Explanatory notes of the structural model of Italy
The Alpine chain and its tectonic and lithological features are represented in the sheets 1, 2 and 3 of the Structural Model of Italy, scale 1:500,000 (Bigi et al., 1990, 1993), printed by SELCA, Firenze. Sheet 1 represents two-thirds of the Alps, from the western arc to the middle of the Tauern window.
Figure 24. Structural Model of Italy.
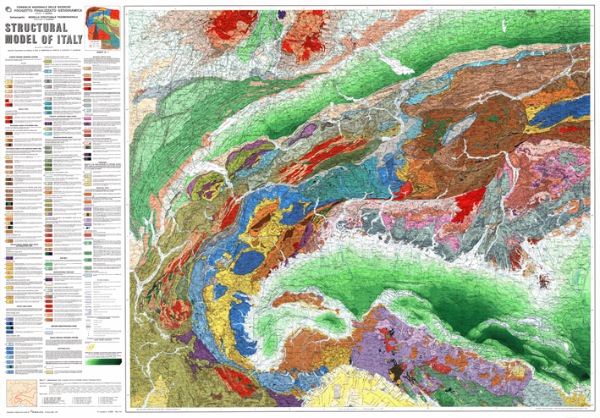
Sheet 1 of the Structural Model of Italy, scale 1:500,000 (Bigi et al., 1990), extending from the Western and Central Alps to the middle of the Eastern Alps (Tauern window). The entire model can be seen in the site e-Geo (http://www.egeo.unisi.it/).
Sheet 2 shows the easternmost Austrian and Slovenian part of the belt, up to the Neogene Vienna and Styria (western Pannonian) basins, including the famous high-P Saualpe-Koralpe-Pohorje basement units and the most eastern ophiolitic occurrence inside the Rechnitz window. Sheet 3 comprises only a minimal part of the belt, i.e. the south-easthern edge of the French-Italian arc, from the Penninic Helmintoid flysh and Subalpine chains to the foreland basement and cover units of Provence. These maps can be seen in the site e-Geo (http://www.egeo.unisi.it/), and a low-resolution image of sheet 1 is included here as Figure 24.
The Structural Model of Italy was the central task of the Progetto Finalizzato Geodinamica promoted by the Italian Research Council (CNR). It constituted the most important and fruitful integration of scientists with geological and geophysical background towards a three-dimensional reconstruction of the anatomy of Italy and its geodynamic evolution, supported by Antonio Praturlon and led by Paolo Scandone and Carlo Morelli. Focusing on Alpine maps, I have drown the entire Europe-vergent chain, by generalizing regional and local maps at various scales, with contributions of Messiga (Voltri Group), Vanossi (Ligurian Briançonnais), Pognante and Polino (Lanzo and Susa ophiolites), Bigioggero, Montrasio, Notarpietro and Potenza (Ossola-Valtellina), Gatto and Gregnanin (Venosta valley-Brenner Pass), Rybach (isobaths of Molasse foredeep), Plöchinger (Northern Calcareous Alps), Beck-Mannagetta (northern Grauwackenzone), Austrian Oil Agency (isobaths of Austrian basins). The Adria-vergent Southern Alps were compiled by the Southalpine working group coordinated by Castellarin and Vai, and the Po plain subsurface features by AGIP Mineraria. The elaboration of these maps was an exciting adventure, but it was a long and exhausting work: the enthusiasm and forces of coordinators and authors ran out, so that the maps were printed without the explanatory notes. This is probably the principal reason because the Structural Model is poorly mentioned. I warmly recommend the use of these maps that can facilitate the first approach of students and non-specialist readers toward the Alpine geology, together with special issues and regional overviews, concerning for instance the French-Italian Alps (Roure et al., 1990; Dal Piaz, 1992, 1999; Vanossi, 1992; Michard et al., 1996; Beltrando et al., 2010; Compagnoni et al., this volume), Switzerland (Trümpy et al., 1980; Pfiffner et al., 1997), Austria (Flügel & Faupls, 1987; Neubauer, 1992; Plöchinger, 1995; Kurz et al., 1998b; Neubauer & Höck, 2000), Southern Alps (Bertotti et al., 1993; Castellarin et al., 1993, 2006; Cita & Forcella, 1998; Castellarin & Cantelli, 2000; Vai et al., 2002; Doglioni & Carminati, 2008; Gaetani, this volume), tectonics (Coward et al., 1989, Ratsbacher et al., 1991; Schmid et al., 2004), paleostructural restorations and pre-Mesozoic geology (von Raumer & Neubauer, 1993; Schmid et al., 2008; Stampfli & Hochard, 2009), metamorphic features (Frey et al., 1999; Oberhänsli et al., 2004) and geochronology (Hunziker et al., 1992; Rubatto et al., 1998; Gebauer, 1999; Thöni, 1999).
Geological outline of the Alps
According to the sense of tectonic transport toward the foreland, the Alps are currently subdivided into two belts of differing size, internal frame, age and geological meaning: 1) the Europe-vergent belt, a thick collisional wedge of Cretaceous-Neogene age, consisting of continental and minor oceanic units radially displaced towards the Molasse foredeep and European foreland; 2) the Adria-vergent Southern Alps, a minor, shallower (non-metamorphic), ophiolite-free and younger (Neogene) thrust-and-fold belt displaced southwards to the Padane plain, which developed inside the previous Alpine hinterland (retro-wedge) of the Adriatic upper plate, far from the oceanic suture. These antithetical belts are juxtaposed along the Periadriatic (Insubric) lineament, a major fault system generated or re-activated during Oligo-Neogene times.
This structural reconstruction is synthetized in Fig. 25, a tectonic map of the Alps and Northern Apennine directly inferred from the Alpine sheets of the Structural Model of Italy, showing the main structural domains and thrust planes. The map covers nearly the same area of the satellite image in Fig. 1, including the Po plain and Northern Apennine. From top to bottom and from the inner to the outer side, the principal Europe-vergent tectonic domains are: i) the Austroalpine multi-nappe and polyphase system, derived from the Adriatic (s.l.) continental margin, which mainly developed during the Cretaceous (eoalpine orogeny); ii) the Penninic zone, a stack of metamorphic nappes scraped off the subducting oceanic lithosphere, European passive margin and ribbon continents, characterized by Tertiary high-P metamorphism and mainly accreted during the Paleogene; its outer boundary is the Penninic frontal thrust; iii) the Helvetic zone, consisting of shallower basement slices and decollement cover units derived from the European margin after the closure of the Valais basin, mainly imbricated from the Late Oligocene onwards. The stacking order of nappes and the age of metamorphic events and related deformations are tought to be representative, with caution and generally, of the outward propagation of the orogenic wave.
Figure 25. Tectonic map of the Alps.
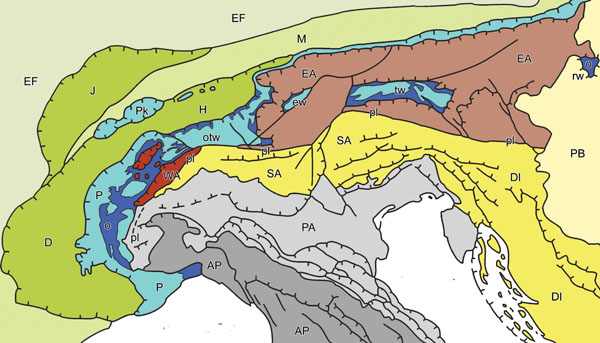
Tectonic map of the Alps. (1) Europe-vergent collisional belt: i) Western (WA) and Eastern (EA) Austroalpine; ii) Penninic domain: continental and ophiolitic (o) nappes in western Alpine arc (P) and tectonic windows (otw: Ossola-Tessin, ew: Engadine, tw: Tauern, rw: Rechnitz); Prealpine klippen (Pk); iii) Helvetic-Dauphinois (H-D) domain and Jura belt (J); iv) Molasse foredeep (M). (2) Post-collisional antithetic (south-vergent) belt of Southern Alps (SA), bounded by the Periadriatic lineament (pl). Dinaric (DI) and Apenninic (AP) thrust-and-fold belts. Pannonian basin (PB), European (EF) and Padane-Adriatic (PA) forelands (Dal Piaz et al., 2003).
The Helvetic units are thrust over the Molasse foredeep, a northward-thinning sedimentary wedge which developed from the Oligocene to the Late Miocene, with multiple successions of shallow marine and freshwater deposits. Its imbricated inner zone (Subalpine Molasse) was extensively dragged southwards below the frontal nappe stack. In the outer French-Swiss Alpine arc, the Molasse basin is bounded by the thin-skinned Jura fold-and-thrust belt of Late Miocene-Early Pliocene age.
The interior of the Alps has been revealed by the above-mentioned deep seismic experiments: two independent Moho surfaces have been recognized (Fig. 13, 21), i.e. the Adriatic and the underlying European Moho, the latter gently bending from the undeformed foreland to the base of the thick collisional wedge and further south (Figure 26). This means that the Alps display a lithospheric asymmetry, the orogeny was dominated by Europe-vergent displacements, and the antithetic Southalpine belt is only a superficial and late feature of the Adriatic upper plate. If surface geology is integrated with interpretation of seismic images, the Europe-vergent orogen is a mantle-free crustal wedge which tapers to the north, floating over the current European lower plate, and indented, to the south, by the current Adriatic (Southalpine) lithosphere (Figure 26).
Figure 26. Crustal and litospheric section of North-Western Alps.
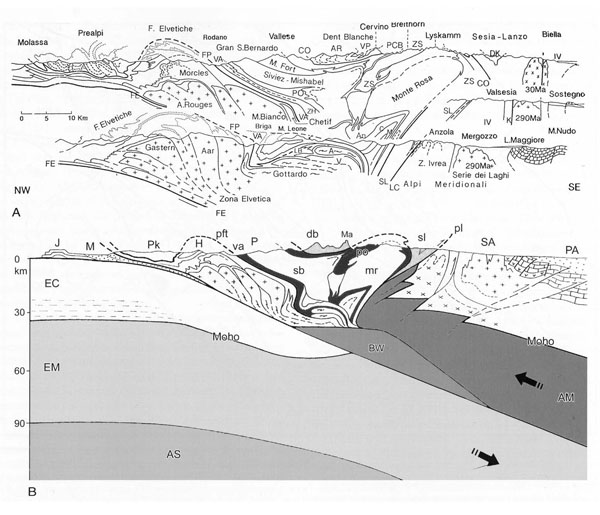
(A) Crustal section (modified from Dal Piaz, 1992) - Main structural features of the exposed collisional belt in the north-western Alps by superposition of the Aosta-Valais section (structural depression) over of the Ossola-Tessin dome, modified from Argand (1909), Escher et al. (1988) and Dal Piaz (1992). From top to bottom 1) Western Austroalpine: 2a Diorite-kinzigitic zone (DK), Valpelline series (VP), Arolla series (AR), Sesia-Lanzo zone (SL), Fobello-Rimella (FR) shear zone along the Canavese line (CL). 2) Ophiolitic Piedmont zone derived from the closure of the Ligurian-Piedmont ocean, including the composite Combin zone (CO), Zermatt-Saas unit (ZS) and Antrona unit (An). 3) Upper-Lower Penninic basement and cover nappes: Monte Rosa, Portiengrat (Pt), Camughera-Moncucco (CM), Pontis (PO), Zone houillère (ZH), Berisal (B), Monte Leone (ML), Lebendun (LB), Antigorio (A), Verampio (V); outer-lower Penninic Sion-Courmayeur and Valais units (VA). 4) Helvetic-Dauphinois domain: see cartoon for details. 5) Frontal-basal thrusts of the Penninic (FP) and Helvetic (FE) nappe stack. 6) Southern Alps: Laghi series (SLA), including Permian granites, and Ivrea-Verbano (IV) lower crust. Oligocene magmatism (32-30 Ma): Biella and Miagliano intrusives (30 Ma) are also shown, without labels.
(B) Lithospheric section (from Dal Piaz, 1997, modified) - 1) Western Austroalpine: Sesia-Lanzo inlier (sl) and Dent Blanche nappe s.l. (db), including the Matterhorn-Monte Cervino (Ma). 2) Penninic domain (P): Piedmont ophiolitic units (po), Monte Rosa (mr) and Grand St Bernard (sb) nappes, lower-outer Penninic Valais zone (va), Penninic klippen (Pk), Penninic frontal thrust (pft). 3) Helvetic basedment slices (crosses, dashed), adherent cover units and decollement cover nappes (H). 4) Oligocene-Neogene Molasse foredeep (M). 5) Jura belt (J). 6) Buried wedge (BW) of European mantle or high-P lower crustal units. 7) European lithosphere: continental crust (EC) and mantle (EM) of subducting lower plate; asthenosphere (AS). 8) Adriatic lithosphere (SA): antithetic (south-vergent) retro-wedge belt of the Southern Alps (SA) and mantle (AM); Canavese line, Periadriatic fault system (pl). 8) Padane-Adriatic foreland (PA).
The orogenic wedge groups the Austroalpine, Penninic and Helvetic superunits, and may be subdivided into three diachronous parts: 1) the older and upper part (Eastern Austroalpine) is a large nappe stack, including a subduction complex which developed before the Late Cretaceous deposition of Gosau beds; 2) the inner-axial part (Western Austroalpine-Penninic) is a pre- to syn-collisional subduction complex that was generated from the Late Cretaceous to the Eocene, includes the Adria/Europe collisional zone marked by one or more ophiolitic units (at different structural levels), and displays polyphase metamorphism evolving from diachronous blueschist or eclogite facies imprint (Late Cretaceous-Eocene subduction), locally coesite- and diamond-bearing, to high-T Barrovian overprint (mature collision, slab break-off) of Late Eocene-Early Oligocene age, cut in turn by the Periadriatic magmatism (32-30 Ma); 3) the outer and younger part (Helvetic-Dauphinois) consists of shallower basement slices and largely detached cover nappes which derived from the European continental margin, escaped the subduction low thermal regime and, from the Oligocene, were accreted in front of the exhumed Austroalpine-Penninic wedge; pre-Alpine features are well preserved in the basement units even if in places they display a Barrovian metamorphic overprint (Frey et al., 1999; Oberhänsli et al., 2004).
The essential features of the Europe-vergent Austroalpine, Penninic and Helvetic tectonic domains and the antithetic Southern Alps are briefly outlined in follows, starting from the Structural Model of Italy (updated legend) and based on the review of the Alps (Dal Piaz et al., 2003) elaborated for the International Geological Congress in Florence (2004), and on the recent, previously cited literature.
Austroalpine
The Austroalpine is subdivided into two sectors (eastern and western), based on contrasting distribution, structural position, and main deformation age, as pointed out in the first part (Bigi et al., 1990; Dal Piaz et al., 2003; Oberhänsli et al., 2004; Schmid et al., 2004).
Eastern Austroalpine
The Eastern Austroalpine tectonic system is a thick stack of cover and basement nappes located on top of the Europe-vergent belt, extending from the Swiss/Austrian border to the Vienna and Pannonian basins (Fig. 27). Its allocthony over the Penninic zone is marked by some ophiolitic units of debated provenance exposed in the Engadine, Tauern and Rechnitz windows. To the north, the Austroalpine system overrides the outer-lower Penninic Rheno-Danubian flysch belt of Cretaceous-Eocene age and, to the west, the ophiolitic Platta-Arosa nappe, as well as, south of the Engadine sinistral strike-slip fault, the Margna basement unit, generally correlated since Staub’s work (Fig. 7) to the Dent Blanche nappe. To the south it is juxtaposed to the continental crust of the Southern Alps along the Tonale-Pusteria-Gailtal-Karawanken (Periadriatic) fault system. The kinematic evolution of the Eastern Austroalpine is constrained by eclogitic to Barrovian fabrics dated as “mid” Cretaceous (eoalpine), and by Gosau beds (Coniacian-Eocene intramontane basins) that seal major thrusts, therefore the essential tectono-metamorphic history of the Eastern Austroalpine is older than that (Late Cretaceous-Eocene) recorded by the Western Austroalpine. The main tectonic transport inferred from kinematic indicators was first to the north or north-west and then to the north.
The Eastern Austroalpine is subdivided into two (Matura et al., 1980; Spicher, 1980: Tectonic Map of Switzerland; Structural Model of Italy, 1990; Schmid et al., 2004; Schuster et al., 2004) or three main groups of nappes (classic Austrian literature, e.g. Neubauer & Höch, 2000, Fig. 27), often with different assumptions and results. The Upper and Middle Austroalpine correspond in general to the Upper Austroalpine in the Structural Model of Italy. The subdivision used in follows is based on the present-day geometrical sequence of the nappe stack, from top to bottom, without paleogeographic inferences.
Upper Austroalpine – On the top of the nappe stack we can see the Northern Calcareous Alps and some Paleozoic basement nappes. The Northern Calcareous Alps are an imbricated pile of decollement cover nappes forming a 500 km-long belt extending from the Swiss eastern edge to the Vienna basin (Plöchinger, 1995; Mandl, 2000; Behrmann et al., 2006). These cover sheets are made up of Permian and/or Mesozoic clastic to carbonate deposits, including platform (Hauptdolomit) and basin (Hallstat) sequences, mainly detached from the Graywacke zone along evaporite-bearing shales. Their origin is controversial. The Paleozoic basement units are located west (Steinach klippe), south-east (Gurktal nappe, Graz Paleozoic) and north (Graywacke zone, Grauwackenzone) of the Tauern window. These units consist of Early Paleozoic to Middle Carboniferous metasediments and discordant Late Carboniferous to Tertiary covers, with a greenschist to very-low-grade Alpine imprint (e.g., Sassi & Menegazzo, 1971; Oberhänsli et al., 2004; Schuster et al., 2004).
The underlying group of nappes (Middle Austroalpine in Neubauer & Höch, 2000, and some other reconstructions) put together most of the huge crystalline basement and minor cover units of the Eastern Alps, i.e. the Silvretta, Oetztal and Ortler-Campo nappes, west of the Tauern window, followed to the south by the Ulten-Tonale nappe, a fragment of Variscan lower continental crust with eclogitic relics and slices of garnet-spinel peridotite (Godard et al., 1996; Martin et al., 2009; Scambelluri et al., this volume). The Silvretta nappe is a large crustal fragment located north-west of the Engadine window, which overrides the ophiolitic Platta-Arosa nappe and the Lower Austroalpine Bernina (to the south-west) and Landeck Phyllite units (to the north). It consists of a polymetamorphic basement which is dominated by Variscan and older mineral assemblages in various P-T conditions (sillimanite, kyanite and later andalusite), and is discontinuously overprinted by a very low-grade to greenschist facies Alpine retrogression (Magetti et al., 1987; Frey et al., 1999). Its Mesozoic cover is only preserved in the south-western edge of the nappe.
The Oetztal nappe is exposed between the Engadine and Tauern windows. It is a large and essentially coherent fragment of polymetamirphic basement which overrides the Penninic units of Engadine, the Landeck Phyllite and the Mesozoic metasediments of Scarl nappe, along the Schlinig thrust. Moreover, the Oetztal nappe plays as the hangingwall of the Brenner detachment and is juxtaposed to the Lower Austroalpine Insbruck Phyllite along the northern extension of the Brenner line. The basement is represented by paragneisses and micaschists with gneissic granitoids (Late Ordovician protoliths), minor mafic bodies, and some marbles. Scattered remnants of Permian-Eotriassic silico-clastic metasediments and Mesozoic marbles are preserved in the eastern side, tectonically capped by the Steinach nappe. The high-grade fabric is mainly Variscan, marked by kyanite, sillimanite or andalusite index-minerals in distinct sectors (Neubauer et al., 1999; Neubauer, 2002; Schuster et al., 2004). The eolpine overprint (Thöni, 1999) ranges from very low-grade (northern Oetztal) to greenschist (chloritoid), amphibolite (staurolite-kyanite, southern Oetztal), and local eclogite facies conditions on mafic rocks in a south-eastern lower subunit (Hoinkes et al., 1991, 1999; Miller. & Thöni, 1995; Schuster et al., 2004; Thöni, 2006).
The Ortler-Campo nappe nappe groups crustal slices of polimetamorphic basement and monometamorphic Permian-Mesozoic cover sequences. The former mainly consists of quartz-phyllites, micaschists and paragneisses with beds of marble and quartzite, and transposed bodies of gneissic granitoids from pre-Variscan protoliths. The latter consists of a Permian-Eotriassic clastic and evaporitic basal series, followed by dominant Triassic dolostones and marbles, extending from the Engadine to the Ortler massif. The nappe is characterized by a pre-Alpine greenschist to amphibolite facies imprint and an eoalpine low-grade retrogression mainly along shear zones, cut in turn by andesitic dykes and tonalite-gabbro intrusions of Oligocene age (Martin et al., 2009).
The central sector of the Easter Austroalpine, south of the Tauern window, is represented by the Mules-Merano-Anterselva complex and Schober-Kreuzeck groups, the narrowed eastern extension of the Oetztal basement. Differential uplift along the Deferegger-Anterselva-Valls fault (DAV) allowed the preservation of pre-Alpine amphibolite facies features and radiometric dating in the southern block, formed by micaschists, paragneisses, migmatites, amphibolites, marbles and gneissic granites from pre-Variscan protoliths, whereas in the northern block retrogressed rocks and phyllonites of eoalpine and later age are dominant (Borsi et al., 1973, 1978; Sassi et al., 1974), including relict eclogites of debated eoalpine age (Schober group).
From the Tauern window to the eastern end of the Alps, the Upper Austroalpine groups various basement and cover units, partly similar to those summarized above. The most important are, from top to bottom: the polyciclic Muralpen units, including the Micaschist-Marble complex (Niedere Tauern), the Speick complex (early Variscan ophiolite) and the Gleinalpe-Rennfeld core complex, followed by the Koralpe-Saualpe (Koriden) and Sieggraben basement units and the Pohorje massif which, as previously seen, are characterized by the occurrence of eoalpine eclogites from Permian mafic protoliths (Thöni, 1999, 2006; Sassi et al., 2004; Janak et al., 2006).
Lower Austroalpine - The Lower Austroalpine includes some basement and cover units located below the Upper (Middle) Austroalpine system and generally referred to as Lower Austroalpine: these units are exposed in the western (Err-Bernina), central (Innsbruck Quartz-Phyllite, Radstatt system) and eastern (Semmering-Wechsel window) ranges of the Eastern Austroalpine.
Figure 27. Overview map of the Eastern Alps.
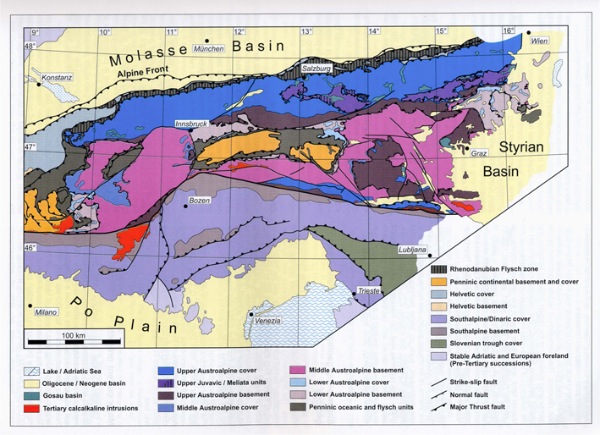
Overview map of the Eastern Alps (Neubauer & Höch, 2000). The crystalline basement is mainly related to the Middle Austroalpine, including the eclogitic and high-grade units.
The Err-Bernina is the lowermost group of basement and cover units sandwiched between the overlying Silvretta and Campo nappes and the underlying Margna and Platta-Arosa nappes. The basement consists of gneissic granitoids and minor paraschists, and is transected by numerous post-Variscan mafic dykes. The cover units consist of Permian-Cenomanian sequences, including the Saluver formation (Samedan zone, Graubünden) of Early-Middle Jurassic age, which is characterized by submarine breccias and sandstones deposited by mass-flow and turbidity currents. This formation is representative of an ocean-continent transition and a part of the distal margin of Adria (Froitzheim & Manatschal, 1996; Manatschal & Nievergelt, 1997; Manatschal & Bernoulli, 1999), like the Canavese zone (Ferrando et al., 2004).
The Innsbruck Phyllite is a large basement unit located between the Tauern window and the Inn valley, cosists of thick sequences of low-grade quartz-phyllites with interbeddings of quartzite, marble, metavolcanic rocks, and is capped by a few remnants of Permian-Triassic cover rocks. The Insbruck Phyllite is imbricated by the Hippold and Rechner nappes (Fig. 28): the former includes Eotriassic to Late Jurassic metasediments, the latter a typical ophiolitic suite which displays a blueschist facies imprint of Eocene age (Dingeldey et al., 1997), pointing for its Penninic affinity.
Figure 28. Rechner ophiolite.
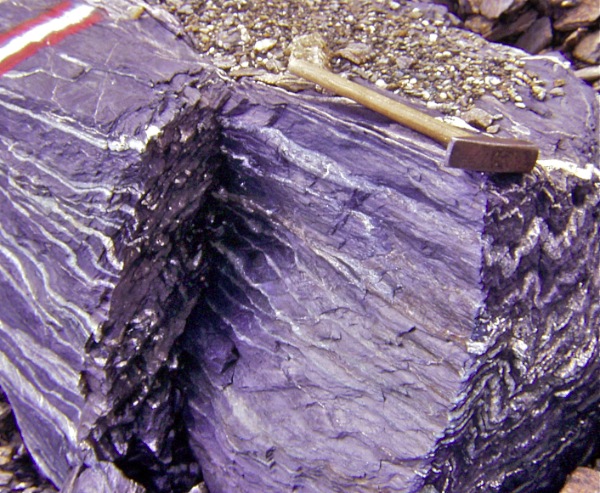
Jurassic metasedimentary cover: bluish slaty-metachert with abundand Mg-riebeckite and thin quartzitic bands.
The Radstadt units, which crop out around the north-eastern edge of the Tauern window (Radstätter Tauern), consist of Lower Paleozoic quartz-phyllites, mafic metavolcanics, marbles, paragneisses and metagranitoids, with greenschis facies eoalpine overprint, and of various cover sheets, including Permian-Triassic clastic and platform carbonate sequences, Liassic basinal limestones and breccias, Middle Jurassic-Lower Cretaceous radiolarian cherts, olistoliths and breccias.
The Semmering and Wechsel basement and cover units are exposed within a large window and to the east override the Bernstein-Rechnitz metaophiolite or disappear below the Neogene infill of the Vienna and Styria basins (Bigi et al., 1993; Oberhänsli et al., 2004). The Semmering system consists of a polymetamorphic basement, with migmatites, gneissic to mylonitic granites, and of Permian-Triassic clastic to carbonate and evaporitic sequences. It is underlain by a stack of basement units and related Permian-Triassic metasediments which constitute the Wechsel dome. The basement display an amphibolite facies regional metamorphism of Variscan age, marked by sillimanite or andalusite, whereas the phyllitic lower units show a greenschist facies imprint of debated age (Permian ?). The eoalpine oveprint increases southwards from greenschist to amphibolite facies (staurolite, kyanite).
The Matrai zone has been reported in the Structural Model as the lower Austroalpine units inside the Tauern window, even if it actually is a tectonic melange of basement sheets, Triassic marbles and ophiolite-bearing calcschists of debated Piedmont-Ligurian (Combin-type) affinity.
The Margna nappe is reported by the Structural Model as the lowermost composite unit of the Austroalpine system in the Central and Eastern Alps, highlighting its debated origin and close similarity with the Dent Blanche nappe, long ago supported by Staub (1957). The Margna nappe consists of Mesozoic metasediments, including radiolarian cherts and scarp breccias, and upper crust (paraschists and gneissic granitoids) and lower crust units (Fedoz high-grade metagabbro). The long-lasting debate on its paleostrucural position and my opinion on this issue have been reviewed and referenced in previous chapters.
Western Austroalpine
As shown in Fig. 29, the Western Austroalpine is a structurally composite nappe system, which is exposed in the Pennine and Graian Alps, from the southern Valais to the central and lower Aosta valley and north-western Piedmonte, and narrowing to the north-east along the internal side of the Ossola-Lugano steep belt (Argand’s root zone), south of the Ossola-Tessin dome. It consists of the Sesia-Lanzo zone (inlier) and numerous external slices (outliers), traditionally reported and grouped as Argand’s Dent Blanche nappe s.l. and its root zone. These continental units override or are tectonically interleaved with the structurally composite ophiolitic Piedmont zone, the major remnant of the Mesozoic ocean. The Structural Model (sheet 1) simply distinguishes three principal units with polyphase tectono-metamorphic features: i) Valpelline and 2nd Diorite-kinzigite upper units, thin rootless sheets of granulite-amphibolite facies lower continental crust of pre-Alpine age, similar to the Ivrea-Verbano zone, except for a weak and discontinuous blueschist or greenschist facies Alpine metamorphic reworking and shear zones (Ridley, 1989; Stünitz, 1989; Babist et al., 2006); in the Artogna valley the kinzigitic suite includes a large body of mantle peridotite (Dal Piaz et al., 1971; Beccaluva et al., 1979), not shown in the Structural Model; ii) Roisan zone and Mt Dolin cover metasediments of Mesozoic age, and basement units consisting of greenschist facies gneissic granitoids from Permian protoliths (Argand’s Arolla Series and Gneiss minuti complex, a term introduced by Gastaldi, 1871-74), layered Permian gabbros and Fobello-Rimella mylonitic shear zone along the contact with the Southalpine Ivrea lower crust, with strongly reduced (or missing) sequences of the Canavese zone between them (Artini & Melzi, 1900; Carraro et al., 1970; Schmid et al., 1987); iii) Eclogitic micascist complex (Stella, 1894) derived from Early Permian granitoids and older high-T paragneiss with kinzigitic affinity, including marbles, eclogitic gabbros (Corio and Monastero) and other mafic bodies. The Eclogitic micascist complex crops out in the internal part of the Sesia-Lanzo zone, up to the upper Sesia valley, and is tectonically juxtaposed to the underlying Gneiss Minuti complex.
Figure 29. Austroalpine-Penninic nappe stack in Aosta valley.
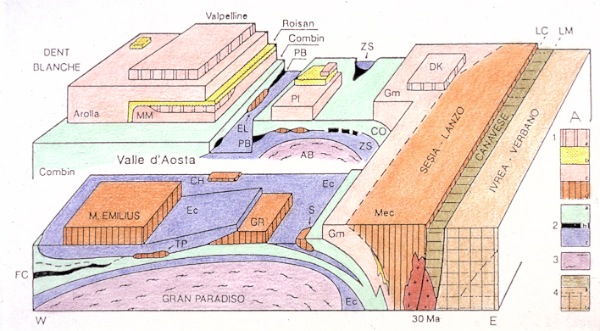
Simplified block diagram of the Austroalpine-Penninic wedge in Aosta valley. Upper Austroalpine outliers: Dent Blanche-Mt Mary (MM)-Pillonet (P) thrust system. Lower Austroalpine eclogitic outliers: Etirol-Levaz (EL), Chatillon (CH), Mt Emilius, Glacier-Rafray (GR), Tour Ponton (TP), Santanel (S). Sesia-Lanzo zone: Gneiss minuti complec (Gm), Eclogitic micaschist complex (Mec).
Legend: 1) Austroalpine: a) Valpelline and 2nd Diorite-kinzigitic unit, b) Roisan, Pillonet and Mt Dolin metasedimentary units, c) Arolla series and Gneiss minuti complex, d) Eclogitic micaschist complex. 2) Piedmont zone: a) Combin zone, b) Pancherot-Cime Bianche (PB) and Cogne (FC) units, c) Zermatt-Saas (ZS) and its extension south of Dora Baltea (Ec). 3) Arcesa-Brusson (AB) and Gran Paradiso nappe (GP). 4) Canavese zone (a), Southern Alps (b). Outer (LC) and inner (LM) Canavese lines.
The contact between the Valpelline and 2nd Diorite-kinzigitic units with the underlying gneissic granitoids (Arolla series, Gneiss Minuti complex) and eclogitic ortho- and paraschists (Eclogitic micaschists complex) is marked by polyphase shear zones, without trace of Mesozoic metasediments (Fig. 30). In the Structural Model this contact is marked by a second-rank thrust, suggesting ductile shear during differential exhumation, instead of a far origin from the Ivrea zone (Carraro et al., 1970). Rock-types similar to those of the Eclogitic micaschist complex also occur in the Mt Emilius, Rafray-Glacier, Tour Ponton, Santanel, Chatillon and Etirol-Levaz slices. The latter two are barely represented in the Structural Model that ignores the Acque Rosse slice, as it was discovered only later (Paganelli et al., 1995; Beltrando et al., 2009a). The different structural position of the non eclogitic upper Austroalpine Dent Blanche-Mt Mary-Pillonet thrust system in respect with the eclogitic lower Austroalpine outliers was cleverly recognized by Ballevre et al. (1986), but the younger age (Eocene) of the eclogitic imprint in the latter units (Dal Piaz et al., 2001) was stil unknown at that time, as well as the already mentioned Late Cretaceous isotope dating on retentive system from the Sesia-Lanzo Eclogitic Micascists complex. Focusing on the original version of the Structural Model, the eclogitic basement slice brought to light by road excavations near Grun (Rolfo et al., 2004) was erroneously linked to the Arcesa-Brusson dome. The Verres slice was attributed to the frontal part of the Sesia-Lanzo Gneiss Minuti complex, through a couple of antiformal and synformal folds with high-angle axial plane (Lardeaux & Spalla, 1991; Spalla et al., 1991). As previously discussed, the Verres unit is now included within the group of eclogitic outliers (strongly retrogressed), as a north-east extension, together with the new Tilly sliver, of the eclogitic Santanel unit (Battiston et al., 1987).
Figure 30. Mylonitic contact between Arolla and Valpelline units of Dent Blanche nappe.
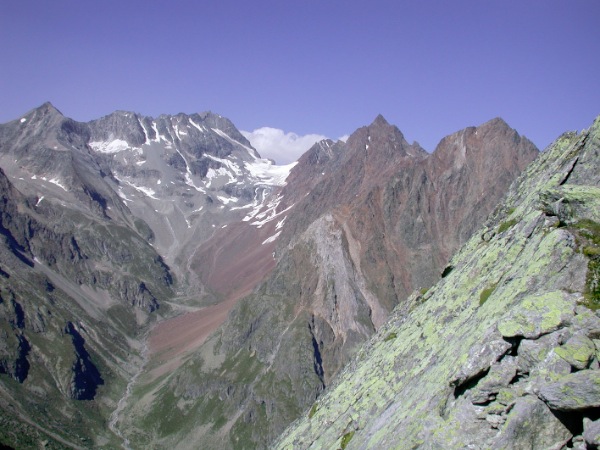
Tectonic contact between the Arolla gneissic granitoids (gray, left) and the overlying kinzigitic complex of Valpelline unit (reddisch, right), marked by a thick mylonitic shear zone, Comba de la Sassa, Valpelline (Dal Piaz, 1992; Pennacchioni & Guermani, 1993; Bucher et al., 2003, 2004).
Summing up, two groups of Austroalpine outliers can be identified in the Aosta valley, based on their structural position and contrasting P-T-time evolution history (Ballèvre et al. 1986; Ballèvre and Merle 1993; Dal Piaz 1999; Dal Piaz et al., 2001): i) the upper one is represented by the Dent Blanche-Mt Mary-Pillonet thrust system which, like the Sesia-Lanzo inlier, occurs on top of the collisional nappe stack, over the entire ophiolitic Piedmont zone; ii) the lower one is represented by the Mt Emilius, Glacier-Rafray, Etirol-Levaz and other minor basement slices, presently located along the tectonic contact between the upper (Combin) and lower (Zermatt-Saas) Piedmont ophiolitic nappes, or within the latter (Fig. 31).
Figure 31. Tectonic map of North-Western Alps.
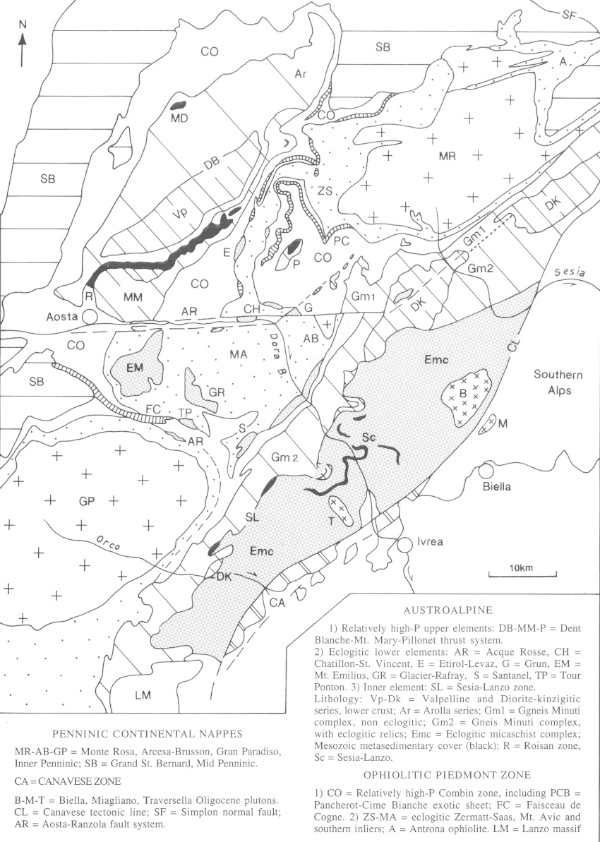
Tectonic map of the North-Western Alps (Dal Piaz, 1999). Western Austroalpine: 1) Upper outliers (non eclogitic): Dent Blanche (DB)-Mt Mary (MM)-Pillonet (P) thrust system; 2) Lower outliers (eclogitic): Acque Rosse (AR), Chatillon (CH), Etirol-Levaz (E), Grun (G), Mt Emilius (EM), Glacier-Rafray (GR), Santanel-Verres (S), Tour Ponton (T); 3) Sesia-Lanzo zone (SL). Lithology: Valpelline (Vp) and 2nd Diorite-kinzigitic series (Dk); Arolla series (Ar), Gneiss minuti complex (Gm1: non eclogitic, Gm2: eclogitic relics); Eclogitic micaschist complex (Emc); Mesozoic cover units: Roisan zone (R); Scalaro, Sesia-Lanzo (Sc). Piedmont zone: 1) Relatively high-P (scarce blueschist relics) Combin zone (CO), including Pancherot-Cime Bianche (PCB), Frilihorn and Cogne (FC) Permian-Mesozoic decollement cover units of debated continental affinity; 2) Eclogitic Zermatt-Saas zone (ZS) and its extension to the Mt Avic massif (MA), beyond the Aosta-Ranzola fault; Antrona ophiolite (A); Lanzo Massif (LM). Inner-upper Penninic Monte Rosa (MR), Arcesa-Brusson (AB), Gran Paradiso (GP) and mid-Penninic Grand St Bernard (SB) nappe systems. Canavese zone and Canavese tectonic line (CA), Aosta-Ranzola fault system (AR), Simplon normal fault (SF).
This subdivision is corroborated by a contrast of metamorphic and chronological constraints: i) the upper Austroalpine outliers display blueschist facies relics dated as Late Cretaceous in the Pillonet klippe (Cortiana et al., 1998), and similar to those of unknown age in the Combin footwall; ii) the lower Austroalpine outliers and Zermatt-Saas ophiolite display a similar eclogitic imprint of Early-Middle Eocene age (Dal Piaz et al., 2001; Beltrando et al., 2009a, 2010b), disregarding the ultrahigh-P conditions documented in the Lago di Cignana unit (Compagnoni & Rolfo, 2003; Forster et al., 2004).
The metamorphic (P ≥ 0.5 GPa) and temporal (25-30 Ma) gap between them does mean that these groups of Austroalpine-Piedmont nappes were diachronously subducted, followed independent paths and were finally coupled during the late exhumation stage, when both were overptinted by the Late Eocene-Early Oligocene greenschist facies regional metamorphism. During the Oligocene, the Sesia-Lanzo Eclogitic complex reached the surface and was coverd by volcaniclastic deposits (Scheuring et al., 1974). In the meantime, the collisional nappe pile was fragmented and noticeably displaced by the east-west trending Aosta-Ranzola normal fault system (Bistacchi et al., 2001), taking the upper Austroalpine outliers north of the Aosta valley about to the same altitude of the lower outliers in the southern side. As a matter of fact, the entire Austroalpine-Piedmont nappe stack is presently exposed in the lowered northern block, whereas only the lower Austroalpine outliers and Zermatt-Saas ophiolite are preserved in the footwall southern block, due to differential uplift and erosion (Fig. 31).
The principal prealpine protoliths of the Western Austroalpine system are represented by the following rocks (Diehl et al., 1952; Kienast & Nicot, 1971; Dal Piaz et al., 1972; Boriani et al., 1974; Compagnoni et al., 1977; Gosso et al., 1979; Vuichard, 1987, 1989; Stünitz, 1989; Dal Piaz, 1993; Gardien et al., 1994; Venturini, 1995): i) pre-granitic felsic granulites, amphibolite-facies metapelites, garnet-rich restites and migmatites with interbeddings of mafic granulite, amphibolite and marble, and concordant to discordant leucocratic dykes; ii) Lower Permian granite-quartzdiorites and gabbros in the Dent Blanche nappe (Diehl et al., 1952; Dal Piaz et al., 1977; Bussy et al., 1998; Monjoie et al., 2007) and Pillonet klippe (Dal Piaz, 1976); iii) detached remnants of a Triassic-Cretaceous sedimentary cover. These protolith are wholly to partly preserved in Alpine low-strain domains of the Valpelline series and 2nd Diorite-kinzigitic zone (Fig. 32), and can also be recognized in the underlying Austroalpine basement units, strongly reworked by the polyphase Alpine overprint, thanks to scattered megascopic, mesoscopic or mineral relics. Typical cases are the gneissic bodies with kinzigitic affinity partly preserved within the Eclogitic micaschist complex in some tributaries of the Gressoney valley. The pre-Permian, probably Variscan age of the pre-Alpine tectono-metamorphic history is firmly established only where some Permian granitoids sharply intrude the high-T schistosity and anatectic fabric, as it can be observed, for instance, in the Mt Morion massif which is poorly affected by Alpine transposition (Burri et al., 1998). This conclusion, however, can not be generalized as the contact between the metamorphic and igneous bodies is often a mylonitic shear zone, therefore we can not exclude a thermal reworking during the Permian lithosphere extension and emplacement of gabbro at the base of a thinned continental crust, as it is attested and dated in the Ivrea zone. Elsewhere, especially in the eclogitic lower outliers and Sesia-Lanzo inlier the high-grade prealpine basement is pervasively retrogressed or converted to Alpine eclogite facies micaschists and pegmatite-derived micaceous quartzites.
A few mafic bodies are also included in the eclogitic lower Austroalpine outliers, i.e. the granulitic gabbros of the Etirol-Levaz slice (Kienast, 1983; Ballèvre et al., 1986) and Mt Emilius klippe (Dal Piaz et al., 1983; Pennacchioni, 1996), Mt Emilius websterite, which is associated to a partly serpentinized mantle peridotite slice (Benciolini, 1989). Further metagabbros are reported from the eclogitic Sesia-Lanzo basement (Venturini, 1995; Rebay & Spalla, 2001).
To sum up, multiple intrusions of granitoids and gabbro emplaced in the Austroalpine domain between the end of the Variscan metamorphism and the Triassic, based on field evidence. Radiometric zircon dates on some granitoid and mafic bodies are bracketed between 293 and 288 Ma (Bussi et al., 1998), whereas biotite cooling ages at the Permian-Triassic boundary were obtained in the Matterhorn and Collon gabbros (Dal Piaz et al., 1977).
Figure 32. Southern face of Mt Nery.
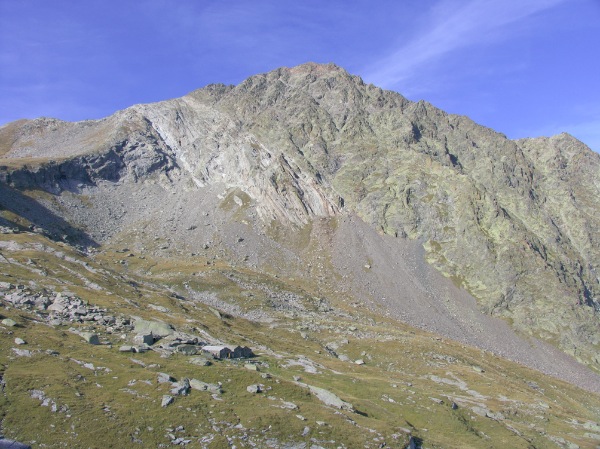
The granulite-amphibolite facies kinzigitic complex is perfectly preserved in the Mt Mary massif, between Ayas and Gressoney valleys. A large greenschist-facies schear zone (clear band)overprints previous blueschist-facies mylonites (late eoalpine ?) along the contact between the 2nd Diorite-kinzigitic unit and underlying metagranitoids and eclogitic micaschists, and in turn is transected by a thick andesitic dyke (Dal Piaz et al., 1971, 1972; Stünitz, 1989; Babist et al., 2006).
The scattered remnants of the metasedimentary cover are mainly represented by Triassic platform carbonates with Late Triassic microfossils (Ciarapica et al., 2010; Fig. 22), rift breccias (Jurassic?), basinal limestones (Late Jurassic?) and flysch-type calcschists (Cretaceous?). As previously discussed, the affiliation of the Fe-Mn quartzite over the Cignana lake (Ballevre & Kienast, 1987) is an open problem. These ophiolite-free sequences are associated to the upper outliers (Roisan zone) and, locally, to the Sesia-Lanzo inlier. The original sedimentary cover of the lower Austroalpine basement outliers, if really did exist, could be represented by the Permian-Mesozoic decollement unit (Pancherot-Cime Bianche) presently transposed at the base of the Combin zone or inside its lower part, between two ophiolitic Combin units (Dal Piaz, 1999; Bucher et al., 2003, 2004).
The Western Austroalpine was metamorphosed and ductilely deformed from the Late Cretaceous to the Early Oligocene under blueschist or eclogitic and then overall greenschist facies conditions (Dal Piaz et al., 1972; Compagnoni & Maffeo, 1973; Frey et al., 1974, 1999; Compagnoni, 1977; Compagnoni et al., 1977; Passchier et al., 1981; Lardeaux et al., 1982; Dal Piaz et al., 1983; Ballèvre et al., 1986; Polino et al., 1990; Lardeaux & Spalla, 1991; Scambelluri et al., 1998; Spalla et al., 1996; Konrad-Schmolke, 2006; Rebay & Messiga, 2007; Beltrando et al., 2010a).
The eclogitic metamorphism is extensively preserved in the internal Sesia-Lanzo zone, partly in the Mt Emilius klippe, and poorly in other lower Austroalpine outliers (Fig. 31). It is recorded by almandine-rich garnet, sodic pyroxenes, phengitic mica, rutile ± glaucophane, Mg-chloritoid, kyanite aggregates (after sillimanite) and zoisite, generating typical eclogitic micaschists from kinzigitic-type paragneisses and granitoids. Among them, it may be recalled the famous eclogitic granitoids of Mt Mucrone, near Biella (Compagnoni & Maffeo, 1973). Peak conditions are estimated around 550°C and 1.5-1.8 GPa. In contrast, the upper Austroalpine outliers only display a blueschist facies imprint, presently recorded by a few relics of aegirine, sodic amphiboles and white mica with high Si content (Ayrton et al., 1982; Cortiana et al., 1998). It can be recalled once again that the eclogite to blueschist facies metamorphism developed during the Late Crecaeous in the Sesia-Lanzo inlier and upper Austroalpine outliers, whereas the similar eclogitic imprint in the lower Austroalpine outliers is of Early-Middle Eocene age. These differences point to a north-westward prograding subduction and distant paleostructural sources of the concerned continental crust units. In the Late Eocene-Early Oligocene a greenschist facies overprint developed to different extent across the nappe stack. It is particularly pervasive in most of the Austroalpine outliers (except of the Valpelline top unit) and the external sector (Gneiss Minuti complex) of the Sesia-Lanzo zone, generally marked by the abundance of porphyroblastic albite.
Penninic Zone
Penninic is the classic name used to group the continental and oceanic nappes which originated from the European continental margin and the Mesozoic ocean. At the onset of plate convergence, both domains belonged to the subducting lower plate and in this sense the term Penninic is correct and is here maintained. However, since the original source of the ophiolitic units with respect to the spreading center is generally unknown, these units could be representative of the Mesozoic oceanic lithosphere potentially related either to the European or Adriatic or both diverging plates. Anyway, restoration of the collisional belt back to Alpine Tethys is complicated by simple-shear rifting, hyper-extended continental margins, mantle denudation and ribbon continents, whilst the frequent anomalies of the ophiolitic stratigraphy in respect with the normal oceanic crust point for a paleostructural setting characterized by active transform faults and fracture zones.
The Penninic zone is a thick pile of continental cover and/or basement nappes capped by and locally interlayered with large or minor ophiolitic units. As shown in the Structural Model, the upper part of the Penninic nappe stack is continuously exposed on both sides of the domal structure of the Lower Penninic nappes in the Ossola-Simplon-Tessin window, the major correlation gap along the longitudinal axis of the orogen. In the Western Alps, the top unit is the structurally composite ophiolitic Piedmont zone which extends from the Voltri massif, near Genoa, through the French-Italian Cottian and Graian Alps, to the structural depression of the Aosta valley and southern Valais, where it is capped by the Austroalpine system. In the Western Alps the Piedmont zone is underlain by the Upper-Inner Penninic Dora-Maira, Gran Paradiso and Monte Rosa nappes, often referred to as Internal Massifs (fixist name). These continental nappes are exposed as large domal structures and disappear below the overlying ophiolitic units in intervening structural depressions (Lanzo and middle Aosta valleys). The Dora-Maira and Monte Rosa nappes are partly underlain by the thinned and back-folded inner sector (Argand’s root zone) of the Grand St Bernard system, the bottom of Money unit (Gran Paradiso) is buried, whereas the Monte Rosa nappe appears to be underlain by the Antrona eclogitic ophiolite, a downward extension of the Zermatt-Saas nappe.
The Mid-Penninic Grand St Bernard system is a large belt of multiple basement and cover units which extends along the entire western arc, from the Ligurian to the Pennine Alps. It is frontally accreted by the Helvetic-Dauphinois basement and cover units along the Maritime and Cottian Alps, through a strip of Subbriançonnais (outer Briançonnais) embricated cover units. North of Moutiers, near the French-Italian boundary, the Subbriançonnais domain is replaced by the Outer Penninic Sion-Courmayeur or Valais zone, including the Versoyen-Roignais ophiolitic unit, externally delimited by the Penninic frontal thrust, the first-rank tectonic boundary between the Penninic and the Helvetic-Ultrahelvetic structural domains. The Lower and Inner Penninic nappe system groups the deepest nappes presently exposed in the core of the Ossola-Tessin window. They consist of basement units, mainly gneissic granitoids, minor sedimentary covers and scarce ophiolitic sequences with amphibolite facies Lepontine metamorphism of Late Eocene-Oligocene age.
Figure 33. The nappe stack in eastern Switzerland.
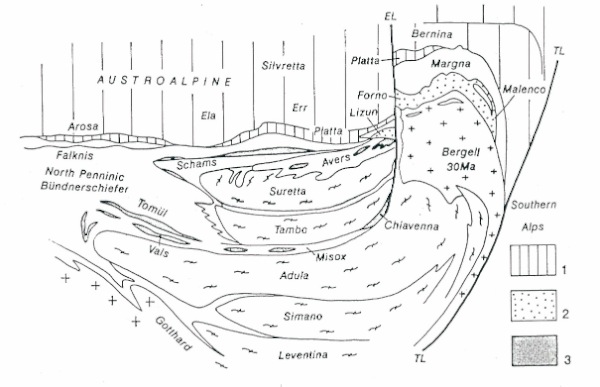
Collisional nappe stack in eastern Switzerland (simplified from Schmid et al., 1996, 1997): 1) Platta nappe; 2) Malenco-Forno-Lizun nappe, S-Penninic; 3) other North and S-Penninic ophiolites; Engadine line (EL); Tonale (Insubric) line (TL).
In the Central Alps, east of the Ossola-Tessin dome, we can see the overlying stack of oceanic and continental nappes, from top to bottom (Fig. 33): i) Platta-Arosa ophiolite; ii) Margna-Sella continental nappe (uppermost Penninic or lowermost Austroalpine); iii) Malenco-Avers calcschist-ophiolite unit; iv) Suretta and Tambo basement and cover nappes and allochtonous cover sheets with Briançonnais affinity (Schams, Falknis-Sulzfluh); v) Adula nappe, an eclogitic continental fragment of debated affiliation (top of the Lower Penninic: Bigi et al., 1990; Subpenninic: Schmid et al., 2004; Upper Penninic, by comparison with the Monte Rosa nappe). The Tambo nappe overrides the Chiavenna ophiolite slice (internal side) and the Misox zone (external side), connecting northwards to the Valais (N-Penninic) calcschists (Bündnerschiefer) and related flysch units (Trümpy, 1980; Bigi et al., 1990; Schmid et al., 1997, 2004; Liati et al., 2003, 2005). The southern part of the nappe stack is perturbed by the Oligocene Bergell intrusion and Engadine fault.
Passing to the Eastern Alps, the Penninic units are confined inside the Engadine, Tauern and Rechniz windows. These windows across the Austroalpine lid are described later and independently from the western units, in order to avoid univocal correlations among them.
Piedmont-Ligurian ophiolite system
The Piedmont zone is a structurally composite ophiolitic nappe system which in places includes exotic decollement cover units or small slivers of continental affinity.
The Alpine metamorphic ophiolites of the Piedmont zone and most of their eastern extension can be subdivided into non-eclogitic and eclogite facies units, both reworked by a greenschist to amphibolite facies overprint. Other differences concern the lithostratigraphic setting, varying between: i) carbonate to terrigenous flysch-type metasediments (calcschists s.l.), with multiple interleavings of tabular metabasalt and major ophiolitic bodies, including serpentinites mantled by ophicarbonate breccias or occasional Mn-rich metacherts; ii) large slices of normal to anomalous oceanic lithosphere, consisting of antigorite serpentinites (from mantle peridotite), often covered by ophicarbonate-ophicalcite breccias (Western Alps, Platta), followed by discontinuous metagabbro bodies, massive to pillow tholeiitic metabasalts, manganiferous metacherts (Middle-Late Jurassic), impure marbles, orogenic deposits, and subduction melanges (Cretaceous?).
In the Aosta valley and southern Valais the Piedmont zone is currently divided into two main ophiolite-bearing nappes: i) the overlying eclogite-free Combin (Tsaté) unit, which is characterized by scattered blueschist facies relics and a pervasive greenschist facies overprint, and ii) the underlying eclogitic Zermatt-Saas unit. North of the Aosta-Ranzola fault, the Piedmont zone includes the ophiolite-free Permian-Cretaceous decollement cover sheet which is located at the base of the ophiolitic Combin unit, or inside it, both forming the composite Combin zone. Disregarding the Alpine metamorphic imprint, the Combin unit roughly recalls the External Ligurides (Northern Apennines), which are characterized by melanges and olistolith-rich flysch sequences, whereas the Zermatt-Saas unit may be correlated with the fragments of oceanic lithosphere of the Internal Ligurides.
As previously outlined, the ophiolitic Combin unit is the tectonic sole of the Dent Blanche-Mt.Mary-Pillonet thrust system, altogether forming a tectonic multilayer which shares a similar blueschist (rare relics) to greenschist facies metamorphic evolution, starting with a Late Cretaceous subduction. The underlying Zermatt-Saas is overlain by or interleaved with the lower Austroalpine outliers, altogether forming a couple of nappes with Eocene eclogitic signature. The Zermatt-Saas ophiolite overrides the Monte Rosa and Gran Paradiso nappes and disappears beneath the Gran Nomenon and Mischabel back-folds (Dal Piaz, 1928; Elter, 1960; Escher et al., 1988, 1997), or back-thrusts (Freeman et al., 1997; Butler & Freeman, 1999; Markley et al., 1999), within the inner Grand St Bernard system. By contrast, in its external side the ophiolitic Combin unit is decoupled from the Zermatt-Saas unit and largely extends over the Grand St Bernard system (Fig. 31). The Combin unit consists of carbonate to terrigenous flysch-type metasediments (calcschists s.l.), often including tabular interleavings of greenschist facies metabasalts (prasinites) and lenticular slices of metagabbro and serpentinite, in places with adherent remnants of oceanic metasediments and Mn-rich deposits. Larger ophiolitic bodies are locally dominant especially in the upper part of the nappe. The Zermatt-Saas unit displays the best preserved remnants of subducted oceanic lithosphere. The ophiolitic suite consists of: i) thick basal titanclinohumite-rich antigorite serpentinites (from mantle peridotites), including pods and dykes of rodingitic gabbro, and locally mantled by ophicarbonate breccias (ophicalcites); ii) discontinuous bodies of Mg-rich and minor Fe-Ti-rich metagabbro and related cumulus ultramafics; iii) massive and pillowed metabasalts, first discovered by Bearth (1959), near Zermatt. The overlying sedimentary cover consists of impure quartzites, locally manganiferous, followed by marbles and minor calcschists, a sequence which roughly recalls the supraophiolitic oceanic cover (Callovian-Oxfordian radiolarian chert and Calpionella limestone) in the Northern Apennine. The cover ends with, or is merely represented by orogenic metasediments (ankerite-bearing garnet micaschists, often rich in large Mg-chloritoid ± glaucophane ± rare sodic pyroxene) and the Rifelberg-Garten unit (Bearth, 1953, 1967; Dal Piaz, 1965, 1974; Bucher et al., 2004). The latter is a peculiar metasedimentary formation of garnet calcschists and micaschists with dominant matrix supporting roundish to lenticular pebbles, generally 1-15 cm in size, of fresch to retrogressed eclogite from tholeiitic metabasalts. In addition, similar and contiguous horizons contain metric or larger boudins of glaucophane-rich eclogites and garnet amphibolites. The Rifelberg-Garten has been interpreted as a subduction melange. The Zermatt-Saas ophiolite displays an eclogitic imprint of Early-Middle Eocene age (49-43 Ma). The maximum peak conditions are recorded by coesite- and diamond-bearing eclogites found in Valtournanche, near the dam of the Cignana lake (Reinecke., 1991; Groppo et al., 2009; Selverstone et al., 2010). The post-eclogitic evolution is characterized by a continuum of re-equilibrations at decreasing pressure, first in relatively high-T blueschist facies conditions and later in Ab-amphibolite or greenschist facies conditions.
This tectonic and metamorphic subdivision of the Piedmont zone has been extended southwards to the Voltri Group, through the entire French-Italian Alps. Blueschist facies units, similar to the Combin sequences, extensively occur from the upper Lanzo and Susa valleys to the Queyras and Voltaggio-Montenotte zone, whereas eclogitic ophiolites similar to those of the Zermatt-Saas unit occur in the inner Lanzo and Susa (Rocciavrè) valleys, Monviso massif and Voltri Group (Bigi et al. 1990; Desmons et al., 1999). The best ophiolite suite is preserved in the Chenaillet area, between Susa and Briançon, consisting of Jurassic radiolarian chert, thick pillow lava, gabbro with high-T oceanic alteration, serpentinitic breccia with a sedimentary matrix and serpentinite, all poorly reworked by the Alpine overprint (Mevel et al., 1978; Lagabrielle, 1987). It can be outlined that these gabbros were emplaced before the onset of oceanic spreading, as attested by Triassic isotope ages, pervasive foliation and metamorphic re-equilibration that are absent in the overlying spilitic basalts (Costa & Caby, 2001).
Antrona unit - The Antrona unit is sandwiched between the Monte Rosa nappe and the underlying “root zone” (Camughera-Moncucco) of the Grand St Bernard nappe according to Argand's reconstruction (Fig. 26, 31). As previously discussed, this ophiolite is probably a slice of the Zermatt-Saas unit, tectonically infolded at the base of the Monte Rosa recumbent fold, rather than a North-Penninic derivative (Schmid et al., 2004; Pleuger et al., 2005; Herwartz et al., 2008): this is supported by lithological, geochemical and metamorphic features closely similar to those recorded in the Zermatt-Saas nappe.
Among the ophiolitic units exposed east of the Ossola-Tessin window, the Malenco-Avers nappe (Fig. 33) is located at the same structural level as the Piedmont zone (Bernoulli et al., 2003). The best ophiolitic exposures are provided by the overlying Platta-Arosa nappe.
Platta-Arosa nappe - In 1905, Gustav Steinmann recognized in this region the close association of serpentinites, diabase and radiolarite and considered this greenstone as characteristic for the axial part of the geosyncline and the deep ocean floor (Bernoulli et al., 2003; Bernoulli & Jenkyns, 2009). The Platta-Arosa nappe is closely similar to the Zermatt-Saas unit from a lithological point of view, but displays a less severe metamorphic imprint. It occurs below the entire western border of the Eastern Austroalpine nappe stack and reappears as uppermost Penninic unit inside the Engadine window (Dietrich, 1969, 1970, 1980; Bernoulli & Weissert, 1985; Bigi et al., 1990; Frisch et al., 1994; Bernoulli et al., 2003). The nappe can be subdivided into some sub-units: the Platta s.s., north of the amphibolite facies Malenco complex (Trommsdorff & Evans, 1977; Trommsdorff et al., 1993), beyond the Engadine line, followed northwards by the Arosa zone and then by Totalp zone, near Davos. The Platta nappe (s.l.) consists of supraophiolitic cover rocks with a close Liguride affinity, pillow lavas, gabbros transected by undeformed basaltic dykes, and ophicarbonate breccias which support the exposure of partly serpentinized mantle peridotite on the ocean floor at the end of rifting (Fig. 34). As previously recalled, this is the best example in the Alps of ocean-continent transition (Bernoulli & Weissert, 1985; Froitzheim et al., 1994; Froitzheim & Manatschal, 1996; Manatschal & Nievergelt, 1997; Desmurs et al., 2001, 2002; Schaltegger et al., 2002; Manatschall et al., 2003; Ferrando et al., 2004; Bernoulli & Jenkyns, 2009). The paleostructural setting has been clearly synthetized and figured by Bernoulli et al. (2003), showing that in continent-facing (proximal) sector of the ocean-continent transition, reconstructed from the upper serpentinite unit, the exhumed mantle is locally overlain by extensional allochthons, continental basement slices, pre-rift sediments and syn-rift marine breccias, that emplaced along extensional detachment faults. Otherwise, in the ocean-facing (distal) side of the ocean-continent transition, inferred from the lower serpentinite unit, the mantle is intruded by pods of gabbro and covered by pillow lavas and breccias that, as a whole, are sealed by a classic suite of deep-water sediments (Radiolarite Fm., Calpionella Limestone and Argille a Palombini). Summing up, this reconstruction is similar to the Early Cretaceous ocean-continent transition along the west Iberian passive margin where the subcontinental mantle was exhumed to the sea floor before the onset of ocean spreading (Bernoulli et al., 2003).
Figure 34. Platta ophiolitic nappe.
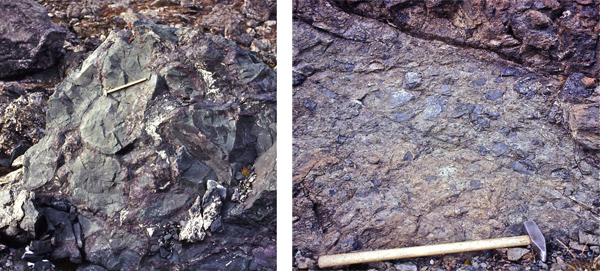
Spilitic pillow-lava (left) and serpentinite breccia (right), Falotta, Oberhalbstein.
Focusing on the igneous activity, both mafic rock types are characterized by εNd values typical for an asthenospheric mid-ocean ridge source, suggesting the existence of concurrent extensional tectonics with magma emplacement, at the onset of sea floor spreading across an exhumed subcontinental mantle. The igneous activity developed at the Callovian-Oxfordian boundary, documented by concordant U-Pb ages of 161 ± 1 Ma on gabbro zircons (Schaltegger et al., 2002). Submarine lava flows, dykes and gabbros show geochemical features typical of tholeiitic magmas (Frisch et al., 1994), evolving from T-MORB to N-MORB composition as they crystallized away from the continental margin (Desmurs et al., 2002). Note that simila results were obtained on selected samples of Piedmont ophiolites in the Aosta valley (Beccaluva et al., 2004).
The Platta-Arosa units can be restored near the Apulian continental margin (Schmid et al., 2004) and were accreted to the Eastern Austroalpine since the Cretaceous (Froitzheim et al., 1994). The Alpine metamorphism is recorded by blueschist facies minerals in mafic ophiolites (lawsonite, blue amphiboles) and metasediments (carpholite, phengite), and/or a low-grade overprint.
Penninic continental nappes
Upper-Inner Penninic units - The Dora-Maira, Gran Paradiso and Monte Rosa nappes and the Arcesa-Brusson window (Ayas valley) occur at the same structural level within the inner part of the collisional wedge, below the Piedmont zone, as lithologically similar but independent crustal fragments. They are made up of a polymetamorphic basement (Variscan plus Alpine), gneissic granitoid and augengneiss from Late Paleozoic intrusives, and a few remnants of Permian and/or Mesozoic cover metasediments (metaconglomerate, quartzite, dolostone, marble, calcschists). The pre-granitic basement consists of high-grade metapelites with abundant sillimanite-cordierite-bearing migmatites (Fig. 35), amphibolite facies mafic bodies from continental flood basalts and scarce marbles. The dominant Alpine derivatives are represented by various kinds of garnet-chloritoid ± kyanite micaschists, albite- or oligoclase-epidote-two micas schists, eclogite to albite-amphibolitic boudins and massive metagranites to schistose augengneisses, that record an eclogitic imprint of Eocene age and a greenschist to amphibolite facies evolution of Late Eocene-Early Oligocene age (Bearth, 1952; Michard, 1967; Dal Piaz, 1971; Compagnoni et al., 1974; Compagnoni & Lombardo, 1974; Dal Piaz & Lombardo, 1986; Ballèvre, 1988; Meffan-Main et al., 2004; Ring et al., 2005; Bertrand et al., 2005; Le Bayon & Ballèvre, 2006; Gabudianu et al., 2009; Beltrando et al. 2010a). In addition, the Brossasco-Isasca felsic unit of the Dora-Maira nappe displays the first occurrence of coesite found in the Alps (Chopin, 1984).
Figure 35. High-grade pre-granitic complex of Monte Rosa nappe.
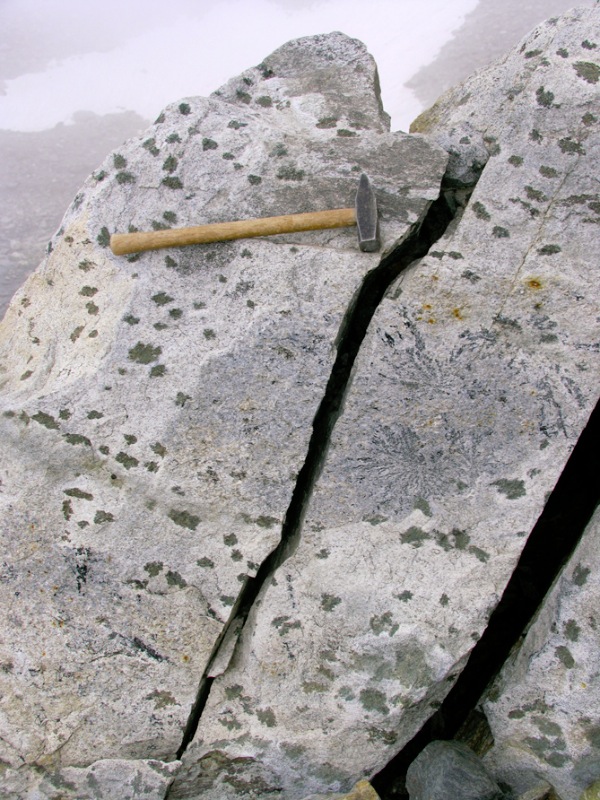
The Variscan pre-granitic complex (mainly migmatites) is relatively well preserved between the Indren and Garstelet glaciers, head of Gressoney valley, south of Rifugio Mantova. This figure shows a pegmatitic dyke with large crystals of cordierite (altered to pinite).
In the eastern Switzerland, the Suretta nappe is mainly built up of a polymetamorphic basement with intercalations of high-P mafic rocks, and of metagranitoids and augengneiss extensively developed in its norther side. Detached Mesozoic metasediments are concentrated along the upper flank of the nappe, and in tight synforms inside the northern basement. Its classic correlation with the Monte Rosa nappe is also adopted by the Structural Model.
Middle Penninic units - The Grand St Bernard (Briançonnais) tectonic system is a group of basement and cover nappes which extends from the Ligurian Alps to the Valais and Ossola valley, where it is the hangingwall of the Simplon normal fault (Mancktelow, 1992, 1995; Seward & Mancktelow, 1994). It also includes the Ambin massif, initially correlated by Argand to the overlying Monte Rosa fold-nappe. The structural position, facies evolution and metamorphic features allow the recognition of internal and intermediate-external basement and cover units which display a polyphase Alpine metamorphism marked by blueschist and/or greenschist facies fabrics of post-Luthetian age. The subduction-related blueschist facies imprint is particularly well recorded in the Acceglio, Ambin, Vanoise, Ruitor and some Valais areas. Four groups of nappes can be generally distinguished (Ellenberger, 1958; Lorenzoni, 1965; Michard, 1967; Caby, 1968; Frey et al. 1974, 1979; Lefèvre & Michard, 1976; Caby et al., 1978; Vanossi, 1992; Cortesogno, 1984; Escher, 1988; Desmons & Mercier, 1993; Gouffon, 1993; Cortesogno et al., 1997; Escher et al., 1997; Steck et al., 1997, 2001; Malusà et al., 2005; Sartori et al., 2006): i) polymetamorphic basement units which predate the Variscan unconformity, clearly documented by relict eclogite and amphibolite facies mineral assemblages which are preserved despite the Alpine overprint (e.g. Ambin, Ruitor, Siviez-Mischabel); ii) basement units of debated age, which are free from significant pre-Alpine relics and can be interpreted either as a Late Paleozoic monocyclic silicoclastic cover (tegument) of the older basement or as an older basement slice in which the penetrative Alpine overprint erased all previous fabrics; iii) Zone houillère, a large external unit consisting of thick sequences metaclastic deposits of Late Carboniferous-Permian age, with conglomerate and coal interbeddings, which marks the Variscan unconformity (Gb. Dal Piaz, 1939); iv) Briançonnais sedimentary cover (Ellenberger, 1953, 1958; Sturani, 1975; Sartori, 1990).
The polymetamorphic basement includes pre-Namurian paraschists and igneous bodies generated by a bimodal magmatism, and is characterized by an amphibolite facies regional metamorphism (Bearth, 1963; Giorgis et al., 1999), and traces of an older eclogitic event (Thelin et al., 1990; Cortesogno et al., 1997). Isotope dating of igneous protoliths from various basement units has noticeably aged the classic sequence of geological events (Bertrand et al., 2000a-b; Guillot et al., 2002; Gaggero et al., 2004; Sartori et al., 2006), and poses serious problems to the existence of the Variscan orogeny itself within the Briançonnais microcontinent. U-Pb zircon dating on metamorphic igneous bodies from Ambin, Vanoise and Ruitor massifs (Guillot et al., 1991, 2004; Bertrand et al., 2000a-b), previously regarded as Permian (eg. Mon Fort and Mt Pourri), yielded Cambrian and Ordovician ages, ranging from 512 ± 7 to 479 ± 5 Ma (Vanoise) and from 471 ± 2 to 460 ± 7 Ma (Ruitor), without clear evidence of polymetamorphic fabrics. Similar results have been obtained in the Briançonnais basement of the Ligurian Alps (Gaggero et al., 2004) for the bimodal (Cambrian-Ordovician) and acidic (Ordovician) igneous suites, as well as in the Swiss sector of the Grand St Bernard system (Bussy et al., 1996; Sartori et al., 2006). Cambrian zircon ages were obtained in the Siviez-Mischabel nappe from the Thyon granophyre (ca 500 Ma, Bussy et al., 1999) and a gabbro body (504 ± 2 Ma) emplaced into the Ergischorn ensemble (Bussy in Sartori et al., 2006). The long debated French-Swiss correlations across the Aosta valley have been reconsidered by Guillot et al. (2004), suggesting the existence of two paired igneous provinces, represented by the Ruitor-Sapey-Pontis external belt, characterized by 480-450 Ma-old aluminous intrusives and Variscan high-grade metamorphism (ca 330 Ma, Giorgis et al. 1999), and by the Vanoise-Mont Fallère-Siviez-Mischabel internal belt, characterized by 520-480 Ma-old alkaline magmatism and few traces of Variscan metamorphism. The massive to gneissic tonalite of the Grand Nomenon (Valsavarenche) back-thrust with a greenschist facies monometamorphic imprint (Amstutz, 1962; Elter, 1987; Freeman et al., 1997), traditionally regarded as a Permian intrusion (e.g., Ellenberger, 1958; Desmons & Mercier, 1993), yielded similar U-Pb conventional and SHRIMP zircon ages of 357 ± 24 and 356 ± 3 Ma (Bertrand et al., 2000b). In spite of the perfect concordance of U-Pb dating, the absence of pre-Alpine metamorphic relics makes doubtful the geological meaning of the isotope data and their inference on the absent or poorly represented Variscan orogeny (discussion in Malusà et al., 2005).
The older basement is intruded by Late Paleozoic granites and subvolcanic acidic bodies, and unconformably covered by Late Paleozoic-Early Triassic clastic deposits and volcanic-subvolcanic bodies, followed by the Briançonnais cover suite, consisting of Triassic shelf carbonates and evaporites, Middle Jurassic-Late Cretaceous pelagic to neritic deposits, and Lutethian-Priabonian turbidites, carefully described in Ellenberger’s (1958) memoir on the Vanoise, showing that the Briançonnais domain played as a structural high during the early stages of Mesozoic rifting (Sturani, 1975; Trümpy, 1980). Detailed descriptions of the Cottian and Ligurian Alps are provided by Guillaume (1969), Sturani (1975), Vanossi et al. (1984), Vanossi (1992), Desmons et al. (1999), Carminati & Gosso (2000). Three major post-Variscan volcanic units with calc-alkaline to alkaline features are described and dated in the Ligurian Alps by Dallagiovanna et al. (2009), yielding 285.6 ± 2.6, 272.7 ± 2.2 and 258.5 ± 2.8 Ma U-Pb zircon ages.
The Subbriançonnais is the outer domain of the Grand St Bernard nappe system in the French-Italian Alps. It is a group of Meso-Cenozoic decollement cover sheets detached from an unknow basement along an evaporitic horizon and displaced westwards over the Dauphinois domain in the Autapie-Embrunais region.
In the eastern Switzerland, the potential counterpart of the Grand St Bernard system is represented by the already mentioned Tambo nappe and related decollement cover units which display a close Briançonnais affinity.
Lepontine basement units - The Lower Penninic (Lepontine) system encompasses the deepest nappes presently exposed in the Alpine belt. From top to bottom, it mainly consists of the Monte Leone, Lebendun, Antigorio and Verampio nappes, in the Italian side, and the Bellinzona-Dascio, Adula, Maggia and Simano nappes in the Swiss side. These units display the features of a high-grade domal complex, due to dominant pre-Triassic basement nappes with an amphibolite facies regional (Lepontine) metamorphism and kilometric post-nappe recumbent folds (Monte Leone, Antigorio; Milnes, 1978; Milnes et al., 1981). Most nappes mainly consist of Alpine gneisses derived from Late Paleozoic granitoids, even if polymetamorphic paraschists, migmatites and ultramafic slices are locally widespread. The basement nappes are directly superposed along shear zones or are separated by thin sheets of cover metasediments, including Permian-Eotriassic metaconglomerate and quartzitic schists, Triassic marble, Jurassic breccia and dominant flysch-type calcschists.
Outer Penninic Valais domain
Focusing on the existence of a second oceanic basin (North-Penninic) within Alpine Tethys (Trümpy, 1980), we deal with the Valais zone in the external part of the Penninic domain, and with its ophiolitic units that have long been known as an oceanic suite. This outer-lower Penninic domain is an arcuate thin-skinned belt which extends from Moutiers to the upper Rhone valley, between the Briançonnais and Penninic frontal thrusts (Bigi et al., 1990). The Valais zone groups a stack of decollement flysch units of Cretaceous age (Brèches de Tarentaise, Sion-Courmayeur zone; Trümpy, 1954, 1980; Elter & Elter, 1965; Antoine, 1971; Fugenschuh et al., 1999), accreted in front of the Grand St Bernard nappe, together with a basal tectonic complex of Late Paleozoic-Jurassic metasediments. The Valais zone also includes some ophiolitic units: the most important and debated is the Versoyen (Roignais) unit, which tectonically overlies the Tarentaise breccia near the French-Italian boundary (Martin et al., 1994; Cannic et al., 1996; Bousquet et al., 2002; Beltrando et al., 2007; Masson et al., 2008), along a thrust contact (Bigi et al., 1990). The contact with the overlying Triassic-Liassic sequences of the Piccolo San Bernard zone (Elter & Elter, 1965) is of controversial interpretation, although a stratigraphic origin has recently been proposed (Masson et al., 2008). The Versoyen unit consists of serpentinite, massive to pillowed metabasalts and minor metagabbro, closely associated, together with some exotic bodies (Paleozoic micaschists, granite and impure quartzite), as tectonic slices or olistoliths, within a dominant sequence of Mesozoic flysch-type calcschists, phyllites and marbles of supposed Mesozoic age (Elter & Elter, 1965; Antoine, 1971; Antoine et al., 1973). The mafic rocks display N-T-MORB-type and island arc tholeiitic affinity (Mugnier et al., 2008), hydrothermal alteration and polyphase Alpine overprint recorded by blueschist and eclogite facies relics and greenschist facies reequilibration (Cannic et al., 1986; Bousquet et al., 2002; Beltrando et al., 2007; Masson et al., 2008).
As previously seen, the opening of the Valais (N-Penninic) basin had originally been considered as a coeval aulacogen of the Jurassic Piedmont ocean, prior to being referred to the Cretaceous, and connected to the generation of the Briançonnais terrane (Stampfli, 1993; Stampfli et al., 2002; Rosenbaum & Lister, 2005). The mafic rocks of the Versoyen unit, long envisaged as derived from the Mesozoic oceanic floor of the Valais basin have recently provided Permian (Beltrando et al., 2007: 272 ± 2, 267 ± 1) or Visean (Masson et al., 2008: 337.0 ± 4.1) U-Pb zircon ages, resulting a Variscan ophiolitic suture (Schärer et al., 2000; Masson et al., 2008) or a post-Variscan igneous suite emplaced into the continental crust during a process of lithospheric stretching before the break-up of Pangea (Beltrando et al., 2007, 2010a-b). In front of these conflicting ages and geological meaning, I prefer the interpretation given by Beltrando et al. (2007): Early Carboniferous dating poses problems, like that obtained from the Gran Nomenon metatonalite (Bertrand et al., 2000), on the Variscan orogeny and its regional metamorphism which are well recorded in the Ruitor basement and in the Helvetic massifs. In any case, whatever the age would be, the existence of a Cretaceous ocean in the Western Alps becomes very improbable (Masson et al., 2008).
Ophiolite-bearing calcschists are exposed in the Ossola-Tessin window (Martin et al. 1994). This unit is interbedded between the Lebendun and Monte Leone nappes and is generally related to the outer-inner Penninic (Valais) domain. Similar sequences of Mezozoic calcschists (Bündnerschiefer) and oceanic ophiolites extensively occur in the Grison area (Fig. 33), in front of and below (Misox zone) the Tambo-Shams-Suretta nappes (Steinmann & Stille, 1999). These metasediments grade upwards to the Turonian-Lower Eocene Prättigau flysch unit. Traces of Cretaceous magmatism or mantle denudation in units supposedly issued from the North-Penninic basin are reported in the Central Alps, either in the Chiavenna ophiolite (Liati et al., 2002), or in the Tasna nappe (Florineth & Froitzheim, 1994).
Penninic windows in the Eastern Alps
The continental and ophiolitic units exposed in the Engadine, Tauern and Rechnitz windows are briefly reviewed here, independently from their discussed connections (buried and eroded) to the Penninic zone in the Western Alps. The Lepontine (Lower Penninic) nappe stack is buried and only the Rheno-Danubian flysch belt extends from the Central to the Eastern Alps.
Engadine window - The Engadine window cuts the Eastern Austroalpine system at the Swiss-Austrian boundary, and exposes a stack of ophiolitic nappes and other thin units, mainly derived from turbiditic sequences of Mesozoic-Paleogene age (Oberhauser, 1980; Trümpy, 1980; Waibel & Frisch, 1989. Hoinkes et al., 1999). Below the previously described Platta-Arosa nappe, the following units crop out from top to bottom: i) Tasna nappe, a metasedimentary sequence of Permian to Late Cretaceous age and Briançonnais affinity, including slices of granitic basement; ii) ophiolitic Ramosch slice; iii) Roz-Tschampatsch cover unit; iv) lower calcschist unit (Grava-Tomül) with some greenschist facies metabasalts.
The Platta-Arosa ophiolite is referred to as South-Penninic (Piedmont-Ligurian), the underlying units as N-Penninic (Valais). Transition from continental to oceanic basement has been recognized in the Tasna nappe and Ramosch zone by Florineth & Froitzheim (1994). The latter mainly consists of serpentinized mantle peridotite, with ophicarbonates, serpentinite breccias, and basaltic pillow lavas (Vuichard, 1984).
Tauern window - The Tauern window is surrounded and overrided the central Eastern Austroalpine, showing a domal stack of oceanic and continental nappes of debated provenance. As reviewed in the first part, these nappes are supposed to be derived from the N-Penninic (Valais) and/or S-Penninic (Piedmont-Ligurian) basin, and from the Briançonnais microcontinent, the European distal passive margin or the Subpenninic domain (e.g., Bigi et al., 1990; Schmid et al., 2004; Kurz, 2007; Mattern & Wang, 2008). Anyway, the oceanic fragments were accreted to the Cretaceous Austroalpine wedge from the onset of the lower plate subduction, followed by the continental cover and basement nappes from the ocean closure onwards. The geological features described in the following update the structural and lithological subdivisions of the Structural Model of Italy, and mainly deals with the Italian south-western edge (Sander, 1929; Bianchi, 1934; Dal Piaz, 1934; De Vecchi & Baggio, 1982; De Vecchi, 1989; Bistacchi et al., 2004, 2007). Classic and modern referencing is essentially done in the first part of this review.
The capping unit of the Tauern window is the oceanic and structurally composite Glockner nappe (Obere Schieferhülle in fixist view) (Fig. 36). The underlying continental system is exposed in the western, central and south-eastern sides of the Tauern window, and is represented by basement sheets and/or decollement cover nappes (Untere Schieferhülle) and prominent crystalline basement massifs. An eclogite-bearing nappe is interposed between them in the southern part of the central window (Miller, 1977; Ratschbacher et al., 2004). Mineral-textural relics of high-P metamorphism are also recorded in the Glochner nappe (blueschist) and Grossvenediger basement (eclogitic boudins; Bianchi, 1934; Cortiana et al., 2004). The crystalline basement, often associated to remants of its post-Variscan cover, consists of Alpine gneissic granitoids and minor mafic bodies (Central Gneiss) from one or more Late Paleozoic batholiths, and various kinds of older (pre-granitic) host rocks with polymetamorphic features, which locally display significant relics of Ordovician eclogites (Sm-Nd: 421 Ma, U-Pb: 418-415 Ma; von Quadt et al. 1997) and an Early Paleozoic to Neoproterozoic oceanic suture, back-arc and island-arc settings (Grundmann, 1988; Reitz & Höll, 1988; Vavra & Frisch, 1989; Vavra & Hansen, 1991; Höck, 1993; Neubauer et al., 1999; Thöni, 1999; Neubauer, 2002; Schuster et al., 2004). The older Penninic basement was pervasively reworked by Variscan high-T metamorphism and anatectic migmatites associated with granitic intrusions.
Figure 36. Grossglochner.
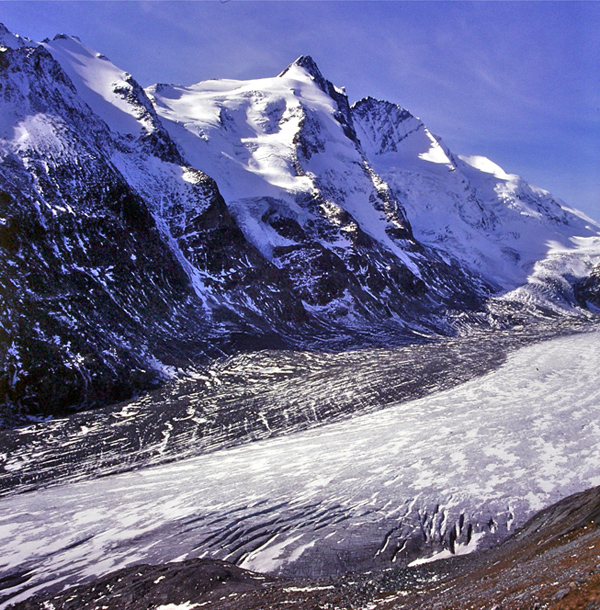
Grossglochner, the highest peak in Austria, gives its name to the major ophiolitic unit in the Eastern Alps.
The western Tauern window is dominated by the Venediger-Zillertal (internal) and Tux (external) nappes, forming the core of two gigantic east-west trending antiforms. In the central sector, the domal Granatspitz basement rises off the surrounding Mesozoic units, whereas in the south-eastern side the main basements are represented by the Sonnblick, Siglitz, Hochalm-Ankogel, Gössgraben, Mureck units (Oberhauser, 1980; Bigi et al., 1990, 1993; Flügel & Faupl, 1987; Kurz et al., 1996, 1998; Neubauer et al., 1999; Schmid et al., 2004). A peculiar feature of the Tauern nappe stack is the penetrative greenschist to amphibolite facies overprint and related ductile deformations of Tertiary age (collisional metamorphism), known from classic Sander’s work on Tauern crystallisation (Morteani & Raase, 1974; Hoinkes et al. 1999; Thöni, 1999, 2006; Oberhänsli et al., 2004; Schuster et al., 2004). Therefore, igneous and older metamorphic fabrics can be preserved to different extent in small to megascopic low-strain domains of the Alpine orogeny. As previously reviewed, the Tauern window is the result of concurrent, late-collisional tectonic processes, i.e. active north-south Adria-Europe plate contraction, tectonic unroofing by orogen-parallel displacement of the Austroalpine along antithetic low-angle extensional detachments on both sides of the window (Brenner and Katschberg normal faults), and upward-lateral extrusion of the Penninic nappe stack (Selverstone, 1988; Behrmann, 1988; Genser & Neubauer, 1989; Ratschbacher et al. 1991).
Focusing on the Italian part of the western Tauern window and nearby areas (Fig. 37), the Glockner top nappe consists of dominant calcschists (Bündnerschiefer) of supposed Late Jurassic-Early Cretaceous age, mainly derived from orogenic trench deposits; minor interbeddings of marble, metachert and quartzite are interpreted as thin oceanic cover remnants still attached to ophiolitic bodies, or scattered inside the calcschists. The ophiolitic suite is represented by the Alpine derivatives of fresch to spilitic (oceanic alteration) submarine tholeiitic basalts, minor serpentinite-ophycarbonate slices, often mylonitic, and a few coarse-grained flaser metagabbro, generally embedded within calcschists (De Vecchi & Piccirillo, 1968; De Vecchi & Baggio, 1982; De Vecchi, 1989; Bistacchi et al., 2003, 2007). The ophiolitic nappe is subdivided into subnappes thanks to the occurrence of scattered trails of exotic bodies, represented by boudinaged thin sheets of a continental cover suite made up of Permian-Eotriassic quartzitic schists and massive quartzites, as well as of Middle-Upper-Triassic marbles and dolostones (Dal Piaz, 1934). Similar rocks also occur, together with basement sliver, in the Matrai zone (Frisch et al. 1989), a relatively thick tectonic mixing shear-unit which discontinuously marks the contact with the overlying Austroalpine, and probably is representative of the original trench-slope transition.
Figure 37. Western Tauern window and surrounding units.
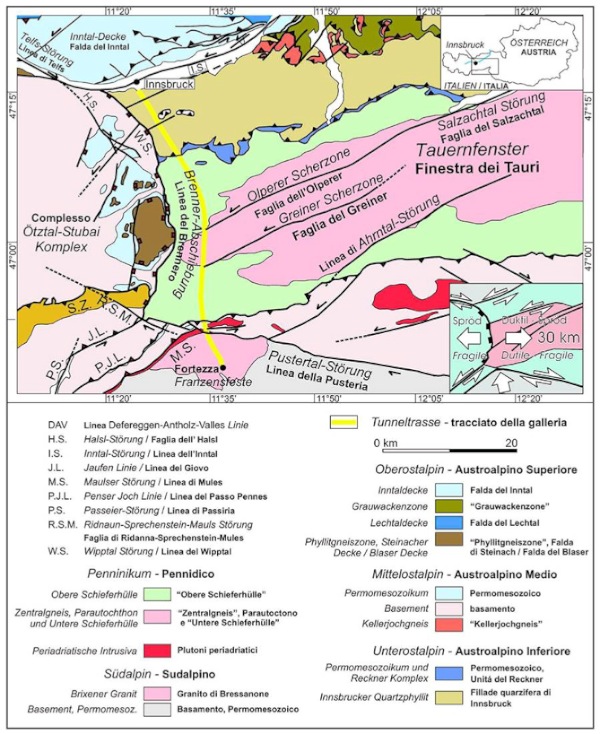
Tectonic map of the western Tauern window, surrounding Austroalpine nappes, Southalpine basement and Periadriatic intrusions. Inset: extensional mechanism for denudation of Penninic nappes. Geological study for Brenner Basis Tunnel, GBA-CFR-UIBK, 2006.
In the western Tauern window, the underlying stack of continental cover and basement nappes is regionally characterized by the east-west trending prominent antiforms of Gross Venediger-Zillertal and Tux, and a narrow subvertical synform (Greiner syncline) between them (De Vecchi & Baggio, 1982; Lammerer, 1986, 1988; Bistacchi et al., 2004, 2007). The Tux-Gross Venediger nappe system consists of a dominant granitic-granodioritic gneiss (Central Gneiss) from intrusives emplaced at the Carboniferous-Permian boundary (Cesare et al. 2001), minor pre-granitic paragneiss (Altes Dach), and autochtonous to detached cover sequences of Permian-Mesozoic age (Untere Shieferhülle). The post-Variscan metasedimentary cover essentially includes: i) Late Paleozoic-Eotriassic basal metaconglomerates (with basement pebbles), black schists, metamorphic arkose-sandstone successions and withish quartzites; ii) Middle-Late Triassic platform dolostones, calcitic-dolomitic marbles, evaporites (Fig. 38) and lagoonal deposits, with later terrigenous imputs; iii) Jurassic sedimentary scarp breccias (continental rifting), multiple alternances of pelitic, arenitic and carbonate metasediments with occasional metabasalts (Brennkogel facies), Late Jurassic finely banded radiolarian metacherts, and fossiliferous carbonates (Hocsteghen marble); iv) Cretaceous alternances of pelitic schists, impure quartzite and calcschists (Kaserer series).
Figure 38. Triassic evaporites.
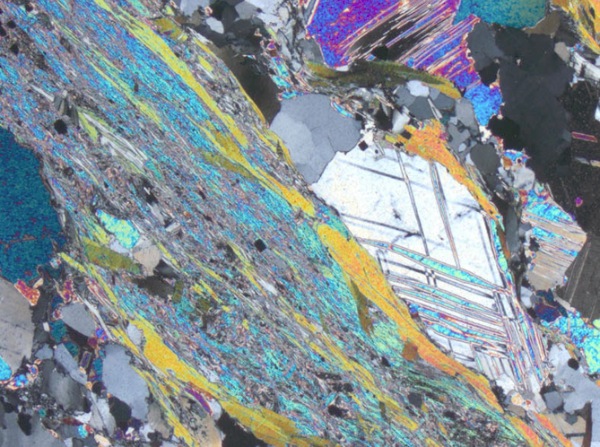
Thin section from German-facies anydrite-rich beds drilled in the buried Greiner synform between Tux and Gran Veneziano antiforms, Vizze valley, Tauern window.
The underlying Storz nappe occurs only in the central and eastern Tauern window, and is made up of a basal polymetamorphic basement with remnants of a pre-Variscan volcanic arc-marginal basin system, orthogneiss from Variscan intrusives, and post-Variscan clastic to phyllitic metasediments. In the central-southern Tauern window, the Storz nappe is overlain by the already mentioned Eclogite zone, that is characterized by abundand boudins of mafic eclogites inside high-P arkosic-pelitic metasediments, quartzites and marbles, roughly recalling the Furgg zone in the southern Monte Rosa nappe. The eclogitic bodies are derived from basalt and gabbro protoliths, tentatively referred to an initial rifting stage (Miller et al., 1980), and the high-P imprint yields the previously cited Eocene ages. The lowermost and largest nappes of the Tauern window mainly consist of gneissic intrusives from Late Paleozoic protoliths, and a pre-granitic polymetamorphic complex. The crystalline basement is unconformably covered by beds of Permian-Eotriassic basal metaconglomerate and/or quartzite, Triassic marbles with terrigenous interleavings and scarce metavolcanics, thick sequences of pelagic carbonates of Oxfordian-Early Titonian age (Hochstegen marble), and capping clastic metasediments of probably Cretaceous age, similar to Kaserer series in the Rote Wand-Modereck nappe.
The Penninic nappe stack is characterized by a pervasive Alpine greenschist to amphibolite facies overprint of Tertiary age (Tauernkrystallisation of Sander, 1911), but subduction blueschist facies metamorphism is locally documented by scattered mineral-textural relics (pseudomorphs after lawsonite) and thermodynamic modeling of mineral zoning (garnets) and fluid inclusions (Selverstone et al. 1984; Selverstone, 1985; Selverstone & Spear, 1985; Christensen et al., 1994).
The Oligocene post-orogenic magmatism is testified in the Italian side by the granodioritic-tonalitic Rensen and Riesenferner plutons and by the Mules tonalitic to gabbro-dioritic lamellae; all of them were emplaced in the surrounding Austroalpine or along the Periadriatic system. Oligocene leucocratic dykes are also found near the Austroalpine-Penninic contact at the southern margin of the Tauern window.
The Penninic units of the Tauern window escaped the Cretaceous orogeny, since at that time they had not yet entered the subduction zone. Indeed, these oceanic and continental nappes display only a polyphase Tertiary metamorphism and related fabrics which developed before the Oligocene, as firmly constrained by the emplacement of andesitic dykes of the Periadriatic magmatism (Mancktelow et al., 2001; Bistacchi et al., 2004). The first metamorphic event is the eclogitic imprint recorded in mafic and metasedimentary rocks of the narrow Eclogite zone (Miller, 1974; Dachs, 1986; Hoschek, 2001, 2007), and as scattered relics in the Grossvenediger basement and elsewhere. P-T estimates are 1.9-2.2 Gpa and 550-630°C and some 40Ar/39Ar dates point for their Eocene age, between 50 and 40 Ma (Zimmermann et al., 1994), as in the Western Alps. This may be confirmed by 40Ar/39Ar ages of high-Si phengites from the blueschist facies Mesozoic metasedimentary (Hippold) and ophiolitic (Reckner) units (Fig. 28) of the structurally composite Tarntal nappe, yielding 44-37 Ma (Dingeldey et al., 1997). This nappe, generally related to the Lower Austroalpine (Bigi et al., 1990; Dingeldey et al., 1997; Kurz et al., 1998) is probably a S-Penninic unit of the Tauern window embricated within the nearby Austroalpine system. Sheet 1 of the Structural Model schematically shows the Reckner serpentinite body inside the Lower Austroalpine sedimentary cover: it includes pods of pegmatoid gabbro and could be a further example of a denuded mantle slice covered by oceanic sediments.
The high-P event reached blueschist facies conditions in some Mesozoic units of the Tauern window, followed by the final and pervasive Barrovian oveprint which developed post-nappe greenschist to amphibolite facies mineral assemblages.
Dealing with the Penninic nappe stack in the western edge of the Tauern window (De Vecchi & Baggio, 1982; Hoschek, 1984; Selverstone, 1985; Selverstone et al., 1984; Selverstone & Spear, 1985; De Vecchi & Mezzacasa, 1986), high-P conditions (1.0-1.1 GPa) are recorded by Lower Shieferhülle units during collisional accretion to the Austroalpine system (Eocene), reaching the metamorphic T-peak during later exhumation (550°C, 0.7 GPa). Otherwise, petrological estimates on the Glockner nappe (Upper Shieferhülle) display a lower P-maximum of 0.7 GPa and a T-maximum of 475°C at 0.6-0.5 GPa. The burial difference between these units (ca 10 km) is explained by thinning of the ophiolitic nappe during Tauern window unroofing (Selverstone, 1985, 1988, 1993), even if an influence of the Tauern thermal perturbation on studied mineral assemblages can not be excluded. Radiometric ages (Christensen et al., 1994) show that garnet in the Lower Shieferhülle developed before garnet in the Upper Shieferhülle (55 Ma vs 35 Ma), both growing until the Oligocene (30 Ma). The shear sense indicators inferred from syn-kinematic garnet and s/c fabrics in Glockner metasediments and from post-kinematic garnet and honeblende cracking in Lower Shieferhülle show a generalized east-west extension with a top-down to the west kinematics (Selverstone, 1988, 1993; Lammerer & Weger, 1998). The greenschiest facies mylonites along the Brenner normal fault cut all previous foliations, but show the same kinematics detected in the Glockner nappe. Summing up, the tectonic denudation of the western Tauern window supposedly initiated since the Eocene-Oligocene boundary, the age of garnet growth in the Glockner nappe (Selverstone et al. 1993; Christensen et al., 1994; Axen et al., 1995; Bistacchi et al., 2004). For maps and details of the geological and structural features on the Italian side of the Brenner Basis Tunnel transect, see Bistacchi et al. (2004, 2007) and reports given to GEIE-BBT.
Rechnitz windows - Blueschist facies ophiolitic units reappear at the eastern corner of the Alps, near the Austrian-Hungarian border, inside the Rechnitz window and other minor occurrences (Möltener, Bernstein, Eisenberg) named, as a whole, Rechnitz Window Group (Höch & Koller, 1989; Koller & Höch, 1990). As shown in the Structural Model (sheet 2, Bigi et al., 1990), these units are discontinuously exposed below the Lower Austroalpine system, mainly surrounded or covered by Miocene-Pliocene deposits (Pahr, 1980). A thick Mesozoic cover sequence of calcareous to terrigenous metasediments is underlain by scattered bodies of metamorphic ophiolites, derived from tholeiitic basalt, gabbro, plagiogranite, ophicarbonate breccia and mantle ultramafics, showing diffuse traces of oceanic hydrothermal alteration. The Alpine evolution is characterized by a typical blueschist facies metamorphism (sodic pyroxene, glaucophane and crossite, lawsonite or epidote, high-Si phengites) of Eocene age (57 ± 3 Ma; Dunkl & Demeny, 1997), followed by a greenschist facies overprint (Koller, 1985; Schuster et al., 2004).
The exhumation of these hig-P oceanic remnants was accomplished at the beginning of the Neogene at the footwall of low-angle normal faults. This is inferred from K-Ar white mica cooling ages (22-19 Ma; Frank in Koller, 1985) and fission-track ages (Dunkl & Demeny, 1997) for zircon (21.9-13.4 Ma) and apatite (7.3-9.7 Ma).
Prealpine decollement nappe system
As shown in the Structural Model of Italy, various remants of formerly larger decollement cover nappes are preserved to different extent in the French-Swiss Prealps and outer ranges, between Annecy and the Glarus Alps (Trümpy, 1980; Caron et al., 1989). The nappe theory was born in this area, when Bertrand's insight and Shardt's regional work replaced Heim's double fold tenet by large thrusts (Trümpy, 1991). The Prealpine decollement nappes and klippen consist of Mesozoic-Paleogene sedimentary sequences which escaped subduction and Alpine metamorphism. Indeed, these units were decoupled from their oceanic and continental substratum since the beginning of the ocean closure and prograding collisional accretion of the European passive margin, and then they were displaced over the Helvetic-Ultrahelvetic foreland, in turn later rearranged as a thrust belt, and finally overrode the inner Molasse. The Chablis and Romandes Prealps are a stack of six major nappes preserved around the Lemano lake. The upper two nappes mainly consist of multiple Cretaceous (Helminotoid) or Cretaceous-Eocene flysch units, and the top one (Simme s.l.) also includes continental detritus of Southalpine affinity (Elter et al., 1966; Caron, 1972), and olistoliths of Ligurian-type ophiolites (Gets unit), i.e. obducted fragments of oceanic lithosphere free of orogenic metamorphism (Dal Piaz, 1999). The Breccia nappe is characterized by Jurassic rift breccias, whereas the underlying Medianes (or Klippen) nappes, mainly of Triassic-Eocene age, are dominated by competent Triassic-Jurassic carbonates (Rigid Medianes) or relatively soft limestone-shale sequences (Plastic Medianes); these units are generally correlated to the Briançonnais domain and their distal (pre-Piedmont) and proximal (Subbriançonnais) margins. The lowest nappes, maily Upper Cretaceous flysch (Niesen) and overlying melange, were probably issued from the Valais (North-Penninic) basin (Trümpy, 1980). Note that the Ligurian-type ophiolites (Gets unit) and their paleostructural restoration near the Canavese zone, suggested by Dal Piaz (1999) and corroborated by Bernoulli et al. (2003), go against the concept of a single Margna-Sesia fragment (Froitzheim et al., 1996).
Similar Helmintoid flysch nappes reappear in the southern Western Alps, either in the tectonic depression between the Pelvoux and Argentera basement highs (Parpaillon-Autapie), or south-east of the latter (Sanremo-Ventimiglia). Both are made up of arenaceous and/or calcareous turbiditic sequences of Upper Cretaceous to Paleocene and locally Eocene (?) age, probably coming from the Piedmont-Ligurian basin, and record the onset and outward propagation of orogenic contraction (Di Giulio, 1992). The Helmintoid flysch was displaced over and then beyond the Briançonnais zone, and lastly to the Dauphinois domain together with their sole of thin Subbriançonnais thrust sheets (Structural Model, sheets 1 and 3).
Rheno-Danubian flysch
The outer Penninic Rheno-Danubian thrust belt is a sedimentary wedge of Early Cretaceous to Late Eocene turbiditic sequences which extend along the entire northern margin of the Eastern Alps (Fig. 27), spanning the entire time of deposition of the Penninic and Helvetic flysch sequences (Oberhauser, 1995; Trautwein et al., 2001; Mattern & Wang, 2008). Even if locally, the Rheno-Danubian flysch is associated to some Jurassic ophiolite fragments, suggesting an oceanic environment for these turbiditic deposits. Their provenance is debated, and ranges, as for the Prättigau flysch, from the conventional N-Penninic (Valais) basin to the S-Penninic (Piedmont-Ligurian) domain (Frisch, 1979; Winkler et al., 1985; Oberhauser, 1995; Schmid et al., 2004). The flysch succession begins with carbonate-rich turbidites and evolves towards mainly silicoclastic deposits, with heavy mineral populations fed from the prograding Austroalpine frontal belt and other sources. Bentonite beds occur at the Paleocene/Eocene boundary. The outer Penninic flysch belt is underlain by discontinuous Jurassic-Eocene cover units, which are exposed in small windows and constitute the stripped eastern extension of the Helvetic-Ultrahelvetic decollement nappes, and both units are displaced over the Subalpine molasse thrust sheets.
Helvetic-Dauphinois zone
The Helvetic and Dauphinois zone (French side) extends along the entire external part of the Western and Central Alps, and narrows towards the outer Eastern Alps, where it is reduced to a few decollement sheets underneath the Penninic Rheno-Danubian flysch. The Helvetic-Dauphinois zone consists of prominent crystalline massifs, discordant or detached post-Variscan sedimentary covers and the classic stack of decollement nappes which predominate in the outer Swiss side (Sturani, 1975; Ramsay & Allison, 1979; Trümpy, 1980; Butler, 1985; Dietrich & Casey, 1989; Ramsay, 1989; Bigi et al., 1990; Sacchi, 1991; Compagnoni et al., this volume). Facies differences and structural position allow this external domain to be subdivided into the underlying-outer (Helvetic-Dauphinois) and overlying-inner (Ultrahelvetic-Ultradauphinois) basement and cover units.
External massifs
The prominent crystalline basement is widely exposed in the Argentera-Mercantour, Pelvoux (Haut-Dauphiné), Belledonne-Grandes Rousses, Aiguilles Rouges-Mont Blanc-Mt Chetif and Aar-Tavetsch-Gotthard external massifs (Fig. 39). The Mt Chetif slice, at the head of the Aosta valley, and the Gotthard massif are referred to as Ultrahelvetic basement units (Spicher, 1980; Bigi et al., 1990). Schmid et al. (2004), however, point for a Subpenninic pertinence of the Gottarhard massif, like the entire Lepontine nappe stack (Engi et al., 2004). Polymetamorphic (Variscan and older) and monometamorphic (only Variscan) basement units may be distinguished, evolving from an Early Paleozoic subduction cycle, through Variscan collision, Laurussia nappe stacking and regional metamorphism, to Carboniferous erosion, orogenic collapse, Late Paleozoic intrusions and wrench faulting (von Raumer, 1987, 1998; Bogdanoff et al., 1991; Bonin et al., 1993; von Raumer et al., 1999; von Raumer & Stampfli, 2008). Relics of Precambrian events are locally preserved. Scattered fossils in anchi-metamorphic or low-grade metasediments document the presence of Cambrian-Ordovician and Early Carboniferous deposits. Isotope dating provides additional information, concerning: i) Archean and Proterozoic cores of exotic detrital zircons; ii) Early Paleozoic (500-420 Ma) ophiolite-plagiogranite, eclogitic imprint, island arc gabbro, granitoids and migmatites; iii) Devonian (400-350 Ma) eclogites, mylonitic shear zones, rifting trondhjemites; iv) Late Variscan (340-300 Ma) greenschist to amphibolite facies metamorphism with widespread crustal anatexis and a Visean thermal peak; gabbro and granitoid intrusions; v) Early Permian granitoids and acidic volcanics (Bonin et al., 1993; von Raumer et al., 1999).
Figure 39. Mont Blanc external massif.
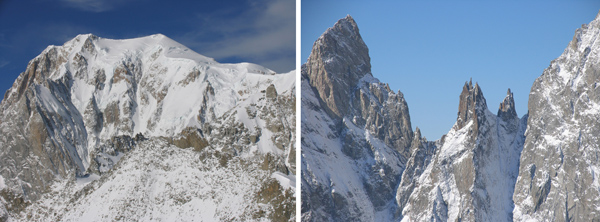
Left – Italian side of Mont Blanc massif, consisting of Late Paleozoic granite and minor capping paraschists.
Right – Arête de Peuterey: the Dames Anglaises, at the center, are modelled in a roof pendant of paraschists with a basal intrusive contact, and lowered into surrounding granitic rocks as a small graben bounded by high-angle faults.
Sedimentary cover
The Variscan basement is unconformably covered by thick sedimentary sequences of Late-Carboniferous to Oligocene age, characterized by asymmetric rifting, fault-bounded basins and passive-margin sequences (Malaroda, 1957; Sturani, 1962, 1963, 1975; Trümpy, 1980; Bigi et al., 1990). The discordant cover locally begins with continental clastic deposits of Late Westfalian and/or Stephanian age, in places coal-bearing. The Permian is represented by clastic sequences, locally including conglomerates (Verrucano facies) and acidic volcanics. Triassic formations consist of thin basal quartzites (Lower Scythian) and/or German-type successions, with shales and abundant evaporites (Keuper) that became the principal decollement horizons during Alpine contraction. In the Pelvoux massif there are spilitic lava flows, tuffs and dykes of Triassic age (Aumatre & Buffet, 1974; Bigi et al., 1990), roughly coheval with the calc-alkaline and shoshonitic extensional magmatism in the Southern Alps (Dal Piaz & Martin, 1998). Jurassic sediments are a thick sequence of limestone, marly limestone and shale, which record a period of rapid subsidence and include submarine sliding breccias and slumps. Cretaceous sediments display pelagic features, with thick limestone-marl alternances and black shales. However, the pelagic sequences are laterally replaced by neritic limestones or dolostones (Jurassic), and glauconitic-phosphatic deposits (Cretaceous), pointing to the existence of distinct paleostructural domains.
The Cretaceous-Tertiary transition is marked by a general emergence, that continued until the Lowermost, Middle or Upper Eocene when, in different sectors, the Helvetic domain was again flooded by the Tethyan sea, generally progressing from the outer to the inner side. This was roughly the time of the ocean closure, continental collision and rapid subduction of the European distal passive margin. The sedimentary evolution of the Helvetic realm is outlined by lagoonal sediments and/or transgressive nummulitic limestones, followed by pelagic marls, and then by thick flysch-type clastic sequences (Taveyanne sandstone-greywacke and similar units) of Priabonian-Lowermost Oligocene age, often including abundant volcanic detritus of diabase-andesite composition and Oligocene age (Ruffini et al., 1995, 1997). Afterwards, sedimentation was interrupted by submarine thrusting of the Helmintoid flysch nappes or ultimate emergence of the Helvetic domain, whereas in the external side the Helvetic sequence graded upwards to the molasse deposits of Oligocene-Miocene age (e.g., Val d'Illiez unit).
The Helvetic-Dauphinois cover units were strongly deformed during the Late Oligocene-Neogene (neoalpine) event, when the orogenic wave propagated from the Austroalpine-Penninic collisional wege to the evolving foreland. Basement and cover units were accreted in front of the exhumed collisional wedge, below the Penninic frontal thrust, and partly recrystallized in anchizone (sedimentary cover), greenschist and locally amphibolite facies conditions (southern Gotthard), with no traces of high-P subduction metamorphism (Frey et al., 1974, 1999).
Helvetic-Ultrahelvetic decollement cover nappes
The Helvetic and Ultrahelvetic cover nappes are decollement thrust sheets and minor recumbent folds which occur between the Penninic frontal thrust and the Molasse foredeep, from the Annecy and Lemano lakes to the Rhine valley (Trümpy, 1980; Ramsay, 1981, 1989; Ramsay et al., 1983; Bigi et al., 1990). The root zone steeply extends from the external basement massifs to the stripped footwall of the Penninic front. These nappes mainly consist of Mesozoic shelf to carbonates and flysch sequences of Upper Eocene-Lower Oligocene age, including the Taveyanne volcaniclastic beds. Permian conglomerates occur in the Glarus Alps. The cover nappes detached along incompetent horizons, such as Triassic evaporites and Middle Jurassic-Cretaceous shales. Oligocene thrusting of the Ultrahelvetic cover sheets developed before that of the underlying Helvetic units. Folds are persistent often for tens of kilometres and the overall structural pattern is representative of a relative Adria-Europe movement evolving from north-south to NW-SE and lastly east-west directions (Ramsay, 1989). The sequence of along-axis tectonic culminations and depressions of the nappe stack has been interpreted as a result of complex ramp-duplex geometry or post-nappe east-west shortening. From bottom to top, we may see the Morcles recumbent fold and the overlying trinity of Diablerets-Gellihorn and Wildhorn nappes, extensively preserved in the structural low between the prominent Mont Blanc and Aar massifs, and capped in turn by remnants of the Ultrahelvetic cover sheets. The pile of Helvetic nappes extends to the east, externally of the Aar massif: it is represented by the Säntis-Drusberg, Axen, Mürtschen and Kammlistock decollement nappes, consisting of Mesozoic (or Permian) to Eocene sedimentary sequences (Spicher, 1980; Trümpy, 1980; Bigi et al., 1990). Similar cover nappes occur in the Chaînes Subalpines (French Alps), west (Chartreuse) and south (Devoluy-Ventoux) of the Belledonne and Pelvoux massifs (Gratier et al., 1989). The Dauphinois sedimentary cover detached along its flat contact with the basement and was strongly deformed by folds, thrust-faults and strike-slip faults, mainly with a dextral kinematics. Slices of crystalline basement are locally displaced over the Subalpine Chaînes. Also in this area, the normal and transcurrent faults of the rifting stage were largely reactivated and inverted by Alpine tectonics, from the Oligocene to the Present.
Molasse Foredeep
The Molasse is a southward thickening sedimentary wedge a few tens kilometres wide, extending from Annecy and Geneva lake area to Vienna, partly buried below the frontal Alpine nappe stack. Its subsurface geometry is inferred from extensive geophysical and drilling exploration, and in the Structural Model of Italy (sheets 1-2) the contour lines (isobaths in metres) of the base of Oligocene are represented. The Molasse foredeep developed from the Oligocene to the Late Miocene, when the Helvetic-Ultrahelvetic basement and cover units were detached from the lithospheric lower plate, and progressively accreted in front of the Austroalpine-Penninic collisional wedge. Lithic clasts, heavy minerals and fission-track dating provide essential information on uplift, cooling and erosion of the Alpine nappe stack (eg., Frisch et al., 1999; Spiegel et al., 2000; Garzanti et al., 2007).
The Molasse infill of the basin is represented by clastic sediments fed by foreland sources and mainly by the erosion of the rising and outward prograding Alpine belt, and deposited during repeated alternances of shallow marine and freschwater conditions, both related to sea-level changes and regional tectonics (Fuchs, 1980; Trümpy, 1980). Older Molasse sediments are mainly shallow marine shales and sandstones of Lower Oligocene age, with ripple-marks to turbiditic features, and multiple intercalations of conglomerate bodies. The overlying Upper Oligocene-Lowermost Miocene freshwater deposits are represented by thick conglomerate sequences alternating with silty and marly shales, or by freschwater limestones and fining-upwards fluvial cycles including coal beds with a subtropical flora and mammalian fauna. Shallow marine conditions renewed in the late Lower Miocene. Deposition was dominated by sandstones and minor conglomerates along channeled tidal flats and fan delta. The sea ultimately retreated from the Molasse basin in the Middle to Upper Miocene. The drainage became dominated by a proto-Rhone river, flowing from the Austrian Alps and Bohemian massif to the west, a large fan delta from Alpine ranges, providing sands and gravels from recycled Alpine cover and basement units.
From a tectonic point of view, the Molasse sequences are subdivided into two main parts, i.e. an inner strip of embricated sheets (Southalpine Molasse), extensively dragged southwards below the low-angle basal thrust of Helvetic-Penninic and Eastern Austroalpine nappe stack (seismic evidence: Pfiffner et al., 1997; Bleibinhaus & Gebrande, 2005; Luschen et al., 2006), and the gently deformed to flat central-outer zone (Tabular-Folded or Plateau Molasse). The former consists of older marine and freeshwater deposits of Oligocene-Lower Miocene age, and constrains the onset of Molasse underthrusting. The latter grades and thickens from the external tabular and poorly deformed zone to the internal zone characterized by large open folds which involve the entire Molasse sequence up to the Late Miocene deposits.
Jura belt
The French-Swiss Jura is an arcuate thin-skinned belt of Mesozoic sediments which were detached from the European foreland basement along evaporite horizons of Middle-Late Triassic age (German facies), severely folded and faulted during the Late Miocene-Early Pliocene outward propagation of the neoalpine orogeny (Trümpy, 1980). This tectonic reconstruction was cleverly envisaged by Buxtorf, more than 100 years ago (Laubscher, 2008).
Deformation was facilitated by reactivation of normal faults generated during the Oligocene opening of the Rhine and Bresse graben, or before, and concentrated along soft beds (evaporite and minor shale) or bedding surfaces of Jurassic sequences.
The sedimentary succession is represented by a thinning nortward prism of limestone and shale mainly of Upper Jurassic age. Following classic Swiss reconstructions, two parallel belts are mapped in the Structural Model: the inner belt (Folded Jura) is dominated by thin-skin ramp-flat thrusts and related folds, mainly disharmonic, while the central-outer belt (Tabular Jura) is characterized by scattered and more gently folding. Both belts are obliquely fragmented by various fault systems, mainly with strike-slip kinematics.
Southern Alps
The Southern Alps are a typical example of deformed passive continental margin well exposed in a mountain range (e.g., Winterer & Bosellini, 1981; Bertotti et al., 1993; Carminati et al., 2010; Gaetani, this volume), including the magnificent natural monument of the Dolomites (Bosellini, 1996; Gianolla & Panizza, 2009), and many other wonders, as the Eocene fossils plants and fish at Bolca (Papazzoni & Trevisani, 2006), the dinosaur ichnosite at the Lavini di Marco (Avanzini et al., 2005), and the Paleozoic fossils recently discovered in the Venetian metamorphic basement (Dieni et al., 2005), together with the Adamello massif (Callegari & Brack, 2002) and related pseudotachylytes (Di Toro et al., 2004; Pennacchioni et al., 2006), as well as the Ivrea-Verbano zone, one of the most spectacular sections across the lower crust (Rutter et al., 2009). Until the Oligocene, this Adriatic domain was the gently deformed retro-wedge hinterland of the Alps, intensively reworked only at its eastern edge by the Paleogene SW-vergent Dinaric belt. From the Neogene, the Southalpine thrust-and-fold belt developed and progressively propagated towards the Padane-Adriatic foreland, reactivating rifting faults (Castellarin et al., 1992, 1993, 2006; Zattin et al., 2006; Doglioni & Carminati, 2008; Cuffaro et al., 2010). Its front is mainly buried beneath the alluvial deposits of the Po Plain and sealed by Late Pliocene or Quaternary deposits. To the north, the Southern Alps are bounded by the Periadriatic fault system. The Canavese zone (Elter et al., 1966; Sturani, 1975; Ferrando et al., 2004), with the related open problems and different interpretations, has been discussed in the previous part.
The Structural Model (sheets 1-2) represents the entire Southern Alps. This map was elaborated by the Southalpine working group, coordinated by Castellarin and Vai, and from surface geology it can be inferred a complete crustal cross-section of the Southern Alps. Thick cover successions are dominant in the eastern sector (Bosellini et al., 1996; Doglioni & Carminati, 2008; Gaetani, this volume), whereas the basement is nearly continuous from the central sector (upper-intermediate crust: Orobic Alps and Como and Maggiore-Verbano lakes area) to the western Southalpine edge where the lower continental crust is exposed in the Ivrea zone.
The crystalline basement encompasses various kinds of Variscan and older metamorphic granulite-amphibolite facies units derived from sedimentary, felsic and mafic igneous protoliths, later intruded by granitoids and gabbros of Permian age, and then deformed by Late Triassic-Jurassic rifting and passivce margin evolution (D’Amico & Mottana, 1976; Boriani et al., 1992; Bonin et al., 1993; Handy et al., 1999). Among them, it can be mentioned the Massiccio dei Laghi (Boriani et al., 1990), which outcrops in northern Piedmonte and southern Switzerland, grouping the famous Ivrea-Verbano zone and the nearby Serie dei Laghi (Maggiore, Varese and Como lakes): the complex features, pre-Alpine evolution and detailed references of this basement, carefully reviewed by Rutter et al. (2009), are summarized as follows.
Ivrea-Verbano zone
The Ivrea-Verbano zone consists of the Ivrea gabbro (a Permian batholith) and the roofing kinzigitic complex, both well exposed and extensively studied in the last decades, since modern pioneering works (e.g., Bertolani, 1969; Rivalenti et al., 1981, 1984; Hodges & Fountain, 1984; Pin, 1986; Brodie & Rutter, 1987), therefore becoming a classic model for igneous underplating below extending continental crust (e.g., Rutter et al., 1993, 1999, 2009; Schnetger, 1994; Quick et al., 1994; Henk et al., 1997; Sinigoi et al., this volume). The higher grade (originally deeper) kinzigitic paragneiss include beds of pure and impure marble and thin to thick (1-200m) bands of mafic granulite-amphibolite that have been interpreted as lava flows or intrusives within the primary (Paleozoic or older) sedimentary sequence of the accretionary complex represented by the kinzigitic unit (Sills & Tarney, 1984), showing different rare-earth element patterns with respect to the Permian mafic rocks. Towards its base, the kinzigitic unit includes an increasing number of mainly concordant, occasionally discordant sheets of Permian mafic intrusions, that can not be easily distinguished in the field from the older amphibolitic bands within the kinzigites. The southern edge of the Ivrea-Verbano zone is dominated by a huge layered mafic complex, some 10 km wide and extending along strike for some 40 km. This is the “mafic formation” (Rivalenti et al., 1981; Zingg, 1983), radiometrically dated as Permian (Pin, 1986: 295-280 Ma; Peressini et al., 2007: 288 ± 4). The western and originally deeper part of the batholith displays a vertical igneous layering and metamorphic granular fabrics, indicating that the layered rocks resided in the subsolidus regime sufficiently long for developing metamorphic granular fabrics; by contrast, the eastern and relatively shallower part of the mafic formation is characterized by igneous textures.
As shown in the Foglio Varallo of the Carta Geologica d’Italia at 1:100,000 scale, in the Structural Model and, with great detail, in the splendid modern map by Quik et al. (2003; also reproduced in Sinigoi et al., this volume), the Ivrea-Verbano zone includes some ultramafic bodies (peridotites, dunites and pyroxenites), mainly located towards its south-western edge and locally interacting with crustal magmas (Sinigoi et al., 1991). According to Quick et al. (1995), none of these ultramafic bodies would extend at depth, beyond the Ivrea Zone, even if some of them are clearly upper mantle slices, evidencing a petrological transition between the lower crust and the lithospheric mantle (e.g., Boudier et al., 1984). In this view, these slices may have been detached from upper mantle and accreted to the kinzigitic unit in Palaeozoic times (Quick et al., 1995). In any case, the existence in the discussed area of the positive gravity anomaly, long known as the geophysical Ivrea body (Berkhemer, 1968; Compagnoni et al., 1977; Roure et al., 1990; Carrozzo et al., 1991), suggests that the Adriatic lithospheric mantle lies beneath and not very far from the Ivrea-Verbano zone. The regional schistosity and associated folds developed in high-grade metasediments have been refolded, with associated axial-plane crenulation cleavages that overprint the partial melting leucosomes of kinzigitic migamitites. The Valle d‘Ossola transect displays a sequence of large antiformal structures (Southern fold, Candoglia, Massonne, Proman), without evidence of synforms beween them, and interpreted as large-scale Type-2 fold interference patterns (Ramsay, 1967; details and refs. in Rutter et al., 2009). These folding and metamorphic episodes predate the emplacement of the mafic formation (Boriani & Villa, 1997). Its intrusion generated contact migmatization and granulite facies conditions on the immediate roofing kinzigites (Schmid & Wood, 1976; Schmid, 1977; Henk et al., 1997). Partial melting processes in the kinzigitic unit, wholly attributed to the mafic intrusion (Schmid & Wood. 1976), were later restricted to the contact zone, where it overprinted a previous metamorphic event with migmatization and degranitization processes (Barboza et al., 1999, 2000; Peressini et al., 2007; Rutter et al. 2007).
Extensive partial melting of crustal sources was envisaged to be responsible for the generation of the Permian epigranites and the rhyolitic caldera in the lower Sesia valley, andesitic melt included (Quick et al., 2009).
The emplacement of the Permian intrusive complex was accompanied by stretching recorded by metamorphic and igneous rocks, with regional lineations plunging NE at about 30° (Rutter et al., 1993). Crustal stretching continued during post-intrusive cooling, mainly accommodated by discrete high-T shear zones with the same kinematics of earlier deformation history (Brodie & Rutter, 1987).
Serie dei Laghi
As shown in the Structural Model and summarized by Rutter et al. (2009), the Ivrea-Verbano zone is laterally juxtaposed along its south-eastern boundary to the Serie dei Laghi (Boriani et al., 1990a). The contact is marked by the Cossato-Mergozzo-Brissago line (Boriani et al., 1990a-b), with mylonites and mafic-intermediate intrusives of appinitic affinity and Permian age (Boriani & Giobbi, 2004). This tectonic discontinuity is cut, in turn, by the Pogallo fault, a younger mylonitic shear zone that is interpreted as a low-angle extensional detachment of Triassic or Jurassic age (Hodges & Fountain, 1984, Schmid et al., 1987; Zingg et al., 1990), even if it displays the same kinematic feature of previous high-T events.
Summing up, the Serie dei Laghi was probably juxtaposed to the underlying Ivrea-Verbano zone along the Cossato-Mergozzo-Brissago shear zone during the Late Paleozoic extensional event and related igneous underplating. Note that this tectonic line is not deformed by and therefore probably postdate the multiple generations of folds recognized in the Ivrea-Verbano zone and Serie dei Laghi. This is also the case of the Schlingen structure (vertical-axis foldding) that is related to the Variscan orogeny (Zurbriggen et al., 1997, 1998). The Serie dei Laghi consists of a suite of metasedimentary schists and gneisses (Scisti dei Laghi, Cenerigneiss, Gneiss Minuti), as well as of large bodies of orthogneiss derived from calc-alkaline Ordovician protoliths (Boriani et al., 1990b; Bigi et al., 1990; Zurbriggen et al., 1997, 1998). Relics of pre-Alpine ophiolites (amphibolites and ultramafic rocks ) have been found inside the Serie dei Laghi (Giobbi et al., 1997); this zone is cut by the Pogallo fault, showing a large left-lateral displacement. Lastly, the Serie dei Laghi includes large post-metamorphic granitic intrusions of Permian age (280-275 Ma; Boriani et al., 1992, 1995) and contemporaneous volcanic extrusives.
Central-Eastern side of the Southern Alps
Low-grade metamorphic basement - The Variscan regional metamorphism decreases from the high- and medium-grade (western and central Southern Alps) to greenschist facies (Venetian region, east of Adamello) and very low-grade (Carnian Alps) conditions (e.g., Boriani et al., 1974; Frey et al., 1974, 1999; Castellarin & Vai, 1982; Sassi et al., 1985; Sassi & Zirpoli, 1989; Sassi & Spiess, 1993; Brack et al., 2008; Cesare et al., 2010; Spiess et al., 2010). This imprint and related ductile deformations predate the discordant deposition of the Westphalian (Lombardy, Ticino) or Lower Permian clastic and volcanic sequences. The easternmost evidence of the Variscan amphibolite facies regional metamorphism (garnet-biotite-staurolite) is presently recorded in the country schists of the Adamello batholith (Brack et al., 2008), whereas the two basement occurrences in the eastern Southalpine (Alto Adige and Valsugana) show a greenschist facies imprint which predates the discordant deposition of Permian volcanic sequences and the intrusion of Permian plutons, mapped in the Structural Model (Bonin et al., 1993; Bellieni et al., 2010). The metamorphic basement (Sassi & Spiess, 1993; Spiess et al., 2010) is dominated by low-grade quartz-phyllitic metasediments with documented Cambrian-Silurian ages (Dieni et al., 2005, 2006; Sassi et al., 2008), including acidic metavolcanic bodies of Ordovician age and minor mafic rocks. Recent Rb-Sr and 40Ar-39Ar dating (Meli, 2004) confirms the Early Carboniferous age of the principal Variscan metamorphism also in the Southalpine domain, clustering at 350 Ma (Rb-Sr whole-rock and mica ages), whereas younger 40Ar-39Ar dates obtained from post-kinematic micas (330-325 Ma) may be interpreted as cooling ages of a second metamorephic pulse which would be closer to the principal Variscan event that it was previously envisaged (ca 330 Ma: Sassi et al., 1985; Thöni, 1999). Paleogeographic reconstructions suggest that, from the Silurian to the Devonian, the Southalpine and Austroalpine phyllitic units belonged to a southern passive continental margin, whereas paleoclimatic data show that, during the Carboniferous, the Southalpine was displaced to a more southern latitude than the Austroalpine (Spiess et al., 2010).
Permian igneous activity – The Permian magmatic activity extensively developed in the Adria-derived Austrolpine and Southalpine domains (Bigi et al., 1990; Bonin et al., 1993) as a post-Variscan extensional-transtensional lithospheric event (e.g., Dal Piaz & Martin, 1996; Rottura et al., 1998; Schaltegger & Brack, 2007; Bellieni et al., 2010), and is widely recorded also in the eastern Southern Alps (Bonin et al., 1993; Bellieni et al., 2010). From north to south, we can recognize: i) the Bressanone-Ivigna-Monte Croce plutons, intruded into the Bressanone quartz-phyllite and externally bounded by the Pusteria an North-Giudicarie lines; ii) minor bodies inside the phyllitic basement (Visonà et al., 1997); iii) the Monte Sabion pluton, intruded into the Rendena schists; iv) the large Cima d’Asta pluton and related satellites. The Permian intrusion age and geochemical features of this calc-alkaline suite is documented by classic and recent works (Del Moro & Visonà, 1982; Barth et al., 1993; Visonà et al., 2007; Marocchi et al., 2008; Avanzini et al., 2010), it consists of granodiorite, S-type granite, minor tonalite and, occasional gabbro, diorite and cordierite-orthopyroxene-bearing granite.
Also the Athesian volcanic group, wholly filling the structural depression open between the prominent metamorphic and intrusive basements of Bressanone and Valsugana-Cima d’Asta is Permian in age (Bosellini, 1996; Klötzli et al., 2003; Visonà et al., 2007; Marocchi et al., 2008; Avanzini et al., 2010). The volcanic sequence is a manly rhyolitic wedge which reaches its maximum thicknes of about 2 km along a border extensional fault of Permian age. The volcanic activity began in the Lower Permian, during the deposition of Ponte Gardena conglomerates (290.7 ± 3 Ma, U–Pb zircon age, Visonà et al. 2007b). In the central sector it started with andesitic lava flows (286.0 ± 3.0 Ma, U–Pb zircon age, Avanzini et al. 2010) and ended with rhyolitic ignimbrites and the development of large calderas (275.0 ± 4.9 Ma, U–Pb zircon age, Avanzini et al. 2010). In the western sector, the first volcanic pulse is rhyolitic (285.9 ± 1.6 Ma, U–Pb zircon age, Marocchi et al. 2008), and the subsequent activity was dominated by extrusion of rhyolitic ignimbrites (274.1 ± 1.6 Ma, U–Pb zircon age; Marocchi et al. 2008).
Triassic magmatism - A new sedimentary cycle developed in the Upper Permian, marked by continental deposits grading eastwards to shallow marine sediments (Gaetani, this volume). In the Triassic, the Southalpine domain was flooded and characterized by carbonate platform and basin systems, with regional evidence of continental rifting developed from the Norian to the Middle-Upper Jurassic boundary, when the Austroalpine and Southalpine domains became the subsiding passive continental margin of Adria. The magmatic activity developed during the Middle Triassic (mainly Ladinian) in the Predazzo-Monzoni-Fassa (Fig. 40) and Recoaro areas, both with shallow intrusions and volcanic activity, and rapidly exhausted (Castellarin & Rossi, 1981; Barbieri et al., 1982; Bosellini et al., 1982; Bonadiman et al., 1994; Visonà, 1997; Dal Piaz & Martin, 1998; Brack et al., 2005). It is also recorded in the Alpi Giulie (Gianolla, 1992). Following the review of Bellieni et al. (2010), the shallow intrusive and subvolcanic bodies consist of olivine–gabbro, monzonite to late granite and syenite, whereas the volcanic products are olivine-bearing basalts to rhyolites. Eruptions in the Predazzo-Monzoni area evolved from initial basaltic to lati-andesitic and latitic lava flows and ended with the a dyke swarm of K-basanites and lamprophyres with carbonatitic and occasionally spinel-peridotite xenolits (Carraro & Visona, 2003).
The calc-alkaline and shoshonitic affinity of the Triassic magmatism has been interpreted as evidence of extensional tectonics and partial melting of previously enriched mantle sources, instead of igneous products of active subduction. This interpretation, early envisaged by Dal Piaz et al. (1979) for Oligocene andesitic to high-K lamprophyric dykes in the Western Alps, was applied to the Triassic and Permian magmatism and further confirmed (e.g., Bonadiman et al., 1994; Dal Piaz & Martin, 1998; Rottura et al. 1998; Schaltegger & Brack 2007).
Venetian volcanic province – As reviewed in detail by Bellieni et al. (2010) and shown in the Structural Model, the Venetian province groups volcanic and sub-volcanic bodies occurring in the Southalpine thrust-and-fold belt and its Padane foreland, from the Adige valley and Lessini–Marostica area to the Berici-Euganei hills; its age is Paleocene to Middle Eocene (Visonà et al., 2007a) or Paleocene to Oligocene, west and east of the Castelvero tectonic line, respectively. The igneous suite consists of dominant basic-ultrabasic volcanic rocks which are representative of an alkaline series (mela-nephelinites, nephelinites, basanites, alkali basalts, hawaiites, trachybasalts) and of a moderately sub-alkaline series (transitional basalts, basaltic andesites), the latter occasionally including mantle xenoliths of spinel lherzolite and spinel harzburgite (Bellieni et al., 2010, and refs. therein). The geodynamic significance of the Venetian Volcanic Province is debated, as its deleopment is related to a mantle-plume inferred from tomographic images (Macera et al., 2003), or to a rifting and trans-tensional conditions supposedly associated with the Alpine event (Beccaluva et al., 2007; Bianchini et al., 2008).
Periadriatic Magmatism
A post-collisional magmatic cycle with calc-alcaline affinity mainly developed during the Oligocene (32–29 Ma) and was rapidly exhausted (Bigi et al., 1990; Blanckemburg et al., 1998). It was the object of the meeting “Il magmatismo tardo alpino nelle Alpi”, held Padova in the 1983, and related excursions to the Euganei hills and to the southern Adamello and Valmasino-Bregaglia massif (Proceedings in Mem. Soc. Geol. It., vol. 26, 1985). Traditionally called Periadriatic magmatism (Exner, 1976), it is represented by plutons, intrusive apophyses and a great number of dykes emplaced around the Periadriatic fault system, from the lower Aosta valley (Venturelli et al., 1984) to the eastern edge of the Alps (Faninger & Strucl, 1978), either across the inner part of the Austroalpine-Penninic wedge or in the nearby northern Southalpine (Fig. 20). Plutons and dykes (stars) are mapped in the Structural Model (sheets 1-2), together with the scarce remnants of volcanic products which escaped erosion in the Biellese and Bacher range. The Adamello batholith (Callegari et al., 1998; Callegari & Brack, 2002) is essentially older. Indeed, even if its northern pluton (Presanella) was generated concurrently with the Periadriatic magmatism, the emplacement of central and southern plutons gos back to the Eocene (42-37 Ma; Mayer et al., 2003).
The generation of the Adamello batholith and eventual other igneous bodies of Eocene age can be directly linked to active subduction at the onset of continental collision (Dietrich, 1976). This is not the case, however, of the Oligocene Periadriatic magmatism which developed about 20 Ma after the ocean closure. Major constraints that need to be evaluated are: i) regional extension in the country crust, facilitating the passive emplacement (rarely forced) of plutons and dykes; ii) variously enriched mantle sources; iii) thermal perturbation allowing partial melting of sources. The better solution is the chronological separation between subduction-related source enrichment and later melting of anomalyzed sources.
In this view, generation and ascent of Periadriatic melts to upper crustal reservoirs can be the igneous feedback of slab break-off and related thermal perturbation, coupled with extension and rapid uplift of the wedge and retro-wedge pair during active plate convergence (Dal Piaz & Gosso,1994; von Blankemburg & Davies, 1995; Blanckemburg et al., 1998).
Geological history
The Alpine-Meditarranean area is a mobile zone which, from the Precambrian, was reworked and rejuvenated by recurring divergent, transcurrent and convergent geodynamic processes. The pre-Alpine history may be reconstructed in the Southern Alps and, to various extents, also in the polycyclical basement units of the Austroalpine, Helvetic and Penninic domains, weakly overprinted by the Alpine orogeny.
Variscan and older evolution
The Paleozoic orogeny and Variscan collision gave rise to Pangea supercontinent by linkage of the Gondwana and Laurasia megacontinents and the consumption of intervening oceans. The future Alpine domains were located along the southern flank of this orogen. The classic Variscan term was coined to define the Carboniferous orogenic paroxysm in central Europe, but precursor events of Ordovician to Devonian age were later documented, suggesting the existence of an essentially continuous Paleozoic orogeny. Traces of older events are locally preserved, becoming more abundant in the last decade, a sort of renaissance of old ideas in vogue before the impact of isotope chronology. As a whole, the pre-Permian evolution of the Alps may be summarized as follows:
1) U-Pb data on zircon and Nd model ages suggest the existence of a Precambrian history. The oldest zircons found in various polymetamorphic basements refer to Precambrian clastic material eroded from extra-Alpine sources (Boriani et al., 1974). The occurrence of Proterozoic-Early Cambrian ocean spreading, island-arc activity, and bimodal volcanism is documented in the European and Adriatic basement, with debated traces of Precambrian amphibolite-eclogite facies metamorphism (Silvretta). Cambrian fossils are occasionally found.
2) Early Paleozoic northward subduction of the ocean flanking Gondwana to the north is recorded in the eastern Austroalpine and Helvetic basement, with recycled Precambrian rocks, mafic-ultramafic ophiolites and marginal basin remnants. Subduction is inferred from the accretion of a Paleozoic orogenic wedge, eclogitic mineral assemblages in mafic and felsic rocks, and calc-alkaline island-arc magmatism (460-430 Ma): these relics are mainly preserved in the Variscan metamorphic basement of some Southalpine, Austroalpine and Helvetic-Dauphinois units.
3) The Silurian-Lower Carboniferous continental collision (classic Variscan orogeny) generated crustal thickening by nappe stacking, low- to high-grade regional metamorphism in relaxed or thermally perturbed conditions, pervasive anatectic processes, post-nappe deformations, flysch deposition, and syn-orogenic igneous activity (350-320 Ma; Compagnoni et al., this volume). From the Late Carboniferous, the collapsed Variscan belt was sealed by clastic deposits (Hercynian unconformity) and intruded by post-orogenic plutons.
Permian-Mesozoic evolution
The Variscan plate convergence ended around the Carboniferous-Permian boundary, when transcurrent and transtensive tectonics became dominant on the scale of the Eurasian plate (Muttoni et al., 2009; Gaetani, this volume). Asthenosphere upwelling, thermal perturbation and lithosphere attenuation marked the Lower Permian onset of a new geotectonic regime in the future Adriatic domain. The Permian evolution was characterized, on a lithospheric scale, by extensional detachments, asymmetric extension (with Adria as upper plate) and widespread igneous activity from asthenospheric sources. In the Austroalpine and Southalpine basement, igneous activity began with underplating of Lower Permian gabbro batholiths, emplaced below and inside rising sections of attenuated continental crust, and then recrystallized in granulitic conditions. The overheated roofing crust generated anatectic melts which partly migrated to upper crustal levels. This cycle is also recorded by shallower granitoids and fault-bounded basins filled by clastic sediments and volcanic products.
A calc-alkaline to shoshonitic igneous pulse developed in the Middle Triassic, mainly and better preserved in the Southern Alps, and was produced by partial melting of previously enriched mantle sources (Variscan subduction) during lithosphere attenuation (Piccardo, this volume). From the Late Triassic, continental rifting between Adria (Africa) and Europe generated the Alpine Tethys, a deep-water seaway marked by listric faults, half-grabens and syn-rift deposits. Rifting ended at the Middle-Upper Jurassic boundary when the Mesozoic ocean began to spread. This age is constrained by deposition of radiolarian cherts on subsiding continental blocks in late syn-rift Early Bajocian times, and then developed in the oceanic crust from the Middle Bathonian onwards, nearly coeval with the oldest occurrences in the Central Atlantic. The Austroalpine-Southalpine domains became parts of the Adriatic continental passive margin, opposite to the European margin formed by the Penninic and Helvetic-Dauphinois domains. The subsidence history of the Adriatic margin is well recorded in the sedimentary successions of the Northern Calcareous Alps and the less deformed Southern Alps. The subsidence history of the European margin is recorded in the Prealpine klippen, the metamorphic Briançonnais cover, and the better preserved Helvetic-Dauphinois sedimentary sequences.
Continental rifting was probably generated by simple shear mechanisms, with Europe as extensional upper plate (opposite to the Permian setting). The continent-to-ocean evolution is particularly complex. From some central and western Alpine ophiolites, the local exposure on the ocean floor of a denuded and altered peridotitic basement (e.g., Malenco, Arosa and Aosta areas) may be envisaged. This hypothesis is corroborated by ophicarbonate breccias, continental detritus or cherts and deep water sediments deposited on top of mantle serpentinites, recalling modern exposures along fracture zones and ocean-continent transitions. In this view, coherent continental remnants of the extremely thinned extensional upper plate may have been lost inside the Tethyan ocean, as isolated allochthons and potential sources for some Western Austroalpine and Penninic continental nappes presently inserted between ophiolitic units. As previously seen, other units recall either fragments of normal oceanic lithosphere, or tectonic slices and olistoliths of oceanic suites inside dominant turbiditic deposists.
Restoration of the Tethyan ocean is a long and intriguing problem, mainly due to the occurrence of multiple ophiolitic horizons inside the collisional zone. Indeed, this complex multilayer may represent two or more oceanic branches, or merely the ultimate result of orogenic dispersal by polyphase folding, ductile shear and transposition. The Piedmont zone is the largest ophiolite in the Alps. It extends over most of the western Alps and, also named South-Penninic, reappears beyond the Ossola-Tessin window in the central (Malenco-Avers, Platta) and eastern Alps (Glockner, Rechnitz), below the eastern Austroalpine. Minor ophiolites, generally associated with flysch-type metasediments, are located at lower structural levels, mainly in the outer-lower Penninic domain from the north-western (Ossola-Tessin) to the central Alps (Grisons) and Engadine window, whereas the ophiolitic Versoyen (Valais) unit has recently lost its oceanic igneous nature and Mesozoic age. De-imbrication of the nappe stack in the Central Alps suggests that these ophiolitic units are derived, respectively, from the Piedmont-Ligurian (S-Penninic) ocean and a northern basin (N-Penninic, Valais), supposedly separated by the Briançonnais microcontinent or exotic terrane. Alternative reconstructions include i) a single Jurassic ocean with ribbon continents and/or variously-sized extensional allochthons, or ii) younger (Cretaceous) opening of the N-Penninic basin, partly contemporaneous to the closure of the Piedmont ocean.
Alpine orogeny
The Alpine orogeny first operated in the Eastern Austroalpine and then extended to the entire Alpine Tethys, gradually progressing from internal to external domains.
The earliest Alpine orogeny developed in the Eastern Austroalpine and was accomplished before the deposition of the Late Cretaceous Gosau beds: it has been related to the closure of a western branch of the Triassic Meliata ocean, extending to the Eastern Austroalpine domain through the Carpathians, and leading to a pre-Gosau continental collision. This reconstruction does account for the eclogitic (subduction) to Barrovian (collisional) metamorphism of eoalpine (Early-mid Cretaceous) age and wedge generation, although the oceanic suture is poorly preserved and the axial trend of the Triassic ocean (parallel or transversal to the future Alpine belt) is uncertain.
The subsequent orogeny developed in the entire Alpine belt from the Late Cretaceous (Western Austroalpine) onwards, and was closely related to the subduction of Piedmont (South-Penninic) oceanic lithosphere below the Adriatic active continental margin, leading to Eocene collision between Europe and Adria. The first stage of Alpine contraction was dominated by a subduction-related low thermal regime which initiated with the onset of oceanic subduction (Mid Cretaceous ?): this is revealed by the oldest (Late Cretaceous) high-P peak in the western Austroalpine, and lasted until the Eocene syncollisional subduction of the distal (pre-Piedmont) European margin, clearly recorded by the eclogitic to blueschist imprint in the upper and middle Penninic continental units. This stage was characterized by the growth of a pre-collisional to collisional (Austroalpine-Penninic) wedge at the Adria active margin. Since the beginning, it was devoid of a proper lithospheric mantle, being first underlain by the subducting oceanic lithosphere and, after ocean closure, by the passive margin of the European lower plate undergoing syncollisional subduction and accretion. The evolution of the Cretaceous-Eocene Alps has been alternatively interpreted to reflect: i) accretion of delaminated fragments of lithospheric microcontinents separated by oceanic channels; ii) tectonic erosion of the Adriatic active margin; iii) accretion, by tectonic underplating, of originally thin crustal fragments resulting from an extended or hyper-extended upper plate margin (ocean-continent transition). Exhumation of the high-P Penninic nappes was allowed by periodic extension in the wedge suprastructure, associated with nappe accretion at depth under active plate convergence.
From the Late or Latest Eocene (in differing areas), the subduction-related cool regime was replaced by relaxed and perturbed thermal conditions. Indeed, the high-P units of the subduction complex were rapidly and progressively exhumed to shallower structural levels and overprinted by a Barrovian metamorphism of Late Eocene-Lower Oligocene age, characterized by high thermal gradient (35 to 50°C/km; Frey et al., 1999). Soon after, a post-collisional magmatic cycle widely developed and was rapidly exhausted during the Oligocene (32-30 Ma). It is recorded along the Periadriatic fault system (Bigi et al., 1990). Older magmatic products (42-37 Ma) occur in the central-southern part of the Adamello batholith. The Periadriatic magmatism was generated by partial melting of lithospheric mantle sources previously enriched by fluids released by the subducting lithosphere during the Cretaceous-Eocene, and its generation is generally referred to slab break-off and related thermal perturbation, coupled with extension and rapid uplift of the wedge during active plate convergence. The Periadriatic magmatism ceased at the Oligocene-Miocene boundary, when renewed collisional shortening disactivated the magmatic sources.
The continuing plate convergence progressed and was accomodated mainly through accretion both in the foreland and interland, coupled with ultimate extrusion and cooling of the Austroalpine-Penninic wedge. The Helvetic basement slices and decollement cover nappes were accreted to the Austroalpine-Penninic wedge and displaced over the sinking Molasse foredeep, whereas an opposite-vergent thrust-and-fold belt developed in the Southalpine upper crust, mainly generated by indentation of the Adria mid-lower lithosphere into the wedge rear. In the meantime, the overthickened Austroalpine-Penninic nappe stack underwent orogen-parallel tectonic denudation along low-angle detachments (e.g., Ratschbacher et al., 1991).
At a lithospheric scale, the mature collisional belt continued to maintain its asymmetry. Seismicity, GPS measurements and foreland subsidence provide evidence of a still active Alpine contraction and tectonic extension (e.g., Sue & Tricart, 2003; Delacou et al., 2004; Chiarabba et al., 2005; Sue et al., 2007; Cuffaro et al., 2010; Devoti et al., this volume).