Origin of the Italian Magmatism
The origin of Italian potassic and ultrapotassic rocks and of their peculiar trace elements and isotopic signatures were the object of a scientific debate centered on two main possible mechanisms: a) within-plate origin, possibly linked to partial melting of an up-rising mantle plume (e.g., Vollmer & Hawkesworth, 1981; Vollmer, 1990; Ayuso et al., 1998; Castorina et al., 2000; Gasperini et al., 2002; Bell et al., 2004); b) orogenic to post-orogenic origin, related to partial melting of the sub-continental lithospheric metasomatised mantle at a destructive plate margin, with important contributions from recycled sediments (e.g., Cox et al., 1976; Peccerillo, 1985, 2005a; Rogers et al., 1986; Beccaluva et al., 1991; Conticelli & Peccerillo, 1992; Downes et al., 2002; Bianchini et al., 2008).
Some of the authors suggesting the within-plate origin also point out the need for an increasing influence of an upper crustal geochemical component northward, although no convincing reasons have been provided to explain it in the frame of a within-plate, extensional geodynamic setting (e.g., Hawkesworth & Vollmer, 1979; Vollmer, 1990; Gasperini et al., 2002; Bell et al., 2004). In the anorogenic ‘plume-related’ model the crustal signature is generally attributed to shallow level magma contamination processes. The high extent of shallow level crustal contamination required to reproduce the Italian ultrapotassic magmas (> 70 vol. % to gives the lamproitic isotopic signatures) seems unlikely to occur at shallow level without suffering extensive crystallization of mafic phases. This would have decisely decreased the MgO, Ni, and Cr contents of the mafic rocks bringing them on the differentiated felsic side (Conticelli, 1998; Turner et al., 1999; Murphy et al., 2002). On the contrary, the most ultrapotassic rocks from each magmatic province (i.e. lamproites, kamafugites and lucitites), which also display the highest crustal signature, are the ones bearing the highest abundances in MgO and compatible trace elements (Tables 2-8). In addition these rocks have liquidus highly forsteritic olivine, which does show textural characteristics typical of extra olivine from cumulus processes. In addition, a proportion of assimilated crust higher than 40 vol. % would rapidly freeze the magma, stalling its ascent and causing it to crystallise completely. In summary, even a small amount of crustal contamination would result in rapid crystallization and fractionation of mafic minerals. MgO, FeO, Ni, Sc, and Cr contents would dramatic decrease in the contaminated magma with no further enrichment in K2O (Conticelli, 1998; Turner et al., 1999; Murphy et al., 2002).
Avanzinelli et al. (2008) recently reported a comprehensive study of U-Th disequilibria on the youngest Italian rocks with the aim of investigating the mechanism of source melting. The authors excluded the possibility of the plume-related genesis, in particular of a plume upwelling from a slab window located under the Neapolitan area, on the strength of significant 238U-excess measured in the volcanic rocks of the Neapolitan district of the Roman Province. In fact, 238U-excess is widely accepted as one of the key evidence of subduction-related magma genesis (e.g., Elliott et al., 1997; Hawkesworth et al., 1997). The occurrence of such excesses only in Neapolitan rocks is ascribed to the recent addition of a U-rich fluid component, which is also expected to act as a trigger for magmatism. The lack of any significant disequilibria in the Latian districts (Roman Magmatic Province) and Amiata rocks (Tuscan Magmatic Province) is also inconsistent with adiabatic melting of a deep-seated mantle plume, which would produce significant 230Th excesses (Avanzinelli et al., 2008). On the other hand, significant 230Th- excesses, an indicator of adiabatic melting, have only been measured in a sample from the Monticchio lakes volcanism, in the Lucanian Magmatic Province (Avanzinelli et al., 2008, 2010).
Subduction-related metasomatism
The isotopic charateristics discussed above matches well with the incompatible trace element distributions ubiquitously shown by the volcanic rocks of each magmatic province. It is generally thought that fractionation of High Field Strength Elements (HFSE) with respect to Large Ion Lithophile Elements (LILE) is a typical characteristic beared by orogenic magmatic suites (e.g., Peccerillo, 1985; Hofmann, 1996, Hoefs, 2010) and that Pb peaks are generated by sediment recycling within the upper mantle (Avanzinelli et al., 2008). The relative immobility in aqueous fluids of incompatible high field strength elements and Th with respect to large ion lithophile ones has long been used to distinguish between the roles of fluids and melts during metasomatism (e.g., Hawkesworth et al., 1990; Keppler, 1996; Elliott et al., 1997; Elliott, 2003; Kessel et al., 2005). Conversely, the budget of other trace elements such as Th, is controlled in arc rocks by sediment recycling (e.g., Elliott et al., 1997; Plank & Langmuir, 1998; Tommasini et al., 2010). High Th concentrations, Th/Nb and Th/REE ratios in subduction-related volcanic rocks are interpreted as representative of recycled sediments. The comparison of these ratios with subducted sediments have suggested that the latter are recycled as melts (e.g., Elliott et al., 1997; Hawkesworth et al., 1997; Class et al., 2000; Elliott, 2003; Plank, 2005) enriched in Th and incompatible trace elements, but still depleted in HFSE, probably due to the occurrence of residual rutile during sediment partial melting (e.g., Elliott et al., 1997; Tommasini et al., 2007).
At sub-arc temperatures and pressures the physical distinction between fluids and melts is lost above the ‘second critical end point of saturation’ (e.g., Kessel et al., 2005; Hermann et al., 2006) where they converge to a ‘supercritical liquid’, but ‘fluid’-like and ‘melt’-like liquids can still be distinguished using the relative proportion of H2O and solute contents, depending upon the T°C of ‘supercritical liquid’ formation (e.g., Hermann et al., 2006). Klimm et al. (2008) showed that the trace element budget of metasomatic agents released by the basaltic subducting crust at at 2.5 GPa, regardless of whether they are ‘fluids’ or ‘supercritical liquids’, depends upon the solubility of accessory phases such as allanite and monazite (controlling REE and Th and U) and rutile (determining the Ti and Nb contents). At normal slab/mantle interface temperatures these accessory phases are stabilised in the residuum during slab melting, strongly sequestering REE, Th, (in allanite and/or monazite) and Ti and Nb (in rutile) from the metasomatic liquids. The resulting metasomatic liquid is enriched only in the elements not hosted in the residual accessory phases (Cs, Rb, Ba, K, Pb, Sr and U over Th), perfectly reflecting the widely accepted composition of the slab derived aqueous ‘fluids’. With increasing temperature or/and pressure the metasomatic liquids incorporate higher amounts of solutes (i.e. ‘melts’), and the solubility of allanite, monazite and rutile increases until they are eventually eliminated from the residue with Th, and REE released into the melts (Hermann, 2002; Kessel et al., 2005; Klimm et al., 2008). However, until over-saturated, allanite and rutile will completely control the REE, Th, U (allanite) and Ti, Nb (rutile) content of the sedimentary ‘melts’. Similar conclusions have been reached by Skora & Blundy (2010) starting from radiolarian clay-rich sediments.
Allanite and monazite have partition coefficients for LREE and Th extremely higher than those for heavy REE (HREE) and U; thus allanite-saturated ‘melts’ from recycled pelitic sediments would have lower LREE/HREE and higher U/Th than their sedimentary protolith. On the contrary if temperatures are high enough keep the metasomatic liquids undersaturated in such minerals, then the Th, U and REE can be released in the liquids and their trace elements contents and ratios would be dependent on other mineral phases. The high Th/U and LREE/HREE of most Italian rocks (with the exception of the Neapolitan district) suggest that the dominant metasomatic agent is a ‘melt’ generated at temperatures where rutile was stable is still rutile-saturated, hence the HFSE depletion, but allanite and monazite were not, hence the high Th/U (Avanzinelli et al., 2009). In this frame the high LREE/HREE of most of Italian ultrapotassic and associated shoshonitic rocks (Figs. 8, 10, 12, 14, 16, 24, 26, and 28) has been ascribed to the critical role of garnet during sediment melting (Avanzinelli et al., 2008). This is consistent with the high amount of residual garnet observed during experiments of melting of both basaltic (Kessel et al., 2005) and pelitic or carbonate rich (Kerrick & Connolly, 2005) sediments. The possibility of acquiring the garnet signature during by deep melting in the garnet stability filed (i.e. metasomatised mantle source deep enough to stabilise garnet) was ruled out by Avanzinelli et al. (2009) on the basis of the lack 230Th excess (see also Asmerom, 1999).
Figure 41. Ba/Th vs. Th/Nb for the Italian ultrapotassic rocks.
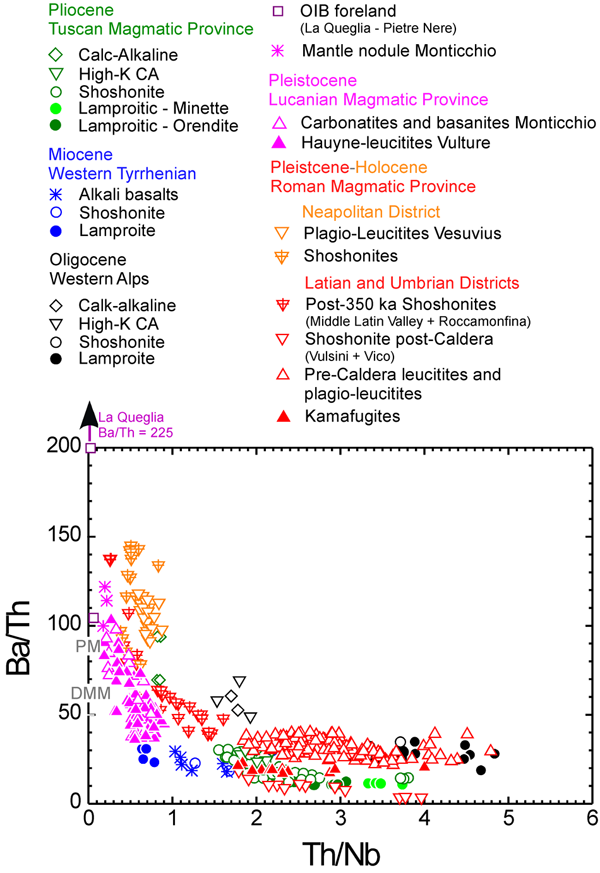
Plotted rocks with MgO > 4.5 wt. %. Data from Conticelli et al. (1987, 1991, 1997, 2002, 2007, 2009a, 2009b, 2010c, 2010d), Ayuso et al. (1998), Conticelli (1998), Pappalardo et al. (1999, 2002), D’Antonio et al. (1999b), Perini et al. (2000, 2003, 2004), Downes et al. (2002), Cadeaux et al. (2005), De Astis et al. (2006), Peccerillo & Martinotti (2006), Di Rienzo et al. (2007), D’Orazio et al. (2007, 2008), Owen (2007), Prelevic et al. (2008), Stoppa et al. (2008), Avanzinelli et al. (2008, 2010), Boari et al. (2009a,b), Cadeaux & Pinti (2009), Melluso’s unpublished data; Tommasini’s unpublished data.
The dominiant role of sediment derived ‘melts’ does not exclude the involvement of ‘fluids’ generated at lower temperature, but simply dimishes their possible effect on the final composition of the metasomatised mantle source, given their smaller ability of carrying trace elements with respect to ‘melts’ (Hermann et al., 2006). In fact a widespread fluid-like component might have permeated the mantle source of Italian magmas in a more pervasive way than the melt-like metasomatism that generates the most enriched ultrapotassic products (see later discussion section).
The role of metasomatic ‘fluids’ vs. ‘melts’ is evident in a Ba/Th vs Th/Nb plot (Fig. 41). Negative correlation is ubiquitous, but the various magmatic provinces define different trends. The widest range is shown by the volcanic rock of the Roman Magmatic Province, where a decrese in Th/Nb, coupled with increasing Ba/Th, is evident passing from the oldest and northernmost districts (i.e. Latian districts) to the youngest and southernmost one, which is the Neapolitan district. Leucite-free (i.e., shoshonite) volcanic rocks from the post-caldera and the most recent periods of the Roccamonfina and Middle Latin Valley volcanoes, the two southermost of the Latian districts of the Roman Province (above the 41st parallel; Figs. 1 and 11), show Th/Nb vs Ba/Th values that ideally fill the gap. The occurrence of significant 238U-excess in volcanic rocks from the Neapolitan distric (Avanzinelli et al., 2008) confirms a major role for a young (few ka, see Avanzinelli et al., 2008) fluid-like metasomatic component (i.e. allanite/monazite saturated); the position of the youngest products of Roccamonfina and Middle latin valley in Fig. 40, along with their vicinity in space and time with the Neapolitan districts might indicate a role for such a fluid component also in these magmas.
An increase of Ba/Th is also observed passing from ultrapotassic rocks (lamproites and minettes) to associated shoshonites and high-K calc-alkaline rocks within the products of the Tuscan Magmatic Province and Western Alps). As discussed above, this might suggest the presence of a pervasive fluid related metasomatism which is overshadowed by the recycled sediment melt component which dominates the geochemical composition of the most enriched magmas (see next section). The opposite occurs in the Western Tyrrhenian (i.e. Crosica province). Ultrapotassic magmas from Sisco (Corsica) display low Th/Nb and Ba/Th values (Fig. 41), with the latter even lower than upper mantle values (PM = 82.2, DMM = 48-60; Wood, 1979; Sun & McDonough, 1989), with little HFSE/LILE fractionation and lack of Pb peak (Fig. 10c). These characteristics suggest that the orogenic component in Corsica was significantly different than that responsible for the other provinces, less important or possibly even absent (Avanzinelli, 2009; Conticelli et al., 2009a, 2010c).
In summary Italian ultrapotassic rocks, both leucite-free and –bearing, with the exclusion of the Sisco one (Corsica), were generated in a lithospheric upper mantle enriched in K and incompatible elements by pelitic sediments recycled mostly as ‘melts’ (e.g., Conticelli et al., 2007, 2009a; Avanzinelli et al., 2008, 2009).
Further issue to be addressed are: i) the time-related and geographic transition from Tuscan lamproite-like ultrapotassic magmas (silica-saturated, leucite-free) to Roman kamafugitic/leucititic-type ones (silica-understaurated, leucite-bearing); ii) the temporal and geochemical sequence from ultrapotassic products to shoshonites and high-K calc-alkaline rocks within each magmatic province.
Transition from leucite-free to leucite-bearing ultrapotassic rocks in the Italian peninsula
The shift from silica-saturated to silica-undersaturated (i.e., from leucite-free to leucite-bearing) ultrapotassic magmas with time is not only a compositional characteristic that controls the final mineralogical assemblages of the volcanic rocks, but it also seems to have a link with the rate of ultrapotassic magma production. Indeed in the Western Alps, Western Tyrrhenian Sea, and Tuscan Magmatic Provinces ultrapotassic rocks are strongly subordinate to shoshonitic mafic rocks and their derivative magmatic terms, whereas in the Roman Magmatic Province leucite-bearing ultrapotassic magmas dominate volumetrically over final shoshonites, which are confined to final stage activity and do not occur in all volcanic districts.
Silica-saturated lamproitic ultrapotassic magmas (leucite-free) are generated within a metasomatised lithospheric refractory upper mantle through incongruent melting of a phlogopite-bearing harzburgitic source under excess of H2O, then low XCO2 (Foley, 1994). Experimental studies have shown that silica-rich lamproitic magmas are generated in a lithospheric mantle source originally depleted in the basaltic component and subsequently refertilised by metasomatism in a phlogopite-orthopyroxene-olivine paragenesis (e.g., Arima & Edgar, 1983a; Edgar, 1987; Foley, 1992b, 1994). Interaction with granitic melts from pelitic sediments is capable to stabilize modal phlogopite in a peridotitic mantle wedge (Sekine & Willie, 1982a, b, 1983; Wyllie & Sekine, 1982). Such metasomatic enrichment through melts from recycled pelitic sediments is also able to explain the higher Al2O3 content of ultrapotassic Italian magmatic rocks with respect to within-plate ones. Conticelli et al. (2007) have modelled for the source of Tuscan lamproite, the amount of recycled crustal component needed to produce the observed Sr, Nd, Pb, and Os isotopic characteristics (Fig. 40). These authors have estimated about 65 vol. % of a crustal-derived component, introduced as melts within the lithospheric depleted mantle. The partial melting of pelitic sediments at relatively high-pressure, within the stability field of garnet for pelitic sediments, following the model of Johnson & Plank (1999) gives a melt consistently enriched in SiO2, Al2O3, and K2O, and depleted in MgO, and FeO, but with an mg-# within the range of 0.85 and 0.80. The composition of this mixture is compatible with a mineralogy dominated by phlogopite/K-feldspar, with minor orthopyroxene and olivine, and possibly no clinopyroxene, as also shown by melting experiments on lamproitic rocks (e.g., Edgar, 1987; Foley, 1994, and references therein). Such a high proportion of metasomatic component is unrealistic to have pervasively patently modified the entire mantle wedge, but this might just represents the core of a peridotite-modified veinlet (Foley, 1992b). Indeed “melts” flowing through the lithospheric peridotite react with the wallrock and then freezing down in a newly formed metasomatized paragenesis, with most of the surrounding mantle unaffected by this reaction (Foley, 1992b; Perini et al., 2004; Conticelli et al. 2007; Avanzinelli et al., 2009). Then upper mantle will only be metasomatised along the main melt pathways thus generating a network of metasomatised veins surrounded by unreacted mantle (e.g., Suen & Frey, 1987; Menzies et al., 1991; Foley 1992b).
Silica-undersaturated kamafugitic to leucititic ultrapotassic magmas (leucite-bearing) are conversely generated in a metasomatised upper mantle by partial melting of a phlogopite-bearing wherlite controlled by a CO2 excess with respect to H2O, then high XCO2 (e.g., Lloyd et al., 1985; Edgar, 1987 and references thereins). Considering that the mantle beneath the Italian region is particularly depleted in the basaltic component (prior to metasomatism) as evidenced by the major and trace element contents of most primitive ultrapotassic magmas (e.g., lamproites, kamafugites and leucitites) and their olivine-spinel pairs compositions (Conticelli et al., 2007, and references therein), a refertilization to produce a sub-lithospheric mantle source with modal clinopyroxene and phlogopite is needed (e.g., Edgar et al., 1980; Arima & Edgar, 1983a; Lloyd et al., 1985; Edgar, 1987 and refences thereins). Thus CaO-enrichment is required beside K and incompatible elements, as well as a source for CO2. Such enriching agent might be consistent with the addition of CaCO3 from the recycled sediments (Thomsen & Schmidt, 2008a; Franzolin et al., 2009; Poli et al., 2009). Reaction of such agent with depleted peridotite generates a re-fertilised phlogopite-bearing wehrlite or phlogopite-bearing lherzolite, with phlogopite and clinopyroxene formed at expenses of olivine and orthopyroxene within the metasomatised vein network. Partial melting of such metasomatised veins at relatively low pressure and high XCO2 is able to produce strongly silica-undersaturated ultrapotassic magmas, from kamafugitic to leucititic in composition (e.g., Wendlandt & Eggler 1980a, b). Arguments for the genesis of Italian kamafugites through the melting of a re-fertilised sub-continental lithospheric mantle at low pressure and under high XCO2 have been shown experimentally (Conticelli et al., 1989). In addition, evidence for the occurrence of CO2-mantle degassing beneath the Italian peninsula has been shown on the basis of geophysical data by Frezzotti et al. (2009, 2010, this volume).
Such a scenario also agrees with the trace element and isotopic distribution of Roman kamafugites and leucitites. Indeed the shift from leucite-free to leucite-bearing ultrapotassic rocks in Italy has been related with differences in the initial composition of the recycled sediments on the basis of isotope and trace element compositions of mafic terms. Conticelli et al. (2002) suggested that the decoupling of Sr and Nd isotopes between Tuscan and Roman magmatic provinces (Figs. 36-37) was caused by the change of the recycled sediment from pelitic to marly. Avanzinelli et al. (2008) suggested a southward increase of the CaCO3 sedimentary fraction related to the composition of the pelitic sediment cropping out along the Apennine chain. Avanzinelli et al. (2009) suggested an increasing carbonate-rich sedimentary component on the basis of 87Sr/86Sri and trace element variations observed along the Italian peninsula. Indeed a negative correlation of 87Sr/86Sri vs. Sr/Ce and Sr/Rb is observed (Fig. 42) and it can also explain the disappearance of the negative Sr anomaly passing from mafic ultrapotassic rocks of Western Alps and Tuscan Magmatic Provinces to Roman mafic rocks (Figs. 8, 10, 12 vs. Figs 16, 18, 20, 22, 24, etc.). Sr and Ce are little fractionated when sediments are recycled as ‘melts’, although they are if the sediment is recycled as ‘fluid’; Sr and Rb are both easily carried by fluids, but might be slightly fractionated during sediment or mantle melting. Therefore the combined use of Sr/Ce and Sr/Rb ratio should provide information about the original composition of the sediment end-member (i.e., carbonate- vs. clay-rich). In figure 42 the carbonate-rich component, characerised by high Sr/Ce and Sr/Rb, increases southward, with leucite-free rocks from the Tuscan Magmatic Province and Western alps plotting at low Sr/Rb and Sr/Ce close to the composition of clay-rich pelitic sediments. In the 87Sr/86Sr vs Sr/Ce diagram (Fig. 42) ultrapotassic rocks, from leucite-free lamproites to leucite-bearing kamafugites and plagio-leucitites, plot over and along the array connecting pelitic sediments to limestone. Rocks from the Lucanian Magmatic Province plot away from that array close to OIB-like compositions (Fig. 41). It is worth noting that Western Tyrrhenian (i.e. Corsica) rocks lie at mid way between Western Alps and Tuscan lamproites and the field of sedimentary compositions. Neapolitan district rocks and post-cladera shoshonites from Middle Latin valley and Roccamonfina also plot at lower 87Sr/86Sr. This is consistent with the recent addition of a further component, as previously discussed to explain the increase of Ba and U contents in the same rocks (Fig. 41) and the 238U-excess reported for the Neapolitan district (Avanzineli et al., 2008).
Figure 42. Sr/Ce and Rb/Sr vs. 87Sr/86Sri for the Italian ultrapotassic rocks.
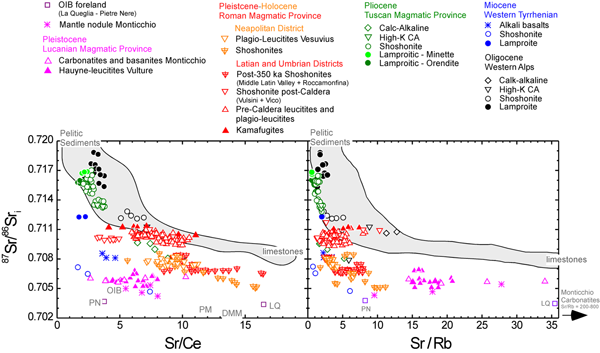
Plotted rocks with MgO > 4.5 wt. %. Data from Conticelli et al. (1987, 1991, 1997, 2002, 2007, 2009a, 2009b, 2010c, 2010d), Ayuso et al. (1998), Conticelli (1998), Pappalardo et al. (1999, 2002), D’Antonio et al. (1999b), Perini et al. (2000, 2003, 2004), Downes et al. (2002), Cadeaux et al. (2005), De Astis et al. (2006), Peccerillo & Martinotti (2006), Di Rienzo et al. (2007), D’Orazio et al. (2007, 2008), Owen (2007), Prelevic et al. (2008), Avanzinelli et al. (2008, 2010), Boari et al. (2009a,b), Cadeaux & Pinti (2009), Melluso’s unpublished data; Tommasini’s unpublished data.
The need for carbonate-rich sediment recycled as allanite-undersaturated melts in the source of the investigated magmas might provide some constraints on the condition of sediment melting. Carbonates are expected to behave as refractory phases at sub-arc depths (up to 180 km: Yaxley & Green 1994; Molina & Poli, 2000; Schmidt et al., 2004). Recent experimental studies (Thomsen & Schmidt, 2008a, b; Poli et al., 2009) have demonstrated that carbonate-saturated pelites (i.e. marls) might produce potassic granite or phonolite melts at temperatures from 900 °C, at 2.4 GPa, to 1070 °C, at 5.0 GPa. As already previously shown, when these melts are mixed with depleted mantle wedge they might represent suitable metasomatic agents for producing phlogopite-bearing wherlite/peridotite from which kamafugitic magma is produced by partial melting. Allanite and monazite solubility provides similar temperature constraints: in the same pressure range (2.5-4 GPa) the temperature required to exhaust allanite are >1000 °C (Hermann, 2002; Klimm et al., 2008); admittedly this value could represent an over-estimate, given the doped composition of the experiments and the basaltic starting sediment composition; experiments on clay-rich sediments showed residual monazite up till 900°C (Skora & Blundy, 2010), whilst no studies are available for carbonate-rich sediment lithologies. The temperature range for sediment melting (900-1100°C) indicated by the geochemistry of the studied rocks is much higher than that estimated at the slab/mantle interface by thermal models of both ‘cold’ and ‘warm’ subduction (e.g., Peacock, 2003: Kelemen et al., 2003). To explain this apparent inconsistency we suggest two possible scenarios; i) an increase in temperature of the slab/mantle interface due to locking of the subduction zone following continental collision; ii) the physical incorporation of portions of the subducted sediments from the top slab into the mantle, either by imbrication or via diapirs (e.g., Kelemen et al., 2003; Klimm et al., 2008), and their melting in the hot central region of the mantle wedge.
It is worth to note, however, that a recent study by Tommasini et al. (2011) indicates a more complex mechanism for the metasomatic enrichment of the mantle-source of Mediterranean lamproitic magmas. These authors have found that a multiple metasomatism event is needed to constrain the extreme enrichment in K and concomitant fractionation of Th and Sm with respect to La. This emerges from a widespread positive Th/La vs Sm/La correlation found in Tethyan Realm lamproites (including those from TMP, Corsica and Western Alps discussed in this study) and associated shoshonitic rocks (Tommasini et al., 2010; Conticelli et al., 2010b), which is opposite to what observed in subduction related magmas worldwide (Plank, 2005).
From ultrapotassic to potassic and sub-alkaline volcanic rocks.
Western Mediterranean ultrapotassic rocks are intimately associated in space and time to shoshonite and in some cases to high-K calc-alkaline suites: this association occurs in the Western Alps, Tuscan and Roman Provinces (Central Italy), but also in Murcia-Almeria (south-eastern Spain), Macedonia, Serbia, Montenegro, and Turkey (e.g., Conticelli et al., 2002, 2007, 2009a; Altherr et al., 2004; Peccerillo, 2004, 2005a; Prelevic et al., 2004, 2005; Avanzinelli et al., 2009). In the Western Alps correlation with time is not clear, also because the analytical error is sometimes greater than the possible time elapsed from one series to the other one. The best evidence of this time-dependent geochemical variation can be observed in the Tuscan and Roman magmatic provinces. In most cases above a single plumbing system, stratigraphy and geochronology testify the succession from either lamproite- or kamafugite-like rocks, to shoshonites and high-K calc-alkaline rocks, through high-K shoshonites or leucitites (e.g., Perini et al., 2004; Conticelli et al., 2007, 2009a, 2009b, 2010d; Frezzotti et al., 2007; Boari et al., 2009b). The opposite is observed at Somma-Vesuvius volcano where plagio-leucititites follow shoshonitic volcanic rocks (Cioni et al., 2008, and references therein), similarly to the succession observed at Stromboli volcano in the Aeolian Arc (e.g., Ellam et al., 1988; Peccerillo, 2001, 2005a; Alagna & Peccerillo, 2010, this volume).
The geochemical transition between magmatic series differently enriched in K and incompatible trace elements (i.e. from ultrapotassic to shoshonites or vice versa) has received at least three explanations: i) relaxation of isotherm that intercepts the solidus of variably metasomatized upper mantle levels, with the most strongly metasomatized mantle at deepest level (Peccerillo, 2005a; Frezzotti et al., 2007); ii) either increase or decrease with time of shallow level carbonate assimilation, the former claimed to explain the Somma-Vesuvius case (Iacono-Marziano et al., 2007, 2008); iii) incremental partial melting of a manle source metasomatized in a veined network and dilution of the vein end-member by interaction with surrounding peridotitic country-rocks (e.g., Foley, 1992b; Conticelli et al., 2002, 2007, 2009a; Perini et al., 2004; Avanzinelli et al., 2009; Boari et al., 2009b).
The first hypothesis fails to explain why earlier magmas, formed at deeper levels, did not interact with uppermost metasomatised levels. In addition, all primitive mafic magmas independently from their K2O contents seem to have been in equilibrium with a mantle source characterized by the same depletion prior to metasomatism (Conticelli et al., 2007). In fact, differences in the mantle source residuality are not observed within the same plumbing system, but rather between Latian and Neapolitan districts. Therefore the sources of ultrapotassic and shoshonitic magmas should not be too distant from each other.
Recently Iacono Marziano et al. (2007, 2008) have called for strong involvement of sedimentary carbonate assimilation in the Italian rocks to justify the derivation of ultrapotassic leucite-bearing rocks from shoshonite. Such a possibility was firstly postulated by several authors in the early twentieth century (e.g., Daly, 1910; Rittmann, 1933) and later discounted by Savelli (1967). Iacono Marziano et al. (2007, 2008), on the basis of experimental data, justify the passage from shoshonite to either leucititic or plagioclase leucititic magmas through limestone assimilation plus clinopyroxene crystallisation. Conticelli et al. (2009b) tackled this issue focusing on leucite-free shoshonites and leucite–bearing plagio-leucitites from Roccamonfina volcano. The authors attempted modelling of AFC processes able to drive the composition of magmas at Roccamonfina volcano from the post-cadera shoshonites to the pre-caldera leucitites and plagioleucitites and vice versa: they demonstrated that AFC processes, although important for the chemical evolution within each magmatic series, could not be responsible for the trasition from ultrapotassic leucite-bearing magmas to leucite-free shoshonites or vice versa. In the specific case of Somma-Vesuvius further evidence against a major role for crustal contamination are reported by Del Moro et al. (2001).
As previously discussed extremely high contribution from recycled crustal material are required in order to generate the most extreme composition of Italian lamproites and kamafugites. It is unlikely for such a high proportion of metasomatic component to pervasively modify the entire mantle wedge, whilst it have been proposed that it might affect only localised portions of the mantle in a network of metasomatic veins (Foley, 1992b). Indeed “melts” flowing through finite path trhough the lithospheric peridotite, react with the surrounding wallrock, freezing down as a newly formed metasomatized paragenesis, leaving most of the surrounding mantle unaffected by this reaction (Foley, 1992b; Perini et al., 2004). The vein hypothesis is also consistent with most of the metasomatized peridotites observed in nature (e.g., Rivalenti et al., 1995, 2007; Zanetti et al., 1999; Rampone et al., 2010). In the veined model, the mantle source reacts differently during upward migration of the isotherms. Initially, partial melting will affect the portion of the mantle with the lowest liquidus temperature, which is the inner portion of the veins characterized by pure metasomatic mineralogy (Foley, 1992b, 1994). The temperature increase, due to post-orogenic isotthrerm re-equilibrium, triggers partial melting also of the surrounding mantle alolowing the interaction between vein and country rock, thus diluting the metasomatised component (Conticelli et al., 2007). Incompatible trace element and isotopic variations observed for the Western Alps, Tuscan and Roman Magmatic Province, with the exception of the Neapolitan district, which requires an additional U-rich ‘fluid’ component (Avanzinelli et al., 2008), can be explained in terms of mixing between a strongly alkaline component, either lamproite for Western Alps and Tuscany leucite-free rocks or kamafugite for Roman leucite-bearing ones, and a probably sub-alkaline end member (i.e., high-K calc-alkaline). Indeed, it has been argued that the surrounding lithospheric mantle bear itself a subalkaline composition, possibly due to a pervasive ‘fluid’-like metasomatism, similar to that of many arc magmas worldwide, as opposed to the localised ‘melt’ metasomatism that give raise to the vein network (Conticelli et al., 2009b; Conticelli et al., 2010b). Passing from lamproites to shoshonites and high-K calc-alkaline rocks, the influence of the vein budget (i.e. high Th/Nb, low Ba/Th) over the composition of the erupted magmas becomes less important in favour of that of the surrounding mantle (Fig. 41). The increase of Ba/Th toward the less enciched terms (i.e. shoshonite and high-k calc-alkaine) suggests indeed that the surrounding mantle has been itself affected by a ‘fluid’-like component enriched in Ba with respect to Th. This pervasive metasomatism can also facilitate melting of the lithospheric mantle surrounding the vein that was deemed as highly depleted by previous basaltic extraction on the base of major elements and olivine-spinel pairs and that would result otherwise refractory.
The process of diluting the vein contribution by involving increasing proportion of the surrounding lithospheric mantle during partial melting produces isotopic mixing trends, which are not distinguishable from simple mixing between two end-member magmas. However, simple magma mixing at shallow level, within the upper crusts has to be ruled out on petrographic grond, since most of the high-MgO rocks can be confidently considered to be very close to primary magmas compositions. Most of the rocks under consideration have olivine on the liquidus, with compositions in equilibrium with the bulk rock. The latter is considered to be the composition of the magma from which olivine crystallises. If magma mixing occurred at shallow levels, olivine would have begun to crystallise and mixing would have altered the composition of the magma driving it away from equilibrium with olivine.
A further possibility is represented by the recent arrival of a fluid-like metasomatic agent that overprinted the old metasomatic signature and trigger partial melting also in the surrounding mantle. This is likely to be the case under the Neapolitan District, where such arrival is testified by the 238U-excesses (Avanzinelli et al., 2008). In general this process should imprint a different geochemical and isotopic signature in the most recent sub-alkaline magmas (e.g., De Astis et al., 2006; Boari et al., 2009b; Conticelli et al., 2009b).
Within-plate component
The presence of this component and its origin is potentially the essence of the debate about plume vs. subduction-related origin of circum-Tyrrhenian magmatism. Namely, primordial mantle normalised incompatible trace elements patterns of the potassic rocks from the Lucanian Magmatic Province are clearly different from those of the other provinces (Fig. 34c). Troughs at Ta, Nb, and Hf as well as LIL vs. HFS elements fractionations are strongly reduced, especially in the most recent products of the Monticchio lakes. Isotopic signature resembles that of within-plate magmas (Figs. 35, 36, and 37) with values close to those of the OIB enriched terms, although a lithospheric orogenic signature appers to be still present in some way (e.g., Downes et al., 2002; De Astis et al., 2006; D’Orazio et al., 2007, 2008). Indeed, Lucanian Province volcanic rocks also display the most radiogenic Pb and Nd and the least radiogenic Sr isotopic compositions with respect to all Western Mediterranean ultrapotassic and associated rocks (see references in Peccerillo, 2005a and Conticelli et al., 2009a). A within-plate mantle component has been proposed to be involved, albeit in different style and extent, in the genesis of the southernmost Roman magmas, namely those of the Neapolitan district (Ellam & Hawkesworth, 1998; Beccaluva et al., 1991; Ayuso et al., 1998; Peccerillo, 2001; Gasperini et al., 2002; De Astis et al., 2006; Bianchini et al., 2008), and at a still minor extent in the final activity of the southernmost Latian districts (Conticelli et al., 2009b).
In the case of Roccamonfina volcano an abrut shift from pre- to post-caldera volcanic rocks is observed, with decrease of alkaline degree and total abundance of incompatible elements with time. All these characteristics might be consistent with the process of increasing interaction proportion of melts from surrounding mantle with respect to vein melts. In this case, however, the vein vs. wall-rock mantle interaction does not explain the large variation in Sr-Nd-Pb isotopes observed (Figs. 36, 37, and 38). Indeed, the expected isotopic composition of the surrounding mantle, which is low Sr and high Nd isotope ratios, respectively, would suite the versus of variation in the shoshonites; however, a depleted mantle is expected to have developed low U/Pb and Th/Pb and thus to have an unradiogenic signature, rather than the radiogenic one necessary to explain the increase in Pb isotopic ratios towards the shoshonites (Fig. 38). Early Roccamonfina ultrapotassic volcanic rocks (leucite-bearing) display Sr-Nd-Pb isotopes well within the range of values of the other Latian districts, whereas the rocks from the final stage of activity display values well within the field of the Neapolitan district and pointing to the isotopic compositions of the Lucanian Magmatic Province (Figs. 36, 37, and 38). The volcanoes of the Neapolitan district show a more abundant within-plate component with respect to Roccamonfina final activity both in terms of isotopic (Figs. 36, 37, and 38) and incompatible trace element compositions (Figs. 30, 31, and 32). To explain this within-plate component in the ultrapotassic and shoshonitic rocks of the Neapolitan district, Beccaluva et al. (1991) suggested the occurrence of a mantle wedge characterized by an OIB signature prior to metasomatism south of the 41st parallel. This possibility however, does not explain i) the occurrence of this component limited to the final stage activity of Roccamonfina, a volcano slightly north of the 41st parallel; ii) the observed geochemical trends from Roccamonfina to Lucanian rocks passing from Neapolitan ones; iii) the absence of the within plate component in the early Roccamonfina rocks; indeed if mantle wedge had an OIB signature prior to the metasomatism, it is conceivable that this signature would be at some extent preserved also within the veins producing early ultrapotassic rocks.