Geodynamic implications
Ultrapotassic and associated shoshonitic to high-K calc-alkaline volcanic rocks of the Italian region have been produced from anomalously enriched upper mantle source following sediment recycling at a denstructive plate boundary. Sediment-derived ‘melts’ represent the dominant metasomatic agent responsible for the establishment of a metasomatic vein network, but a more pervasive ‘fluid’-like metasomatism is also present. The extreme enrichment in Th and the fractionated REE pattern of the erupted rocks suggests the presence of garnet in the residuum during the partial melting of the recycled sediments brought into the mantle through subductionand crustal delamination. This implies that temperatures during sediment partial melting were high enough to exhaust other accessory phases, such as allanite and/or monazite. These temperatures are higher than those generally expected at the slab/mantle interface, indicating that sediment melting occurred in the hot, central regions of the mantle wedge rather than at the slab/mantle interface. Large HFSE negative anomalies and fractionated REE patterns require rutile and garnet in the residuum.
The first episode of magmatism occurred during the Oligocene within the Western Alps, and it was related to the Alpine collision. Lamproite-like ultrapotassic magmas were generated in strict association with shoshonitic, high-K calc-alkalic and possibly calc-alkalic magmas. Magmas were emplaced during the post-collisional stages of the Alpine chain. Peccerillo & Martinotti (2006), provides evidence that the same metasomatic Alpine event occurred also in Corsica, and Tuscany, well before than Apennine orogenic movements started. Reactivation of this old metasomatised should has promoted the partial melting and the formation of Corsica and Tuscan lamproites. Unfortunately, the Sisco lamproite (Corsica) has a within plate signature that dominate over the orogenic ones, whereas the Tuscan lamproitic rocks have a slightly different isotopic signature with respect to the Western Alps (Conticelli et al., 2009a).
During Tortonian, shoshonitic and high-K calc-alkaline rocks were emplaced along the western margin of the Tyrrhenian Sea (Fig. 43) possibly related to a westward dipping Apennine subduction (e.g., Faccenna et al., 1997; Jolivet et al., 1998; Rosembaum et al., 2002, 2004, 2008; Rosembaum & Lister, 2004). Backward migration of the subducted slab and consequent isotherm relaxation triggered the generation of lamproitic magmatism in the Italian peninsula after the beginning of continental collision. Shoshonites and high-K calc-alkaline rocks followed the eruption of lamproites (Fig. 43) as a consequence of the increasing heat flow that brought to melt larger portion of the mantle wedge, with surrounding mantle becoming dominant with respect to metasomatised veins. Magmatism progressively moved eastward.
The metasomatic event was possibly not generated during the Apennine subduction but through several events of subduction of the Tethys Ocean beneath the European plate (i.e., Tommasini et al., 2010). During the upper Pliocene to lower Pleistocene the lamprotitic to shoshonitic magmatism furtherly migrated eastward with an arcuate distribution immediately back to the Apennine chain where post-orogenic extension started to produce NW-SE elongate basins (Fig. 43b).
After a hiatus of several hundred thousand years, during the Middle Pleistocene (Fig. 43c), magmatism shifted its composition from silica saturated and leucite-free to silica-undersaturated, with formation of kamafugitic to plagioclase leucititic and leucititic magmas, which were erupted along the same plumbing system used by the late Tuscan magmas. In some cases hybridism between Roman and Tuscan magmas took place during the early phases of the Roman volcanism (e.g., Palaeo-Bolsena, Rio Ferriera formation at Vico, Morlupo volcanic rocks at Sabatian district), in other cases reactivated old Tuscan crystallising magmatic reservoirs bringing hybrid rocks to form the Amiata volcano.
The newly arrived magma was silica undersaturated and was generated in response of the recycling of carbonate-rich pelitic sediments within the mantle wedge during last collisional phases. This carbonate-rich metasomatism produced phlogopite-bearing wehrlitic veins, which partial melting under high XCO2 generated kamafugites and leucitites. A further eastward migration of magmatism occurred at the passage from Middle to Upper Pleistocene with the formation of kamafugitic magmatism in intra-apennine area (Fig. 43c).
Figure 43. Schematic tectonic evolution of the circum-Tyrrhenian area since late Miocene.
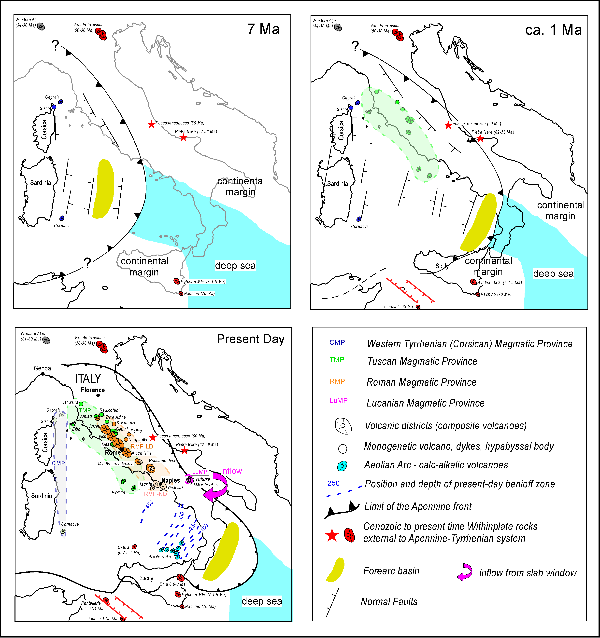
CMP = Corsica Magmatic Province; TMP = Tuscan Magmatic Province; RMP-LD = Roman Magmatic Province – Latian Districts; RMP-ND = Roman Magmatic Province, Neapolitan District; LuMP = Lucanian Magmatic Province; AA = Aeolian Arc Magmatic Province. Redrawn after Faccenna et al. (2004), Cifelli et al. (2007), and Avanzinelli et al. (2009).
During the late Pleistocene asthenospheric mantle from the foreland start to inflow within the mantle wedge through a slab-tear located close to the Bradanic trhough.located close to the bradanic through. Trace element ratios and isotopic values are consistent with the involvement of a within-plate mantle component similar to that of the asthenospheric mantle of the foreland, the Adria microplate. The Lucanian Magmatic Province with the nested Vulture and Monticchio volcanoes is located at the very extreme edge of the overriding plate (Fig. 11), where the mantle wedge is reduced or absent. Toroidal inflow of hot asthenospheric material into the mantle wedge will produce partial melting of the convecting mantle, which interacts with the small amount of metasomatised mantle wedge peridotite as recorded by Downes et al. (2002). This would results in magmatic rocks with intermediate geochemical characteristics between orogenic and within-plate. Inflow is favoured by the south-eastward roll back and progressive fragmentation (i.e., detachment: Wortel & Spakman, 2000) of the subducting plate which is now reduced and segmented into narrow tongues from one original slab (Faccenna et al., 2004; Rosembaum & Lister, 2004; Mattei et al., 2007; Rosembaum et al., 2008). The asthenosperic mantle flow through the tear within the subducted slab in correspondence of the Vulture volcano (Wortel & Spakman, 2000), with time moved into the southern Italian mantle wedge. The motion of the asthenospheric mantle from the foreland within the mantle wedge is recorded by the less evident within-plate signature observed from volcanoes of the Neapolitan district (e.g., Peccerillo, 2001; De Astis et al., 2006), and at a less extent by the sub-alkaline recent volcanic rocks of the Roccamonfina, and even lesser extent of the Middle Latin Valley (Boari et al., 2009b; Conticelli et al., 2009b).
The location of the main tears are controlled by the presence of lateral heterogeneities in the subducting slab such as those represented by the transition from the Ionian oceanic lithosphere to the Apulia and Hyblean continental lithosphere. Such heterogeneities are responsible for the curvature of the Calabrian Arc and for the major fragmentation of the subducting plate. In the Vulture volcano area, the presence of a lateral tear in the subducting slab is suggested by the differing behaviours of the foreland areas southeast and northwest of the volcano. These areas correspond to the transition from the thick carbonate Mesozoic succession of the Apulian platform, which itself corresponds to the buoyant outcropping Apulia foreland, to the transitional and basinal facies outcropping in the Gargano area. The beginning of magmatism at Vulture volcano marks the vertical rupture of the subducted plate. In Lucanian Magmatic Province this scenario is also supported by a 230Th-excess measured in a sample from the Monticchio Lake maars, suggesting an important role for adiabatic melting in an up-welling asthenospheric mantle source (Avanzinelli et al., 2008, 2010).
On the basis of the time of occurrence of the within-plate component we might suggest that the slab-tear in correspondance of the Vulture volcano formed before the middle Pleistocene, indeed the early Vulture volcanic products, which are as old as 0.7 Ma, show the presence of the within-plate component. However, the slab-tear opening northward occurred significantly later. Indeed the ultrapotassic magmatism during the Middle Pleistocene at Roccamonfina and Middle Latin Valley volcanoes was not affectetd by this component. The within plate component appears in these volcanoes at about 035 Ma. Since then the within-plate component invaded the Neapolitan mantle wedge.
Eventually, during the Holocene a further metasomatic agent arrived in the Neapolitan region to account the U-Th isotopic characteristics of Vesuvius, Ischia and Campi Flegrei magmas (Avanzinelli et al., 2008). Indeed the U-Th disequilibria require the addition, shortly before the eruption of the magmas of the Neapolitan district (<10ka), of a U-rich component, which has affected neither the Latian district of the Roman Magmatic Province nor the Monticchio lakes volcanic products of the Lucanian Magmatic Province (Avanzinelli et al., 2008).