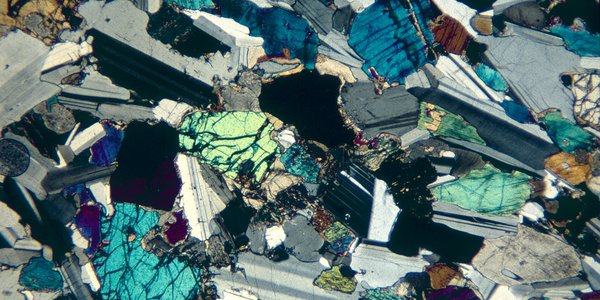
Gabbro consisting of olivine, pyroxene and elongate plagioclase grains. The elongate plagioclase grains reflect a magmatic origin, compared with the typically polygonal grains of high-grade metamorphic rocks (Figure 36), but most grain boundaries are irrational, reflecting subsolidus adjustments Crossed polars; base of photo 4 mm.
Figure 39. Ophitic structure very well preserved in gabbro
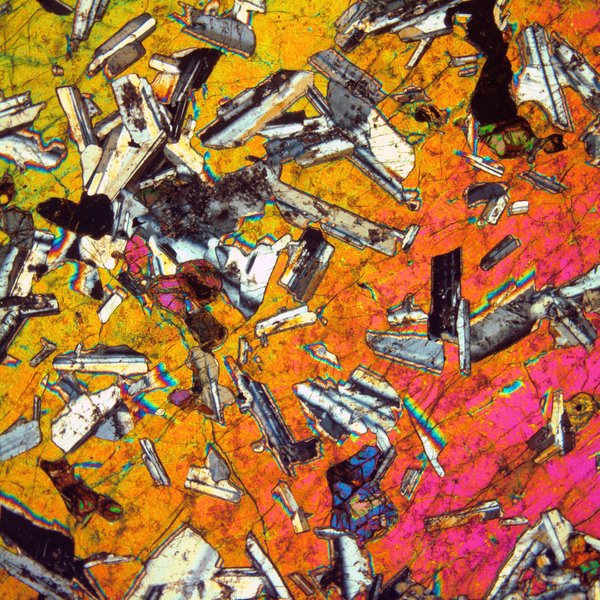
(A) Large grain of clinopyroxene with inclusions of elongate plagioclase grains, forming an ophitic microstructure in gabbro. The rounded corners of some of the plagioclase inclusions suggest minor solid-state adjustment during slow cooling, but most inclusions are euhedral and the overall igneous microstructure is retained. Crossed polars; base of photo 7 mm.
(B) Similar situation to that shown in (A), but with more rounding of plagioclase corners, suggesting a higher degree of solid-state adjustment during cooling, but still with preservation of the igneous structure. Crossed polars; base of photo 3.5 mm.
Figure 40. Oscillatory zoning in quartz and feldspar
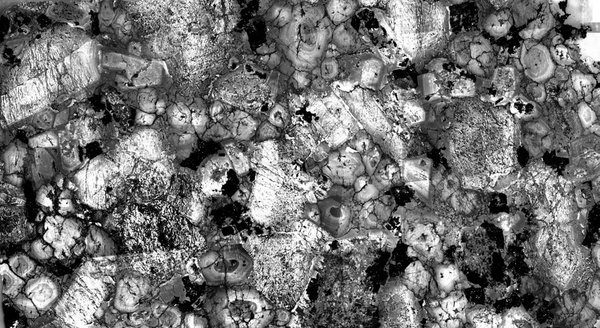
Quartz grains with euhedral concentric oscillatory Ti zoning, revealed by SEM cathodoluminescence, reflecting former crystal growth habits, Vinalhaven pluton, Maine, USA. Some of the zoning patterns indicate rounded former shapes suggesting repeated dissolution, followed by euhedral regrowth. Oscillatory zoning is also present in the plagioclase and K-feldspar (poorly polished). The zoning patterns in all three minerals are evidence of magmatic crystallization. Base of photo 40 mm. Photo by Bob Wiebe.
In contrast to the behaviour of hotter mafic rocks, microstructural evidence (discussed below) indicates that major grain-shape changes during cooling do not occur in most granitoids, in the absence of tectonic deformation. As McBirney [2009, p. 4] wrote: “the story of plutonic rocks does not end with precipitation of a stable mass of cotectic minerals”, because of the abundant evidence of subsolidus intragranular changes (e.g., exsolution in alkali feldspar) and deuteric alteration in granitoids, but these processes typically do not involve major grain-shape changes [Vernon and Paterson, 2008c], even for granitoids in which subsolidus intragranular changes are prominent [e.g., Haapala, 1997]. The contrast between the behaviour of granitoid cumulates and mafic-ultramafic cumulates is probably due mainly to the lower solidus temperatures and hence smaller cooling temperature ranges of granitoids.
(2) Preservation of oscillatory zoning: The putative grain shape changes during cooling would be most likely to occur by high-temperature recrystallization, involving the formation of smaller new grains or the modification and rearrangement of existing grains by grain-boundary ‘sweeping’ during grain boundary migration recrystallization (‘fast grain boundary migration’), which is most effective at high temperatures, especially if water is present [e.g., Vernon, 2004, pp. 338-342; Vernon and Clarke, 2008, p. 273]. Vernon and Paterson [2008c] emphasized that oscillatory zoning would be truncated or even removed by grain-boundary ‘sweeping’. On the contrary, oscillatory and other zoning patterns are typically well preserved in granitoids, and are not appreciably truncated by grain boundaries (Figures 1, 5, 6), except for uncommon examples of 'contact melting' resulting from impingement at the magmatic stage (Figure 7) and internal pattern truncations resulting from corrosion in response to magma mixing during crystal growth (Figure 6). Some granitoids are characterized by complete oscillatory zoning patterns in all three felsic minerals, quartz, plagioclase and K-feldspar [e.g., Wiebe et al., 2004], as shown in Figure 40, and, as mentioned previously, oscillatory zoning can also occur in a wide range of common granitic minerals, such as zircon, allanite, sphene, apatite, cordierite, hornblende and biotite.
Figure 41. Micrographic intergrowth
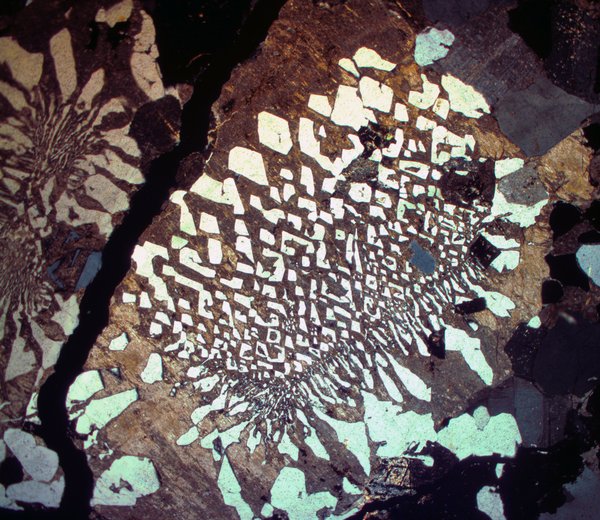
Graphic intergrowth (granophyric variety) of quartz and alkali feldspar in granite, Tallong, New South Wales, Australia. Preservation of this structure, without any tendency to produce rounded or polygonal grain shapes testifies to a lack of solid-state grain-boundary adjustment during cooling. Crossed polars; base of photo 7 mm.
(3) Preservation of other igneous microstructures: Characteristic magmatic features, such as elongate, euhedral to subhedral feldspar or quartz grains, euhedral plagioclase inclusions in quartz and K-feldspar, and graphic intergrowths in some higher-level granitoids are uncommon in metamorphic rocks of equivalent composition, in which solid-state recrystallization/neocrystallization processes dominate [Vernon & Paterson, 2008c]. In particular, graphic intergrowths (Figure 41), with relatively high-energy grain-boundary configurations, in many high-level granitoids show no evidence of recrystallization to form the polygonal aggregates typically produced where such intergrowths are recrystallized [e.g., Vernon, 2004, pp. 256-257, fig. 4.65].
(4) Contrast with metamorphic microstructures: Vernon and Paterson [2008c] emphasized that the shapes of grains and included grains in granitoids typically do not resemble those of medium- to high-grade metamorphic rocks. For example, inclusions of plagioclase in granitoid minerals are typically euhedral, and can be arranged in crystallographic zones in the host mineral (Figure 18), whereas inclusions of plagioclase in metamorphic minerals are commonly rounded and either random or arranged in trails [e.g., Vernon, 1999, 2004]. The contrast with high-grade metamorphic rocks is due partly to the lack of driving forces for recrystallization, owing to the chemical compatibility of the granitoid mineral assemblage, and especially the absence of sufficient solid-state deformation to promote recrystallization [Vernon and Paterson, 2008c].
I emphasize that the foregoing discussion refers to preservation of primary granitoid microstructures during slow cooling in the absence of solid-state deformation or metamorphism, which can induce recrystallization, involving processes such as grain-boundary migration and the formation of new grains [e.g., Vernon, 2000a; 2004; Vernon and Clarke, 2008; Vernon et al., 2010].