Tyrrhenian Sea and the Italian Peninsula
Introduction
Orogenic magmatic activity in the Tyrrhenian Sea and the Italian peninsula took place from the Miocene to the present. There is a temporal continuum from the Oligocene to Miocene magmatism of Sardinia to the orogenic magmatic cycle developed in the Tyrrhenian basin and the Italian peninsula, the all becoming younger southeastward (Table 1). Within single areas, orogenic magmatism is spatially associated with MORB-type to Na-alkaline anorogenic rocks (see Bianchini & Beccaluva, this issue), the latter being younger than orogenic activity. The orogenic magmas are directly related to the south-eastward rollback of the NW-dipping Adriatic-Ionian subduction zones, whereas anorogenic activity reflects backarc extension processes, which follow subduction hinge retreat and are responsible for the opening of the Tyrrhenian basin and the anticlockwise rotation of the Italian peninsula.
Orogenic magmatism in the Tyrrhenian Sea and the Italian peninsula shows extremely variable petrological and geochemical compositions, ranging from arc tholeiitic to calcalkaline, shoshonitic and potassic alkaline (Peccerillo, 2005a). Based on age of emplacement, petrological affinity, and geochemical characteristics of volcanic rocks (Tables 1, 2), several magmatic provinces or zone have been distinguished (see Fig. 41, 42; Peccerillo, 2002). These zones are:
1 – TYRRHENIAN SEA BASIN
a – Central Tyrrhenian Sea floor and seamounts
b – Aeolian Arc and associated seamounts
2 – ITALIAN PENINSULA
a – Mount Vulture, Campanian Province and the Pontine Islands
b – Ernici-Roccamonfina
c – Latium Province or Roman Province s.s.
d – Intra Apennine Province (IAP)
e - Tuscany Province
Tyrrhenian Sea Basin
The Tyrrhenian Sea is an extensional basin developed between about 15 Ma and present, between Corsica-Sardinia block and the Apennine-Maghrebide collisional chain (Carminati et al., this volume). The triangular shape of the Tyrrhenian Sea is the result of a variable degree of extension, which increases from north to south. A major W-E trending lithospheric structural discontinuity crosses the Tyrrhenian Sea. This is known as the 41st Parallel Line (Fig. 20), and continues inland across the Italian peninsula (e.g., Serri, 1990; Bruno et al., 2000). As a consequence of variable extension, the nature and thickness of the crust and the intensity of igneous activity are variable in the southern and in the northern Tyrrhenian Sea. Crustal thickness in the southern Tyrrhenian Sea ranges approximately between 20 to 25 km to less than 10 km, going from the basin margins to the Marsili and Vavilov basins. Moreover, magmatic activity is much more intense in the southern than in the northern Tyrrhenian Sea.
Figure 20. Tyrrhenian Sea magmatism
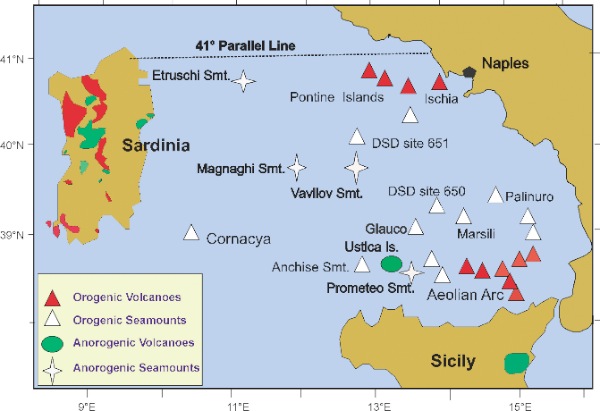
Distribution of orogenic volcanism in the Tyrrhenian Sea basin. Some anorogenic volcanoes and seamounts are also reported.
The upper mantle beneath the Tyrrhenian Sea basin is characterised by occurrence of a 20-30 km thick layer with relatively low values of S-wave velocity (Vs ~ 4.0 to 4.2 km/s). This extends westward beneath Sardinia and the Balearic Sea at a depth of about 80-120 km, and rises to shallower level in the southern and southeastern Tyrrhenian basin (Panza et al., 2007; Peccerillo et al., 2008a; Frezzotti et al., 2009). The occurrence of such a layer has been interpreted as an effect of occurrence of compositionally anomalous mantle rocks, left behind the Ionian-Adriatic slab during its Oligocene to present southeastward retreat.
Igneous activity within the Tyrrhenian Sea basin is variable in age, intensity and composition. In the north, igneous rocks are scarce and belong to the Tuscany Magmatic Province, which will be described later in this paper. In the central-southern sectors, a wide variety of magmas were erupted from Miocene to present. These include three broad groups, which have MORB, OIB and island-arc geochemical signatures. The latter consist of several submarine centers plus the exposed volcanoes of the Aeolian Arc. Although treated separately, there is a continuum in the orogenic activity from Sardinia to the central Tyrrhenian basin and the Aeolian Arc, as a consequence of continuous retreat of the Ionian subduction zone toward the south-east.
Tyrrhenian Sea floor
Orogenic volcanic centres on the Tyrrhenian Sea floor have been found at several places. Older occurrences are Cornacya (12 Ma), Anchise seamount (5.2 to 3.6 Ma), ODP sites 650 and 651 (3 to 1.7 Ma). Marsili (0.8 to present) and other Aeolian seamounts (Sisifo, Enarete, Eolo, Lametini, Alcione, Palinuro) are located in the southeastern sector of the Tyrrhenian basin (Figs. 20, 22). There is a decreasing in age from west to southeastesrn Tyrrhenian basin, where active volcanoes are present.
Figure 21. TAS of Tyrrhenian Sea orogenic seamounts
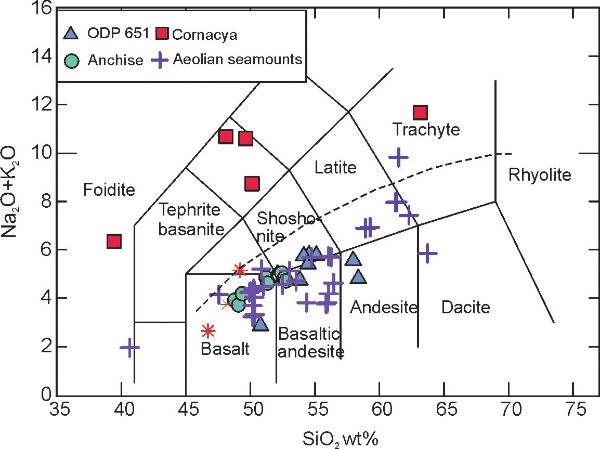
Total Alkali vs. Silica (TAS) diagram for Tyrrhenian Sea floor orogenic volcanoes. Data have been recalculated on a water-free basis. The dashed line is the boundary between subalkaline and alkaline magmas (Irvine & Baragar, 1971).
The TAS diagram based on oxide concentrations recalculated on a water-free basis for some occurrences is shown in Fig. 21.
Cornacya is an about 12 Ma old volcano located SE of the southern Sardinian coast, and consisting of strongly altered (LOI up to about 23 wt %) lavas that contain enclaves of mica-rich lamprophyres. Lava textures are porphyritic with phenocrysts of plagioclase and biotite and minor amphibole and clinopyroxene, surrounded by an altered glassy matrix containing Na-rich plagioclase, anorthoclase, biotite, and secondary products. The lamprophyric enclaves are porphyritic with phenocrysts of altered olivine, amphibole and phlogopite. A shoshonitic to ultrapotassic lamproitic affinity is indicated by immobile element contents for lavas and enclaves, which respectively resemble shoshonites and lamproites from Tuscany (Mascle et al., 2001).
ODP 651 Site is characterised by basaltic lava and sill associated with sediments and serpentinised peridotites (Bonatti et al., 1990). 40Ar/39Ar dating indicates an age of approximately 3.0 to 2.6 Ma (Feraud, 1990). Volcanic rocks have a microcrystalline to moderately porphyritic texture with microphenocrysts of altered olivine, plagioclase, clinopyroxene and some biotite (Bertrand et al., 1990). Secondary phases such as clay minerals, zeolites, Fe-hydroxides and carbonates are common. Incompatible element patterns of lavas and radiogenic isotope signatures show affinities with the calcalkaline (CA) and high-K calcalkaline (HKCA) rocks from Stromboli (Beccaluva et al., 1990).
ODP 650 Site (Marsili basin) contains basaltic rocks beneath an approximately 600 m thick pile of sediments. Magnetostratigraphic and biostratigraphic age for the base of sedimentary pile is 1.9 to 1.7 Ma. Volcanic rocks consist of vesicular altered basalts, containing plagioclase, olivine, clinopyroxene and altered glass mesostasis. Petrogenetic affinity of these rocks is uncertain, because of severe alteration, although a similarity with Stromboli calcalkaline basaltic andesites has been recognised by Beccaluva et al. (1990) on the basis of incompatible element ratios.
Central Tyrrhenian arc. Volcanic occurrences forming a sort of arc crossing the entire central-eastern Tyrrhenian Sea, from offshore north-western Sicily to the western Pontine islands and the Neapolitan area, have been suggested to represent an unique structure (Locardi, 1986). Volcanic centres include the Anchise seamount in the south, some volcanoes in the central Tyrrhenian Sea (Site 650 and Glauco), the buried andesitic rocks of the Campanian plain (Parete-2 well), and the volcanic island of Ponza and surrounding islets. Data are available only for some occurrences. Compositions range from mafic to felsic, the latter being concentrated at Ponza and surrounding islets.
Anchise seamount is a 5.2 to 3.6 Ma volcano (Savelli, 1988) situated west of the Na-alkaline island of Ustica. Collected lavas have a vesicular porphyritic texture, with phenocrysts of clinopyroxene, plagioclase, and minor olivine surrounded by a groundmass of the same phases plus Fe-Ti oxides, sanidine and minor biotite and glass (Calanchi et al., 1984). Major element data indicate a mafic high-K calcalkaline to shoshonitic (SHO) composition, whereas the few available trace elements exhibit enrichment in Rb and depletion in Ti, Nb and Zr.
Ponza, Palmarola and Zannone make up the western sector of the Pontine Archipelago. Exposed products consist of rhyolitic to trachytic obsidian lava flows and domes, dikes, and pyroclastic deposits. K/Ar dating on sanidines indicates ages of 4.2 and 3.0 Ma for Ponza rhyolites, 1.0 Ma for trachytic activity from the same island, and 1.6 Ma for Palmarola (Cadoux et al., 2005; Cadoux et al., 2007). Rhyolites are calcalkaline in composition, although some late-erupted products are peralkaline (Conte & Dolfi, 2002).
Major, trace element and isotopic compositions of western Pontine islands are variable with peralkaline rhyolites strongly enriched in Rb, Th and other incompatible elements. REE patterns are fractionated, with negative Eu anomalies. 87Sr/86Sr are about 0.7085 to 0.7104 in the trachytes and about 0.7105 in the rhyolites. Nd isotopic ratio is higher in the trachytes (143Nd/144Nd ~ 0.51240) than in the rhyolites (143Nd/144Nd ~ 0.51225; Conte and Dolfi, 2002). Pb isotopic ratios are homogeneous (206Pb/204Pb ~ 18.80, 207Pb/204Pb ~ 16.80, and 208Pb/204Pb ~ 39.00; Cadoux et al., 2007). Oxygen isotope composition from whole rocks and separated feldspars ranges from δ18O ~ +7.3 to + 11.1, showing an increase from trachytes to rhyolites (Turi et al., 1991). Rhyolites from the western Pontine Islands have a composition suggesting a derivation from a basalt or andesite parent by fractional crystallisation plus crustal assimilation (AFC). The same processes have been suggested for generation of trachytes, but a moderately potassic alkaline parents has been suggested (Conte & Dolfi, 2002; Cadoux et al., 2005). This has led to conclusion that the western Pontine islands experienced a transition from calcalkaline to potassic alkaline volcanism from early to late exposed activity (Conte & Dolfi, 2002; Cadoux et al., 2005).
Parete-2 volcanics have been encountered by deep drilling in the Campanian Plain, north of the Campi Flegrei caldera. Here, a large calcalkaline basalt to andesite volcano occurs at depths of 300 to 1900 m, beneath the potassic alkaline suite of Campi Flegrei (Di Girolamo et al., 1976; Barbieri et al., 1979; Albini et al., 1980). The lavas at the bottom of the drilled volcanic sequence have an age around 2 Ma. Rocks are porphyritic with phenocrysts of plagioclase, clino- and orthopyroxene and a few biotite. Sr isotopic ratios are relatively radiogenic and overlap the composition of Vesuvio and Campi Flegrei.
Overall, the Central Tyrrhenian arc could represent a remnant volcanic arc left behind by the retreating Ionian slab (Savelli, 1988, 2001; Argnani & Savelli, 1999). In the south, activity shifted toward the east and concentrated in the Marsili basin and the Aeolian Arc. In the northern end, activity also shifted partially to the east, but displacement was much smaller, because of the lower degree of extension of the Tyrrhenian basin. As a result the superimposition of subalkaline and potassic alkaline volcanism occurred in some areas, such as the western Pontine islands and especially in the Campi Flegrei area.
Aeolian Arc and related seamounts
The Aeolian Arc has developed over a continental crust of the Calabro-Peloritano basement, a fragment of the European plate, which detached from the Corsica-Sardinia block and migrated southeastward during the Miocene to Quaternary opening of the Tyrrhenian Sea. The Aeolian Arc consists of large volcanoes forming seven main islands and several seamounts (Fig. 22). Age of volcanic activity exposed at the surface goes from about 400 ka to the present (Gillot, 1987).
Figure 22. Aeolian arc and related seamounts
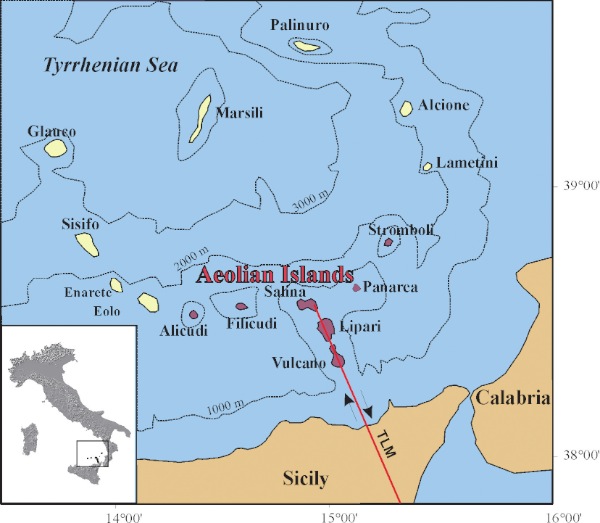
Distribution of Aeolian islands and seamounts. TLM is the Tindari-Letojanni-Malta fault, separating the western and eastern sectors of the arc.
Rocks show a wide range of compositions, from mafic to silicic with calcalkaline (CA) to shoshonitic (SHO) and potassic alkaline affinities (Fig. 23) (e.g., Keller, 1982; Francalanci et al., 2004; Peccerillo, 2005a). A few rocks with an arc tholeiitic composition have been found among dredged samples along seamounts (Beccaluva et al., 1982). Textures are generally porphyritic with ubiquitous plagioclase and clinopyroxene phenocrysts plus olivine in the mafic rocks and orthopyroxene in the calcalkaline intermediate lavas. Biotite and amphibole is present in some intermediate rocks, whereas sanidine appears in the rhyolites. Leucite is observed in some mafic to intermediate potassic rocks from Vulcano and Stromboli. Various types of xenoliths are found in the Aeolian Arc rocks, including fragments from the basement and cumulate igneous rocks. Small ultramafic xenoliths have been found at Alicudi and Stromboli (Peccerillo et al., 2004; Laiolo & Cigolini, 2006).
Three main sectors have been distinguished in the Aeolian Archipelago, showing distinct compositions of volcanic products, ages and volcanological-structural features (Peccerillo, 2005a). The western sector is formed by the islands of Alicudi and Filicudi along a E-W trending fracture system, and has an age of about 0.4 Ma to 20 ka (Keller, 1980; Gillot, 1987). Volcanic rocks mostly consist of mafic to intermediate rocks with a calcalkaline to high-K calcalkaline affinity. A few dacites occur at Filicudi. Rocks show the most primitive compositions over the entire Aeolian Arc, and basalts from Alicudi have Mg#, Cr and Ni abundances not far from values of mantle equilibrated melts. Seismic activity is restricted to the upper 20 km in this sector, and no deep earthquakes have been detected.
Figure 23. Alkali-silica diagrams, Aeolian Arc
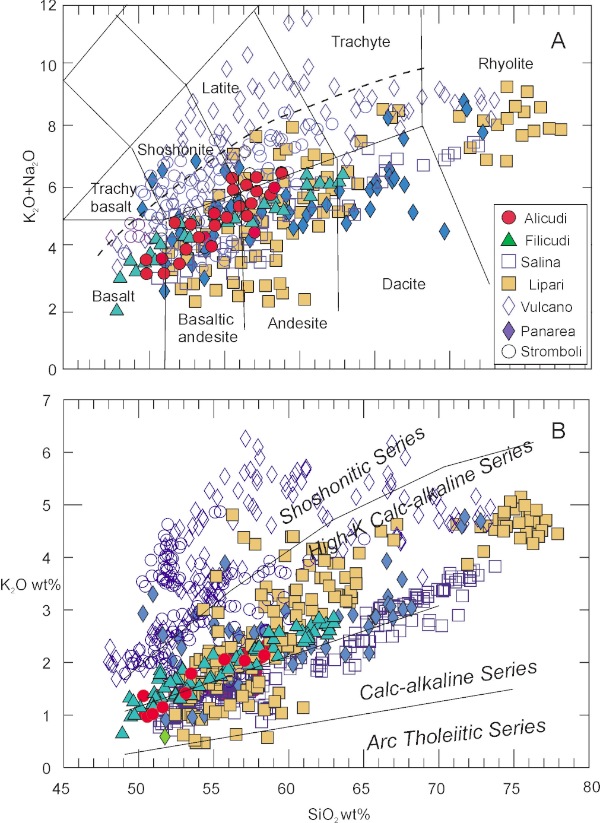
A - TAS and B - K2O vs. SiO2 classification diagrams for the Aeolian Arc volcanics. The dashed line on TAS diagram is the boundary between subalkaline and alkaline magmas (Irvine & Baragar, 1971).
The central sector is formed by the islands of Salina, Lipari and Vulcano. The latter islands are aligned along the NNW-SSE striking Tindari-Letojanni-Malta (TLM) fault system (Fig. 22), a major dextral strike-slip lithospheric fault cutting the Aeolian Arc and running until eastern Sicily and the Malta escarpment. Salina is at the intersection between TLM fault and the western arc tectonic structure. The exposed rocks in the central islands have an age between about 0.4 Ma to present, and have been erupted by both effusive and explosive activity. Youngest activity at Lipari took place at about 580 AD, whereas the latest eruption at Vulcano occurred from 1888 to 1890 AD (Mercalli & Silvestri, 1891). Volcanism developed within pull-apart basins along the main fault system. Rock composition ranges from basaltic to rhyolitic, with CA, HKCA and SHO affinity. Some leucite-bearing potassic alkaline rocks occur at Vulcano. Rhyolitic magmas are typical of central Aeolian islands and are very abundant at Lipari and Vulcano. Temporally, silicic magmas are restricted to the last 40 ka. Seismicity is still shallow and concentrated along the Tindari-Letojanni-Malta fault.
The eastern sector is formed by Panarea and Stromboli, developed along NE-SW faults. The exposed rocks have ages between about 0.2 Ma and present. Rock compositions range from mafic to silicic, and have a calcalkaline, shoshonitic to potassic alkaline affinity. Calcalkaline-shoshonitic intermediate to rhyolitic rocks form the bulk of the Panarea Island and surrounding islets. Stromboli rocks are mafic to intermediate in composition and range from calcalkaline to potassic alkaline, with an irregular increase of potassium with time. The present-day activity erupts shoshonitic basalt strombolian scoriae and lava flows. Deep seismic activity occurs beneath the eastern arc, with earthquake foci defining a narrow and steep NNW dipping Benioff plane that extends up to the Neapolitan area where about 500 km deep earthquakes have been detected (e.g., Falsaperla et al., 1999; Panza et al., 2003, 2004).
Alicudi consists of calcalkaline basalt, basaltic andesite and andesite lava flows and domes, and minor pyroclastics (Fig. 23a). Incompatible element patterns are fractionated and show negative anomalies of HFSE, and small positive spikes of Sr and Pb (Peccerillo et al., 2004; Fig. 24a). Sr isotope compositions are the lowest in the Aeolian Arc (87Sr/86Sr = 0.70343 to 0.70406), and decrease from basalt to andesites. Nd isotope ratios are the highest (143Nd/144Nd = 0.51280 to 0.51290; Fig. 25a), and show the usual negative correlation with Sr isotopes. Pb-isotope ratios are moderately radiogenic (206Pb/204Pb ~ 19.19 to 19.67; 207Pb/204Pb ~ 15.62 to 15.67; 208Pb/204Pb ~ 39.07 to 39.36; Fig. 25b), and slightly increase from basalts to andesites. Oxygen isotope ratios on separated clinopyroxenes range between δ18O ~ +5.0 to +5.6, with a rough positive correlation with 87Sr/86Sr (Peccerillo et al., 2004). He-isotope composition measured on olivine and pyroxene phenocrysts has R/RA around 6.5 to 7.1 (Martelli et al., 2008).
Figure 24. Spider-diagrams, Aeolian Arc
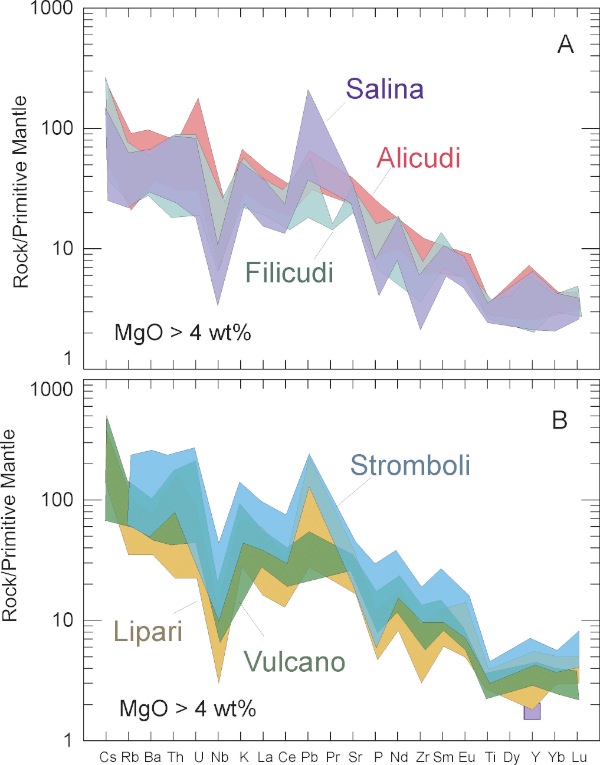
Incompatible element patterns normalized to primordial mantle composition for mafic rocks (MgO > 4%) from the Aeolian Arc.
Filicudi consists of calcalkaline basalt to high-K calcalkaline andesite and dacite lava flows, domes, and pyroclastic products. Mantle-normalised incompatible trace element patterns of mafic rocks are similar to Alicudi, whereas Sr-isotope ratios (87Sr/86Sr ~ 0.70401 to 0.70474) are slightly higher and Nd-isotope ratios lower (143Nd/144Nd = 0.51267 to 0.51276). Pb-isotope are in the range 206Pb/204Pb ~19.31 to 19.67; 207Pb/204Pb ~ 15.64 to 15.69; 208Pb/204Pb ~ 39.11 to 39.47 (Fig. 25; Santo et al., 2004).
Salina is sited at the intersection between the E-W trending Alicudi-Filicudi alignment and the Tindari-Letojanni-Malta fault system. The island is characterised by the two well-preserved symmetrical cones of Monte Felci and Monte Porri, which were constructed upon older products of the eroded Rivi, Capo and Corvo volcanoes. Age of the exposed products ranges between about 400 to 13 ka (Keller, 1980; Gillot, 1987).
Rocks range in composition from CA basalts to rhyolites (Fig. 23). Trace element abundances are variable, and LILE/HFSE ratios (e.g., Ba/Ta, La/Nb, Th/Ta) are generally higher than observed in other Aeolian magmas (Fig. 24a).
Sr-isotope ratios (0.70397 to 0.70507) and oxygen isotope ratios on whole rocks (δ18O‰ = +6.4 to +8.5) increase slightly with decreasing MgO (Gertisser & Keller, 2000). Nd isotopes (0.51265 to 0.512815) and Pb isotopes are moderately radiogenic (206Pb/204Pb ~ 19.30 to 19.66, 207Pb/204Pb ~ 15.61 to 15.77, 208Pb/204Pb ~ 39.15 to 39.51) (Fig. 25).
Lipari is the largest of the Aeolian islands. Exposed products have an age ranging from about 220 ka to late Roman times (about 580 AD). Rock compositions range from CA basaltic andesite to rhyolite (Fig. 23). Early activity erupted basaltic andesitic and andesitic lavas and pyroclastic products. These were followed by high-K andesites and minor dacites. Strongly explosive eruptions from centres located between Lipari and Vulcano (70 to 13 ka) deposited high-K calcalkaline to shoshonitic pyroclastic products (the so-called Brown Tuffs). Younger activity gave acidic obsidian lava flows, domes and pumices.
Major and trace element variations show scattering, and distinct variation trends for some trace elements have been found (e.g., Crisci et al., 1991). Incompatible element patterns are fractionated with negative anomalies of HFSE and positive spikes of Sr and Pb in the mafic rocks (Fig. 24b). Sr, Nd and Pb isotopic compositions (87Sr/86Sr = 0.7043 to 0.7067; 143Nd/144Nd = 0.51276 to 0.51242; 206Pb/204Pb = 18.46 to 19.63; 207Pb/204Pb = 15.61 to 15.71; 208Pb/204Pb = 39.05 to 39.43) exhibit strong variations indicating significant interaction with crust, a process that is testified by the occurrence of andesitic rocks containing xenocrysts of cordierite, garnet and sillimanite (Barker, 1987; Di Martino et al., 2011).
Vulcano is the second largest island and one of the active centres of the archipelago. It consists of several superimposed volcanic centres, with two large intersecting calderas. Exposed activity started in the southern sector of the island where a stratovolcano (Primordial Vulcano) with a central caldera was constructed. Successively, the activity shifted northward where a second caldera collapse formed (Caldera della Fossa). Historical and present activity is concentrated in the centre (La Fossa Cone) and at the northern border (Vulcanello) of the Fossa caldera. The latest eruption took place at La Fossa Cone in 1888-1890 and was characterised by explosive emplacement of abundant juvenile and accessory pyroclastics. This eruption, later used to define “vulcanian” explosions was carefully described by Mercalli & Silvestri (1891) in a paper, which is considered as a milestone of descriptive volcanology.
Rock compositions at Vulcano range from mafic to felsic, with high-K calcalkaline to shoshonitic and potassic alkaline (Potassic Series: KS) affinities (Fig. 23b). Calcalkaline rocks are lacking among the outcropping products. As at Lipari, there is an increase in silica with time. However, enrichments in potassium and incompatible elements are stronger at Vulcano and potassic alkaline rocks containing leucite are erupted especially during historical activity at Vulcanello, at the northern tip of the island.
HKCA rocks (Primordial Vulcano) have lower incompatible element abundances than younger shoshonitic and potassic products (De Astis et al., 1997). Patterns of incompatible elements of mafic rocks show similar shape but overall higher element abundances than at Lipari (Fig. 24b). Sr-isotope ratios range from 0.7042 to 0.7059. When only the most primitive rocks are considered, HKCA rocks from Primordial Vulcano show less radiogenic Sr isotopic compositions than younger SHO and KS rocks (De Astis et al., 1997). He-isotope composition measured on olivine and pyroxene phenocrysts gave values of R/RA ~ 2.3 to 4.9 (Martelli et al., 2008).
Panarea and surrounding islets are the emergent remnants of a large composite volcano rising about 1700 m above sea floor and forming a flat platform at a depth of about 100-150 m below sea level. Exposed rocks have a dominant CA to HKCA andesitic, dacitic and rhyolitic composition, but a larger range of magma types is evidenced by lithic clasts that reveal the occurrence of shoshonitic basalts and shoshonites. Ages range between about 150 and 45 ka.
Major and trace element compositions of Panarea volcanics show an increase of incompatible element contents from calcalkaline to HKCA and SHO rocks at comparable degree of evolution (Calanchi et al., 2002). Mantle normalised incompatible element patterns show high LILE/HFSE ratios and a positive spike of Pb. Sr isotope ratios (87Sr/86Sr = 0.7040 to 0.7057) increase from calcalkaline to shoshonitic rocks, whereas the few available Nd and Pb isotopic data do not show any significant trend (143Nd/144Nd = 0.51284 to 0.51257; 206Pb/204Pb = 18.18 to 19.43; 207Pb/204Pb = 15.67-15.71; 208Pb/204Pb = 39.13-39.36). Overall, Panarea calcalkaline rocks have isotopic signatures and incompatible trace element ratios that are similar to those of the western Aeolian Arc. In contrast, geochemical and isotopic compositions of HKCA and SHO rocks are midway between the western arc and Stromboli.
Stromboli and the nearby islet of Strombolicchio represent the top of a structure that rises about 2000 m above the sea floor, and reaches 924 m above sea level. Age of the outcropping rocks is about 200 ka at Strombolicchio and between about 100 ka and present at Stromboli (Condomines & Allegre, 1980; Gillot, 1987; Gillot & Keller, 1993). Compositions range from mafic to intermediate, with calcalkaline and high-K calcalkaline to shoshonitic and potassic alkaline (KS) affinities (Francalanci et al., 1993a). Calcalkaline rocks are minor with respect to dominant HKCA, SHO and KS products. KS rocks contain leucite. Overall, there is an increase in potassium with time, but with many irregularities. The present-day activity erupts shoshonitic basaltic lavas and scoriae by continuous "strombolian" mild explosions, and occasional outpouring of lava flows (Rosi, 1980; Francalanci et al., 1989; Bertagnini et al., 2003). Craters are located on a terrace at the top of a large depression bounded by parallel faults, known as Sciara del Fuoco.
In general there is an increase in incompatible element abundances from CA to HKCA, SHO and KS rocks, along with increase in potassium. Sr-isotope ratios (87Sr/86Sr = 0.70500 -0.70757) also increase the same way, reaching the most radiogenic compositions in the KS. 143Nd /144Nd (= 0.51265 to 0.51243) decreases with increasing potassium. Pb isotopic compositions are less radiogenic than at other Aeolian islands (206Pb/204Pb = 18.93 to 19.09; 207Pb/204Pb = 15.64 to 15.70; 208Pb/204Pb = 39.01 to 39.16; Fig. 25). Incompatible element patterns show moderate HFSE negative anomalies (Fig. 24). He-isotope compositions show R/RA ratios of about 2.4 to 4.6, the lowest in the Aeolian Arc (Martelli et al., 2008). In general, the Stromboli rocks have very similar radiogenic isotope compositions and ratios of several incompatible elements as those from the Campania volcanoes (Peccerillo, 2001a).
Figure 26. Central Italy volcanoes
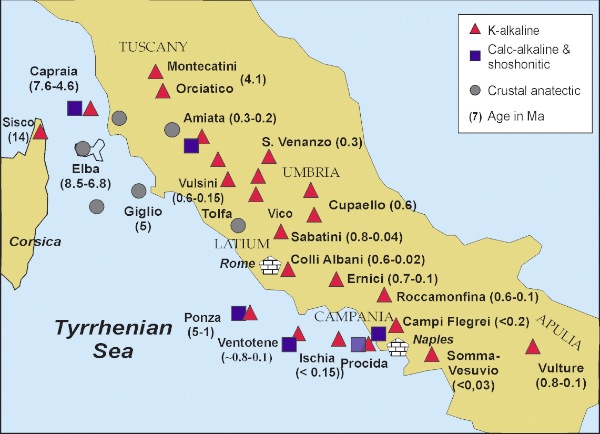
Distribution of orogenic magmatism in central Italy. Note that ages, indicated in parentheses, decrease souteastward.
Aeolian Seamounts
There are several seamounts located to the northwest (Sisifo, Enarete, Eolo) and to the northeast (Lametini, Alcione, Palinuro) of the Aeolian Archipelago, defining a horseshoe-type pattern around the Marsili volcano and basin (Fig. 22). Another center has been recently discovered offshore Calabria (De Ritis et al., 2010), and has been suggested to be the source of pyroclastic rocks commonly found in Callabria. Geochronological data of Beccaluva et al. (1985) and Trua et al. (2002, 2004) indicate ages from 1.3 to less than 0.1 Ma for Aeolian seamounts. Based on available data, compositions are mostly calcalkaline (Marsili, Sisifo, Eolo, Palinuro, Alcione) to shoshonitic (Enarete, Eolo, Sisifo), with a few arc tholeiitic basalts (Lametini and southern Marsili basin).
Marsili is the best studied of the Aeolian seamounts. It is huge (about 60 km long) NNE-SSW elongated structure, rising about 3000 m above the center of the Marsili basin, an area affected by extremely high degree of extension (Nicolosi et al. 2006). Rocks from Marsili seamount consist of calcalkaline basalts a few high-K andesites. Compositions of basalts reveal the occurrence of two types of primary magmas, exhibiting different enrichments in incompatible elements and LILE/HFSE ratios (Trua et al., 2002, 2004 and references therein). Sr- and Nd-isotope ratios show a wide range of values (87Sr/86Sr ~ 0.7032 to 0.7052; 143Nd/144Nd ~ 0.5127 to 0.5129), with the most radiogenic-Sr compositions being found in the rocks with higher LILE/HFSE ratios (Trua et al., 2004).
Petrogenesis of Aeolian Arc magmas
The Aeolian Arc volcanics have variable compositions both at local and regional scale. These can be summarised as follows:
1 – The islands of Alicudi, Filicudi and Stromboli, sited at the western and eastern ends of the arc, have compositions ranging from mafic to intermediate, with the absence of acid rocks. The islands of Panarea and Salina contain some rhyolites. Acid rocks become extremely abundant at Vulcano and Lipari, in the center of the archipelago. Rhyolitic activity starts during the latest stage of evolution of volcanism in all these islands.
2 – The western islands of Alicudi, Filicudi and Salina show a modest variation of potassium contents in mafic magmas, and are composed exclusively of calcalkaline to high-K calcalkaline rocks, with absence of shoshonites. At Lipari, Vulcano and the eastern islands of Panarea and Stromboli, shoshonitic magmatism is present along with calcalkaline and/or HKCA products. Leucite-bearing volcanics are found at Vulcano and Stromboli. Therefore, there is an increase in the range of potassium contents from west to east.
3 – Sr and Nd isotopic signatures respectively increase and decrease going from the western island of Alicudi to the central and eastern islands. At Stromboli, the highest Sr- and lowest-Nd isotopic ratios are observed. There is also decrease of He isotopic ratios from west to east, with R/RA decreasing from about 7 at Alicudi to about 2 at Stromboli.
5 – Abundances of incompatible trace elements increase the same way as potassium. Instead, ratios of incompatible trace element have more complicated patterns. For instance, LILE/HFSE ratios (e.g., La/Ta) show lower values at the extreme islands of Alicudi and Stromboli, with respect the central islands, especially Salina (Francalanci et al., 2004).
Increase in the amount of silicic rocks from external to central islands suggest that shallow level evolution processes of magmas had less intensity in the external islands. Occurrence of mafic to intermediate rocks at Alicudi, Filicudi and Stromboli suggests that magma evolution was modest and affected calcalkaline parental melts. Polybaric fractional crystallisation, accompanied by moderate interaction with the crust has been suggested for these two islands (Peccerillo et al. 2004; Santo et al. 2004; Bonelli et al. 2004).
Evolution processes in the central arc were more intensive that in the western islands, and rhyolitic compositions were reached. Acidic compositions were the result of complex processes dominated by fractional crystallisation plus mixing and crustal assimilation, starting from calcalkaline and shoshonitic magmas. Crustal contamination was more intense at Lipari, where some rocks contain significant amounts of crustal material (Barker, 1987; Di Martino et al., 2011). Mixing-mingling processes also played an important role (De Astis et al., 1997; Gioncada et al., 2003).
When mafic magmas over the entire Aeolian Arc are considered, one can observe several important variations of key geochemical parameters. K2O and LILE and 87Sr/86Sr in rocks with MgO > 5% increase from west to east, as mentioned earlier. However, a similar time-related increase of potassium and incompatible elements is also observed within single islands. It is unlikely that these variations depend only on crustal assimilation processes by the ascending magmas. This is excluded by the large amount of crust that would be necessary (more than 50-60%) to drive Sr isotopic compositions from values of about 87Sr/86Sr ~ 0.7035 - 0.704 found in the western and central islands to values of 87Sr/86Sr ~ 0.706 - 0.707 observed at Stromboli. Therefore, it has been concluded that the range of trace element and radiogenic isotopic compositions observed for mafic rocks both along the arc and within single islands, reflects those of the mantle sources. This points to strongly heterogeneous upper mantle both at regional scale and beneath some islands, especially Stromboli (e.g., Francalanci et al., 1993a,b, 2007).
Compositional heterogeneities in the mantle source of Aeolian Arc relate to different amounts and types of upper crustal material carried into the mantle wedge by subduction processes (Ellam et al., 1988; Francalanci et al., 1993b, 2007). Regional isotopic (Sr, Nd, Pb, He) variations suggest that whereas the mantle sources of western-central islands underwent input of fluids or melts coming from an oceanic-type slab, upper crustal material (subducted sediments) were important mantle contaminants beneath eastern islands (Ellam et al., 1988, 1989; Calanchi et al., 2002; Francalanci et al., 2007 and references therein). Variations of trace element and radiogenic isotope signatures within single islands, especially at Stromboli, would be related to vertical mantle heterogeneity, possibly generated by variable contamination of the peridotite column by sedimentary material from the Ionian subducted slab.
Francalanci et al. (1993b, 2007) highlighted significant along-arc variations of incompatible trace element ratios (e.g., Ba/Nb and La/Ta or other LILE/HFSE ratios). This was suggested to reveal a different role of transfer by fluid phases from slab to the mantle wedge. However, variable LILE/HFSE ratios may also indicate different types of pre-metasomatic mantle sources (Ellam et al., 1989; Peccerillo, 2001b).
In conclusion, the bulk of geochemical and radiogenic isotope data for mafic Aeolian Arc magmas support an increased role of sediments with respect to basaltic slab as contaminant of the mantle sources, going from west to the east. Element transfer from slab to mantle wedge was accomplished by melts and fluids, with the two agents having different roles in the various sectors of the arc (Francalanci et al., 1993b). It is still debated whether the pre-metasomatism mantle wedge had a OIB-type and MORB-type composition (Ellam et al., 1989; Trua et al., 2002, 2004, 2010; Francalanci et al., 2007).
Italian Peninsula
Extensive Plio-Quaternary orogenic magmatism occurs along the western border of the Italian peninsula and some Tyrrhenian Sea islands (e.g., Ischia, Pontine Islands, Tuscan Archipelago). Ages range from about 8 Ma to present, and decrease from north-west to south-east (Fig. 26). Petrological features are extremely variable, from calcalkaline to alkaline potassic and ultrapotassic. Based on major, trace element and radiogenic isotope signatures, several magmatic provinces have been distinguished (Peccerillo, 2002, 2005a), each showing peculiar compositional characteristics (Figs. 41, 42) volcanological features and structural setting (Peccerillo, 2002). Geophysical investigation also evidences different structure of the lithosphere-asthenosphere system in the different regions (Peccerillo & Panza, 1999; Panza et al., 2007). Staring from south, these provinces are:
1 – Mount Vulture
2 – Campania Province (SommaVesuvio, Campi Flegrei, Procida and Vivara, Ischia)
3 – Eastern Pontine islands (Ventotene and Santo Stefano)
4 – Ernici and Roccamonfina province
5 – Roman (Latium) Province s.s. (Alban Hills, Sabatini, Vico, Vulsini)
6 –Intra Apennine Province (IAP: San Venanzo, Cupaello and other minor centres)
7 – Tuscany Province.
Potassic and ultrapotassic compositions are typical of most rocks occurring in the Italian peninsula. These are discussed by Conticelli et al. (this issue). Therefore, their characteristics, origin and geodynamic significance will be only briefly described in this paper.
Mount Vulture
Mount Vulture is an isolated volcano sited east of the Apennine compression front, at the border of the Apulia foreland, a promontory of the African-Adriatic plate. It has been formed by explosive and effusive activity between about 0.8 Ma and 0.1 Ma. The stratovolcano is cut by a summit collapse caldera, which hosts two explosion craters. Rocks are undersaturated in silica, and range from tephrite, foidite, melilitite, to phonolite (Fig. 27; De Fino et al., 1984). Late carbonate-rich pyroclastics and lavas have been erupted (Stoppa & Principe, 1997; D’Orazio et al., 2007). Rock textures are generally porphyritic with various types and abundances of phenocryst phases, including olivine, clinopyroxene, amphibole, feldspars, leucite and haüyne. Haüyne is the most common foid.
Vulture rocks are variably enriched in alkalies (Fig. 27) with K2O/Na2O ~ 0.1 to 1.6. Incompatible element patterns show HFSE negative anomalies, positive spikes of Pb, but also relative depletions in Rb and K, which are not observed in other alkaline rocks from the Italian peninsula (Fig. 28a), and are rather typical of OIB-type rocks such as those of Mt. Etna. Enrichment in volatile elements, especially sulfur and chlorine, are very high, up to percent values. Sr isotope ratios range form 0.7055 to 0.7070, and 143Nd/144Nd ~ 0.5126-0.5128. Lead isotopic ratios (206Pb/204Pb = 19.13-19.48; 207Pb/204Pb = 15.68-15.72; 208Pb/204Pb = 39.16-39.55) show slightly more radiogenic compositions than other volcanic rocks from the Italian peninsula (Fig. 29; De Astis et al., 2006).
Figure 28. Spider-diagrams for Vulture and Campania volcanoes
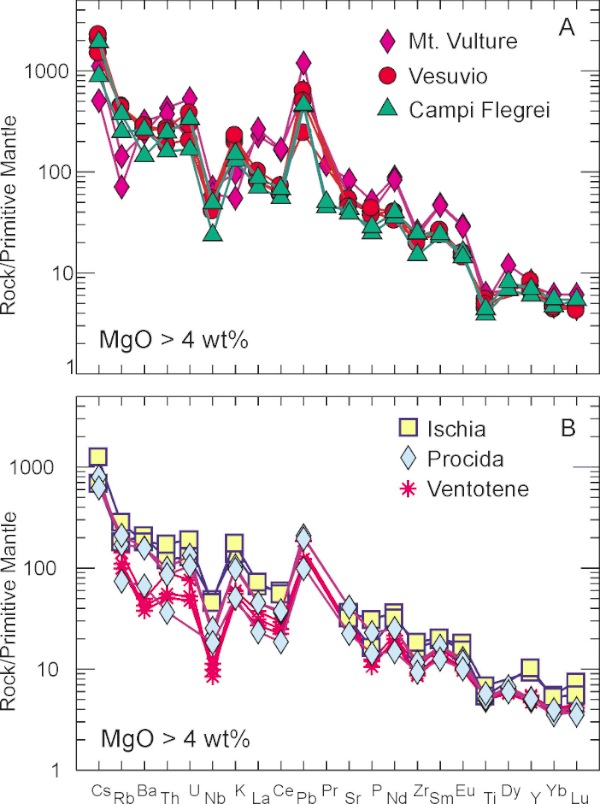
Incompatible element patterns normalized to primordial mantle composition for mafic rocks (MgO > 4%) from Mount Vulture and the Campania Province.
Campania Province and Eastern Pontine Islands
The Campania Province includes the active potassic and ultrapotassic volcanoes of Somma-Vesuvio, Campi Flegrei, Procida-Vivara, and Ischia. Ages range from about 0.2 Ma to present. The Pontine Islands (Ponza, Palmarola, Zannone, Ventotene and Santo Stefano) have variable ages and composition and only the eastern islands (Ventotene and Santo Stefano; age 0.8 to 0.13 Ma) and the younger rocks from Ponza (about 1 Ma) have compositional affinities with Campanian magmatism.
Volcanic rocks from Campania and Eastern Pontine islands range from mafic to felsic and exhibit variable degree of silica undersaturation and enrichment in potassium. Most rocks are moderately enriched in potassium, but reach ultrapotassic compositions at Vesuvio. Blocks of mafic rocks with K2O contents close to calcalkaline compositions have been found at Ventotene and Procida-Vivara (De Astis et al., 2006). As recalled earlier, 2 Ma old calcalkaline basalts to andesites have been found by borehole drilling beneath the Campanian Plain (Parete-2 drilling).
Somma-Vesuvio is a stratovolcano with a polygenic summit caldera, formed by an older cone (Somma) and by an intra-caldera cone (Vesuvio). Exposed activity ranges in age between 30 ka and present, although 0.4 Ma old ultrapotassic rocks have been found by drilling in the Vesuvius area (Brocchini et al., 2001). Vesuvio was built up inside the caldera depression of Monte Somma, after the 79 AD eruption that destroyed Pompeii and Herculaneum (Santacroce, 1987; Rolandi et al., 1998; Santacroce et al., 2003). Three main rock series showing distinct ages and variation trends for alkalies and some trace elements have been distinguished at Somma-Vesuvio (Fig. 30; Joron et al., 1987). The older rocks (> 8 ka) make up a slightly silica undersaturated potassic suite ranging from trachybasalt to trachyte. A second series (about 8 ka to 79 AD) is formed by moderately silica undersaturated phonotephrites, tephriphonolites and phonolites. The younger rocks (from 79 AD to present and forming the Vesuvio cone) are the most undersaturated in silica and range from leucite tephrite to leucitite, phonotephrite, tephriphonolite and phonolite. All rocks have variably porphyritic textures, with plagioclase and clinopyroxene as main phenocrysts. Leucite is more abundant in the younger than in the older rocks.
Sr, Nd, Pb isotopic compositions are similar in the three series (87Sr/86Sr ~ 0.7063 to 0.7080; 143Nd/144Nd ~ 0.5124 to 0.5125; 206Pb/204Pb ~ 18.90 to 19.10; 207Pb/204Pb ~ 15.61 to 15.71; 208Pb/204Pb ~ 38.90 to 39.30; Hawkesworth & Vollmer, 1979; Civetta et al., 1991a; Fig. 29). 176Hf/177Hf isotopic ratios for a few samples are around 0.28278 (Gasperini et al., 2002). Helium isotope studies on clinopyroxene and olivine from historical lavas gave values of R/RA ~ 2.2 to 2.7 (Graham et al., 1993). Oxygen isotope compositions determined on Vesuvio whole rocks (Ayuso et al., 1998) range between δ18O ~ +7.0 to +10.0, and are positively correlated with CaO. REE patterns of Somma-Vesuvio rocks (not shown) are fractionated for both LREE and HREE, with small negative Eu anomalies, which become stronger in trachytes and phonolites. Patterns of incompatible elements normalised to primordial mantle compositions for mafic rocks (Fig. 28a) show negative anomalies of HFSE and positive spikes of LILE and Pb.
Figure 30. TAS and trace element variations of Somma-Vesuvio
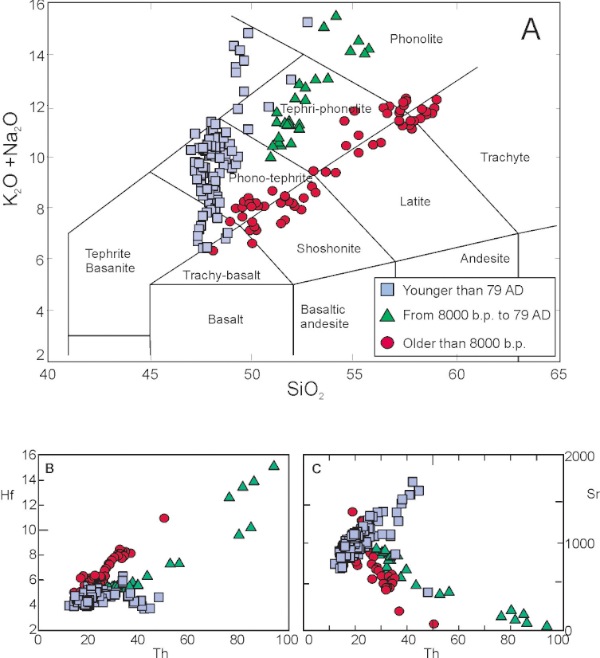
A – TAS diagram for Somma-Vesuvio volcanics. B, C – Th vs. Hf and Sr diagrams for Somma-Vesuvio volcanics.
Major and trace element variation at Somma-Vesuvio rocks suggest that fractional crystallisation was a leading evolution mechanism. Different trends of major and trace elements suggest that different types of phases were separated during fractionation, with a variable role being played by plagioclase. This may be related to polybaric fractional crystallisation and/or to a variable role of carbonate assimilation by potassic magmas, a process that destabilises plagioclase (Peccerillo, 2005b; Iacono-Marziano et al., 2008). Primitive rocks from Somma-Vesuvio have many geochemical and isotopic signatures that are similar to KS rocks from Stromboli, a feature that suggests a similar source for the two volcanoes (Peccerillo, 2001a).
The Campi Flegrei is a volcanic complex formed by two calderas and by several monogenetic cones and craters. The latest activity took place in 1538 AD when the Monte Nuovo phonolitic cone was formed. The Campi Flegrei rocks consist of dominant pyroclastic deposits and minor lavas. Compositions range from trachybasalt to trachyte and phonolite (Fig. 31) and are moderately undersaturated to oversaturated in silica. Some phonolites are peralkaline. Intermediate and felsic rocks largely prevail over mafic compositions.
Figure 31. TAS diagram, Campania volcanoes
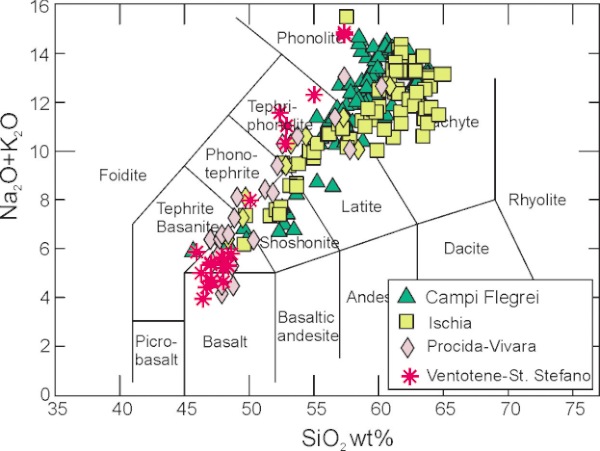
Total Alkalies vs. Silica (TAS) diagram for Campi Flegrei, Ischia, Procida-Vivara and Ventotene-Santo Stefano volcanoes.
Incompatible element patterns of mafic rocks normalised to primordial mantle composition (Fig. 28) show moderate negative anomalies of HFSE and Pb positive spikes. Sr isotopic ratios are similar to Somma-Vesuvio, although some larger range of compositions is observed (87Sr/86Sr ~ 0.7065 to 0.7086; 143Nd/144Nd ~ 0.5124 to 0.5128; 206Pb/ 204Pb ~ 18.90 to 19.25; 207Pb/204Pb ~ 15.65 to 15.77; 208Pb/204Pb ~ 38.95 to 39.38). Compositional variations at Campi Flegrei have been suggested to be related to fractional crystallisation plus come crustal assimilation and mixing, starting from a trachybasaltic parental magma (Pappalardo et al., 2002). Selective enrichment by fluids has been also invoked to explain high abundances of some volatile elements (Cl, F, and alkalies; Villemant, 1988).
Ischia consists prevalently of moderately potassic pyroclastic rocks and minor lavas, slightly less enriched in alkalies than Campi Flegrei. Ages range from more than 150 ka to 1302 AD. Rocks are intermediate to felsic, ranging from shoshonite to trachyte (Fig. 31). Trace element abundances are similar to the equivalent rocks of Campi Flegrei, whereas radiogenic isotope signatures are slightly more primitive (87Sr/86Sr ~ 0.7061 to 0.7076, 143Nd/144Nd ~ 0.51246 to 0.51261, 206Pb/204Pb ~ 19.01-19.21, 207Pb/204Pb ~ 15.66-15.71, 208Pb/204Pb ~ 39.06-39.34; Cortini & Hermes, 1981; Hawkesworth & Vollmer, 1979; Civetta et al., 1991b).
The islands of Procida and Vivara, sited between Campi Flegrei and Ischia, are formed by scoriae, lithic clasts, pumices, and hydrovolcanic ashes erupted between 55 ka and 17 ka (D'Antonio & Di Girolamo, 1994; D'Antonio et al., 1999 and references therein). Rock compositions range from basalt to trachyte with moderate abundances in alkalies (Fig. 31). Some lithic ejecta that show a calcalkaline composition. Overall, the Procida and Vivara rocks have trace element compositions that resemble closely the equivalent rocks from Ischia. However, mafic compositions are more depleted in alkalies and fall in the subalkaline field of Irvine and Baragar (1971). Sr and Nd isotopic ratios are respectively lower and higher than other Campanian volcanoes; Pb isotope ratios are variable (87Sr/86Sr = 0.7051 to 0.7065; 143Nd/144Nd = 0.5125 to 0.5126; 206Pb/204Pb ~ 18.68 to 19.30; 206Pb/204Pb ~ 38.68 to 39.99).
The Eastern Pontine islands of Ventotene and Santo Stefano consists of basalt and trachybasalt to phonolites (Fig. 31; Mètrich et al., 1988; D'Antonio & Di Girolamo 1994, D'Antonio et al. 1999). Mafic rocks are poorly enriched in potassium, but show a steep increase of alkalies with silica. Incompatible element patterns of mafic rocks (Fig. 28) are similar to other Campania potassic volcanics, but absolute abundances are lower. Radiogenic isotope compositions (87Sr/86Sr ~ 0.7070 to 0.7077; 143Nd/144Nd ~ 0.51228 to 0.51244; 206Pb/204Pb ~ 18.50 to 18.86; 207Pb/204Pb ~ 15.64 to 15.68; 208Pb/204Pb ~ 38.45 to 38.99; D'Antonio et al., 1996, 1999; Frezzotti et al., 2007; Conticelli et al., 2009b) resemble Campanian volcanoes but are also close to the moderately potassic rocks (KS) from Ernici and Roccamonfina.
The rocks from the Eastern Pontine islands, Campania Province, and Vulture show a variety of compositions in terms of petrological affinity, enrichments in potassium and incompatible elements. All the mafic rocks show a negative anomaly of HFSE, typical of arc-related magmas. However, the magmas of the Campanian volcanoes have small negative spikes of HFSE and their LILE/HFSE is lower than in other orogenic volcanic suites from central-southern Italy (Peccerillo, 2005a and references therein). Low LILE/HFSE ratios are typical of OIB, and, therefore, it has been suggested that the rocks from Campania and Vulture have intermediate compositions between OIB and arc rocks (e.g., Beccaluva et al., 1991, 2002; De Astis et al., 2006).
Sr-Nd-Pb isotope ratios of Vulture, Campania and Eastern Pontine volcanics have similar range of values, and plot midway between the Aeolian Arc and the central Italy potassic volcanoes, along a trend connecting OIB-type volcanoes of southern Italy (Etna, Iblei) and the upper crust (Fig. 41). This suggests an overall mixing between OIB-type mantle material and upper crust. Such a process is believed to result from mantle source contamination by upper crustal material (Peccerillo 2005a and references therein). Origin of OIB-type mantle is controversial. Inflow of asthenospheric upper mantle from the Adriatic plate after slab breakoff has been suggested by Peccerillo (2001b). Alternatively, the OIB-type signatures may represent deep mantle material that was emplaced at shallow level along structural discontinuities of the slab (Rosenbaum et al., 2008).
Ernici and Roccamonfina
The Monti Ernici volcanoes, consist of several pyroclastic and lava centres with an age of 0.7 to 0.1 Ma, sited in the Mid Latina Valley, about 70-80 km southeast of Rome. Roccamonfina is a stratovolcano with a central caldera and intra-caldera domes, formed between about 0.6 and 0.1 Ma. The peculiarity of this volcanic province is the occurrence of two contrasting series of rocks, showing distinct enrichments in potassium and incompatible elements, and radiogenic isotope signatures (e.g., Appleton, 1972; Civetta et al., 1981; Frezzotti et al., 2007; Rouchon et al., 2008; Conticelli et al., 2009b). A group of rocks showing ultrapotassic composition and classically indicated as the high-potassium series (HKS), ranges in composition from leucite tephrite to phonolite (Fig. 32). These are undersaturated in silica and display high enrichments in LILE and radiogenic Sr. Another group of rocks is less enriched in potassium, is saturated to poorly undersaturated in silica, and ranges in composition from calcalkaline basalt to moderately potassic trachybasalt, latite and trachyte. These latter are generally referred to as the potassic series or KS, and display a moderate enrichment in LILE and lower Sr isotopic signatures than HKS. A complete series of mafic to felsic KS and HKS rocks are found at Roccamonfina, whereas only mafic rocks occur at Monti Ernici.
Figure 32. TAS, Ernici and Roccamonfina
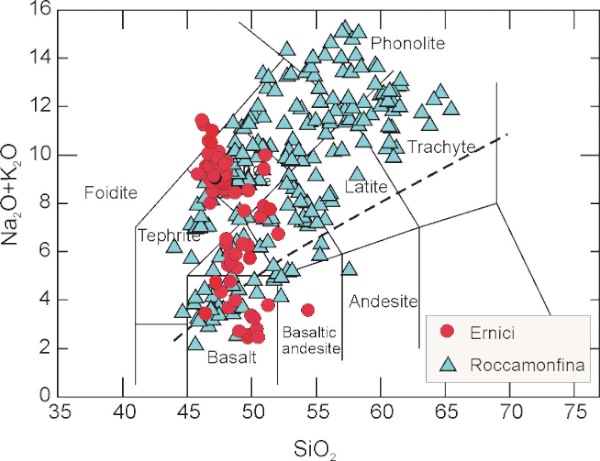
TAS diagram for Ernici and Roccamonfina volcanics. The ashed line is the divide between subalkaline and alkaline magmas.
Abundances of incompatible elements increase from KS to HKS. However, the shape of mantle normalised element patterns are similar (Fig. 33). Sr-Nd isotopic compositions show wide range of values, with an increase of Sr- and a decrease of Nd-isotope ratios from KS to HKS rocks. Pb isotopic ratios are poorly variable and are slightly less radiogenic in the HKS than in the KS rocks (Fig. 34). Overall, radiogenic isotope signatures of Ernici and Roccamonfina cover a large compositional range, linking the Campanian and Aeolian Arc volcanics to those from Latium and Intra Apennine provinces (Figs. 41, 46).
Figure 33. Spider-diagrams, Ernici and Roccamonfina
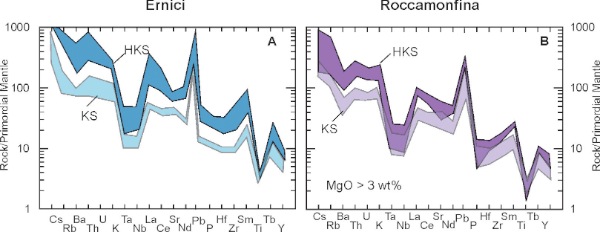
Incompatible element patterns normalized to primordial mantle composition for mafic rocks (MgO > 4%) from Ernici and Roccamonfina volcanoes.
There is a general agreement that the Ernici-Roccamonfina parental magmas were formed in an anomalous and heterogeneous mantle source that had undergone different amounts and types of metasomatic modifications. The variable compositions of the rocks erupted at the surface would reflect such a heterogeneity, but may also depend on variable degrees of partial melting (see Conticelli et al., this issue). Mantle metasomatism is likely related to subduction of the Adriatic plate beneath central Italy, with a possible role of material coming from the Ionian slab.
Roman Province
The Roman Province was early defined by Washington (1906) as the area of potassium-rich volcanism, extending from northern Latium (i.e., the Vulsini district) to the Campania area. A wealth of recent studies, however, have shown that the Campania volcanics have distinct petrological and geochemical characteristics as the Latium rocks, and that the Ernici-Roccamonfina magatism is somewhat transitional between the two (Peccerillo, 1999, 2002). Therefore, we here define the Roman Province s.s. (or Latium Province) as the region formed by the four large volcanic complexes of Vulsini, Vico, Sabatini and Colli Albani, sited between southern Tuscany to the city of Rome, along the border of the Tyrrhenian Sea (Fig. 26). These volcanoes erupted about 900 km3 of dominant pyroclastic products and minor lavas, from about 800 ka to 20 Ka. Volcanism was prevailingly explosive, with numerous plinian eruptions that generated large calderas and volcano-tectonic collapses. The pyroclastic flow deposits generated by these eruptions form thick piles of mildly welded rocks, which have been extensively used as building material form early historical times. The volcanism took place along faults of extensional basins whose formation is related to the opening of the Tyrrhenian Sea.
The Roman Province s.s. mostly consist of felsic, generally phonolitic and trachytic, pyroclastic deposits. These are derivative melts of mafic parents, after some 70-80% fractional crystallisation. This implies that the total amount of potassium-rich melts generated in the Roman Province is much higher than the already huge volumes exposed at the surface.
Rock compositions in the Roman Province are variable and include mildly undersaturated KS trachybasalts to trachytes, and strongly silica undersaturated HKS leucite tephrites, leucitites to phonolites. KS rocks are especially abundant during the latest phases of activity of Vulsini, are less common at Vico and rare at Sabatini, whereas they are absent at Colli Albani (Fig. 35).
Lava textures range from almost aphyric to strongly porphyritic. Pumices are glassy with a few phenocrysts. KS rocks generally contain phenocrysts of clinopyroxene, plagioclase and olivine in the mafic rocks, whereas sanidine and biotite appear in the felsic compositions. Leucite is often observed as phenocrysts and in the groundmass. The HKS rocks are also variably porphyritic with the same phenocrysts as the KS rocks, but with much higher leucite contents. Nepheline and haüyne are also observed. Clinopyroxene is often strongly zoned, a feature which is evident at the petrographic observation due to colour changes from green salite to colourless diopside.
Overall, the rock suites making up the KS and HKS of the Roman Province are formed by dominant fractional crystallisation processes, starting from different types of parental magmas showing distinct abundances of potassium and incompatible trace element, and radiogenic isotope compositions. Mixing and crustal assimilation also played important roles during magma evolution, and zoned clinopyroxenes commonly encountered in Roman rocks are considered as a main evidence of magma mixing. Assimilation of wall rocks is believed particularly abundant at Colli Albani. Here, extensive assimilation of limestones and dolostones strongly modified the evolution path of magmas, driving magma compositions from parent tephrite to foidite, instead of from tephrite to phonolite, as typically observed in other Roman centres (Gaeta et al., 2009; Iacono-Marziano et al., 2007; Peccerillo et al., 2010 and references therein).
Patterns of incompatible elements normalised to primordial mantle of mafic KS and HKS rocks have different element abundances but similar shapes, with enrichments in LILE, positive spikes of Pb, and marked depletion in HFSE (Fig. 36), whose abundances are much lower than in the Campanian volcanoes and are close to MORB (Peccerillo, 2005a). Radiogenic isotopic compositions are moderately variable, with 87Sr/86Sr ~ 0.7090 to 7110, 143Nd/144Nd ~ 0.5121, and 206Pb/204Pb ~ 18.80; 176Hf/177Hf ~ 0.28258.
Figure 36. Spider-diagrams, Roman Province
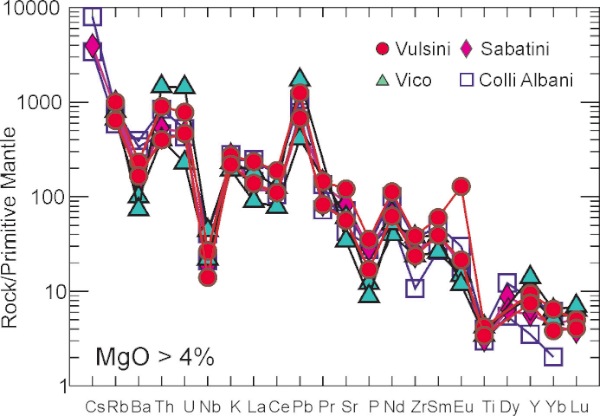
Incompatible element patterns normalized to primordial mantle composition for mafic rocks (MgO > 4 wt%) from the Roman (Latium) volcanic Province.
The bulk of geochemical and petrological data suggest that the parental magmas of Roman volcanoes were generated by various degrees of partial melting of a lherzolitic upper mantle, which contained phlogopite, generated by metasomatic processes. Geochemical and radiogenic isotope data suggest that metasomatic modifications were accomplished by addition to the upper mantle of sedimentary material with a marly composition (Peccerillo et al., 1988). This was related to subduction processes of the Adriatic slab beneath central Italy (e.g., Conticelli and Peccerillo, 1992; Conticelli et al., this issue).
Ultrapotassic rocks are rare at a global scale, but are very abundant in central Italy. The reason of this anomaly is still unclear, and although representing a main petrological and geodynamic problem, has not received much attention. Ultrapotassic magmatism is particularly abundant in the Latium region, in which the four huge polycentric volcanic complexes of Vulsini, Vico, Sabatini and Colli Albani developed almost contemporaneously over a time span of less than 1 Ma. This area has been affected by strong extensional tectonic regime, connected with the opening of the Tyrrhenian basin. Moreover, the upper mantle beneath the Latium area was also affected by two stages of metasomatic modification (Peccerillo, 1999), as it will be discussed later. One occurred during Alpine subduction of the European plate beneath the northern African margin; a second stage occurred during subduction of the Adriatic plate beneath the Italian peninsula. Both metasomatic stages were accomplished by introduction of continental-type material into the upper mantle. The extensive mantle modification resulting from the two-stage metasomatism, along with strong regional extension, may represent the reasons for the generation and eruption of large amounts of ultrapotassic magmas in this region.
Intra Apennine Province
Volcanoes of the Intra-Apennine Province (IAP) consist of several small centres made of ultrapotassic pyroclastic rocks and minor lavas, scattered along the axial zone of Apennines. The main occurrences include San Venanzo, Cupaello, and Polino, in Umbria. Lavas are found only at San Venanzo and Cupaello.
Lavas have olivine melilitite and kalsilite melilitite composition and show a typical ultrapotassic kamafugitic affinity, i.e. strong undersaturation in silica, lower SiO2, Na2O, Al2O3 and higher CaO and K2O/Na2O than Roman rocks. Carbonate-rich pyroclastic rocks are also present in this province. Kamafugitic lavas, especially those from San Venanzo, have incompatible element patterns and radiogenic isotope signatures that are similar to the mafic rocks of the Roman Province. This clearly suggests an origin in compositionally similar mantle sources that had undergone similar type of metasomatic modifications.
More controversial is the origin of carbonate rich pyroclastic rocks. Some authors suggest a carbonatitic nature (Stoppa & Woolley, 1997), whereas an interaction between kamafugitic magmas and sedimentary carbonates is suggested by other authors (Peccerillo, 1998; Barker, 2007). High oxygen isotopic compositions of silicate phases and of carbonates, with δ18O‰ ~ + 14 in olivine and pyroxene and δ18O‰ ~ + 20 to + 25 in calcite, support the latter hypothesis.
Tuscany Province
This is by far the most complex magmatic province in Italy. It includes both volcanic and intrusive rocks and extends from the Tuscan Archipelago to the Tolfa-Manziana area, a few km north of Rome (Fig. 37). Ages range from about 8 to 0.3 Ma. A small 14 Ma old ultrapotassic minette from Sisco, Corsica, is also included in the Tuscany Province.
Figure 37. Tuscany Magmatic Province
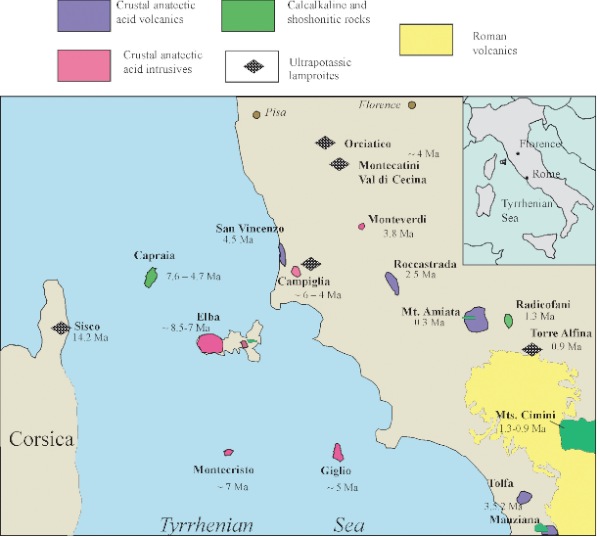
Distribution, composition and ages of magmatic rocks of the Tuscany Province. Note the overlap with the Roman (Latium) Province.
Rock compositions are variable from mafic to felsic (Fig. 38), and show calcalkaline, shoshonitic to ultrapotassic alkaline affinities. Ultrapotassic rocks in Tuscany have distinct petrological characteristics as the equivalent types in central Italy, and are classified as lamproites. These are oversaturated in silica and have high silica content (SiO2 ~ 55-60), high MgO (~ 7-10%) and Mg# (70-75), low CaO (3-5%) and Na2O (~ 1.5%). Many shoshonitic rocks, especially at Radicofani, result from mixing between lamproite and calcalkaline or Roman-type KS magmas (D’Orazio et al., 1994; Peccerillo, 1994; Peccerillo et al., 2008b).
Mafic rocks have high MgO and ferromagnesian element contents, and are of obvious mantle origin. Acid rocks are polygenic and may be generated by crustal anatexis, by fractional crystallisation of mafic-intermediate parents plus interaction with crustal rocks, and by mixing between different types of mantle-derived melts and crustal anatectic magmas (Poli, 1992; Poli et al., 2003).
Figure 38. Alkali-silica diagrams, Tuscany Province
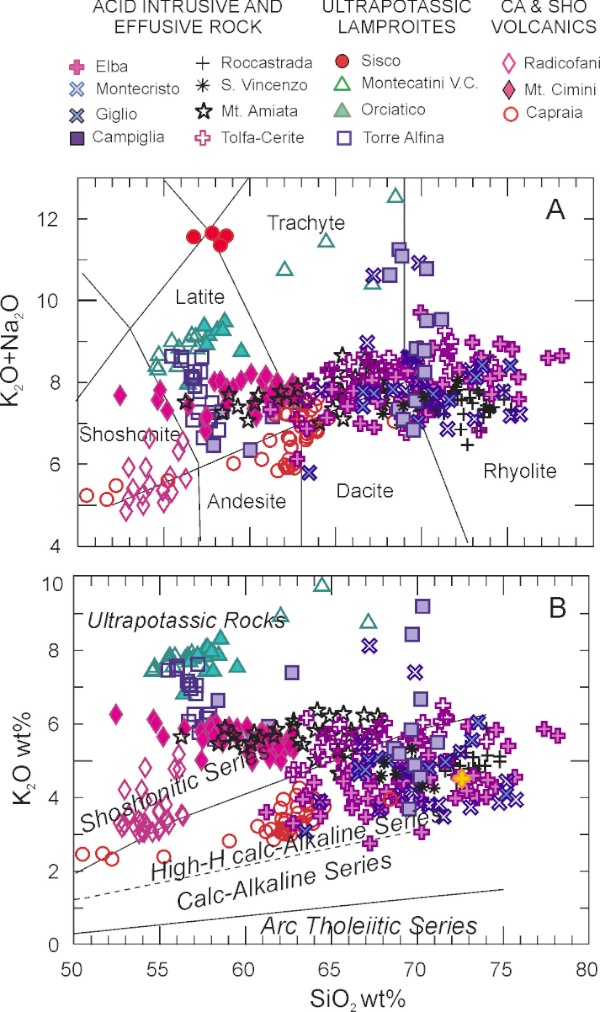
A - Total Alkalis vs. Silica, and B - K2O vs. SiO2 classification diagrams for volcanic rocks from the Tuscany Province.
Calcalkaline and HKCA to shoshonitic rocks in Tuscany mainly crop out at Capraia, but also occur as mafic enclaves in some granitoid intrusions and acidic lavas (e.g., Elba; Poli, 1992). Shoshonitic rocks occur at Radicofani and Cimini but are also found in minor amounts in other centres, such as the silicic volcano of Monte Amiata. Lamproitic rocks occur at Montecatini val di Cecina, Orciatico, Torre Alfina and Sisco (Corsica).
Incompatible element abundances of mafic rocks increase from CA to SHO and lamproitic magmas. Mantle normalized incompatible element patterns of all mafic rocks (Fig. 39) are fractionated and show evident negative anomalies of HFSE, Sr and Ba. These patterns are very similar to those of the Oligocene mafic rocks of the Western Alps, where a similar association of calcalkaline, shoshonitic and lamprotic magmas is observed (Peccerillo et al., 1988; Peccerillo & Martinotti, 2006). Overall, these patterns resemble those of some upper crustal rocks, such as shale and gneiss.
Figure 39. Spider-diagrams, Tuscany Province
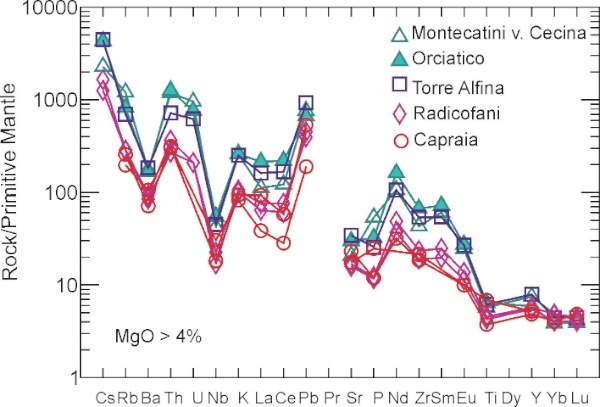
Incompatible element patterns normalized to primordial mantle composition for mafic rocks (MgO > 4) from the Tuscany Province.
Sr-Nd isotope ratios of Tuscany mafic rocks are variable. Capraia shoshonitic basalts and HKCA andesites show the least radiogenic Sr and the highest Nd isotopic signatures in the Tuscany Province (87Sr/86Sr ~ 0.708-0.710), whereas more radiogenic Sr compositions are found at Radicofani (87Sr/86Sr = 0.713-0.716), Cimini and Amiata (Poli et al., 1984; Conticelli et al., 2002) (Fig. 40a). Lamproitic rocks have the highest 87Sr/86Sr (~ 0.713-0.717) and the lowest 143Nd/144Nd (~ 0.521-0.522) among mafic rocks. Pb isotopic ratios are poorly variable (Fig. 40b).
Figure 40. Radiogenic isotope compositions, Tuscany Province
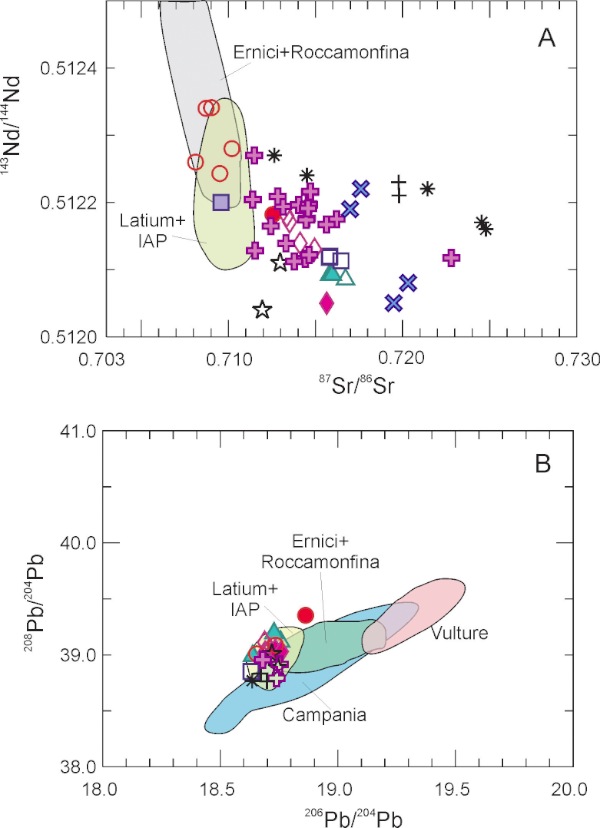
Sr-Nd-Pb isotopic compositions of volcanic rocks from the Tuscany Province. Symbols as in Fig. 38.
Silicic rocks in Tuscany consist of lavas (San Vincenzo, Roccastrada, Monte Amiata and, at some extent, at Monti Cimini), and several intrusions occurring in the Tuscan archipelago (Elba, Giglio and Montecristo islands), the northern Tyrrhenian Sea floor (Vercelli seamount) and on the mainland (Campiglia). Other intrusive bodies occur at shallow depths beneath the Amiata and Larderello area. Pyroclastic rocks are only present at Mt. Cimini where rhyodacitic ignimbrites crop out. Intrusive rocks have similar major, trace element and radiogenic isotope compositions as extruve rocks.
Some silicic rocks (e.g., Roccastrada peraluminous rhyolites; Mazzuoli, 1967) represent pure crustal anatectic magmas, and were egenerated by partial melting of metapelites (Giraud et al., 1986; Pinarelli et al., 1989). Their Sr-isotope ratios are high (87Sr/86Sr ~ 0.718-0.720 ca) and Nd isotopes are low (143Nd/144Nd ~ 0.51222).
Other acid rocks (e.g., rhyolitic lavas of San Vincenzo, Cimini, Amiata, Tolfa-Cerveteri) are hybrids between crustal anatectic melts and subcrustal mafic-intermediate magmas (Vollmer, 1976; Giraud et al., 1986; Pinarelli, 1991) Their 87Sr/86Sr and 143Nd/144Nd are variable and sometimes show disequilibrium between phenocrysts and groundmass (Ferrara et al., 1989; Feldstein et al., 1994).
Petrological and geochemical variability of magmas in the Tuscany Province suggests complex petrogeneitic processes, which are still debated. The large variety of mafic magmas calls for an origin in a heterogeneous mantle source. The low CaO, Na2O and Al2O3 and the high MgO, Ni and Cr of lamproites suggest an origin in an upper mantle that was depleted in mineral phases that contain CaO, Na2O and Al2O3 as major components (i.e., clinopyroxene). On the other hand, high potassium contents of lamproites points to the occurrence of phlogopite or other K-rich phases in the source. Therefore, a phlogopite harzburgite has been suggested as a source of lamproitic magmas in Tuscany (Peccerillo et al., 1988; Conticelli & Peccerillo, 1992). Phlogopite was generated by metasomatic processes, which were also responsible for enrichments in incompatible elements and radiogenic Sr of mantle source. Upper crustal material with a pelitic composition is the best candidate for mantle contaminant in Tuscany (Peccerillo et al., 1988). Calcalkaline and shoshonitic rocks have higher CaO and Na2O and Al2O3, and lower K2O and incompatible trace elements than lamproites. This suggests an origin in a mantle source that contained clinopyroxene but was less intensively metasomatised than the lamproite source. However, the similar shape of incompatible element patterns suggests that the nature of metasomatic contaminant was the same as for lamproites.
The geochemical evidence suggesting that the Tuscany upper mantle was contaminated by crustal material with a pelitic composition, led to infer a subduction-related origin for this magmatism (Peccerillo et al., 1988; Conticelli & Peccerillo, 1992; Serri et al., 1993). However, the age of the subduction event(s) is discussed. It has been noted that similar mafic rocks as in Tuscany, also occur in the Western Alps, where magmatism is Oligocene in age and has been associated to mantle contamination during subduction of the European plate beneath the western Alpine margin. Since Tuscany mafic rocks resemble closely those in the Western Alps, it has been suggested that also the Tuscany upper mantle was contaminated during Alpine subduction (Peccerillo & Martinotti, 2006 and references therein). This is supported by the hypothesis that Tuscany region represents a portion of the Alpine belt shifted eastward during opening of the Tyrrhenian basin (Doglioni et al., 1998).
Tyrrhenian Sea and Italian Peninsula Orogenic Magmatism: A Summary of Compositional Features and Geodynamic Implications
The very wide petrological and geochemical variations of orogenic magmatism in and around the Tyrrhenian Sea, highlight complex origin and evolution processes for magmas and their sources. Parental magmas originated within the upper mantle and underwent various evolution processes, including fractional crystallisation, assimilation, and mixing, giving different types of derivative magmas. However, compositions of mafic rocks are believed to reflect rather closely those of mantle-equilibrated parents, and, therefore, are able to furnish important constraint on source composition and processes. The main compositional features can be summarised as follows:
1 - Major, trace element ratios and radiogenic isotope signatures of mafic rocks are variable and reflect heterogeneous mantle sources with diverse modal mineralogy, trace element and isotopic compositions.
2 - Potassic alkaline composition are dominant in the Italian peninsula, and also occur in the central-eastern Aeolian Arc. This requires an origin of primary melts in phlogopite-bearing mantle sources. The presence of this mineral in the upper mantle demands metasomatic enrichment processes, a hypothesis strongly supported by high abundances of LIL element and the anomalous radiogenic isotope compositions of magmas. High ratios of LILE/HFSE, which are typical of island arc magmas, require that subduction processes are responsible for mantle metasomatism. However, this conclusion is not unanimously accepted, and alternative hypotheses invoking mantle plumes or deep mantle fluids have been suggested (e.g., Locardi, 1988; Bell et al., 2004). Such an issue is discussed by Peccerillo & Lustrino (2005) and will not be reiterated here.
3 - Sr-Nd-Pb-He isotopic variations of Plio-Quaternary Italian orogenic volcanics define continuous trends between the OIB-type rocks of Etna and eastern Sicily, and the upper crust (Fig. 41). This points to an interaction between mantle and crustal end-members in the genesis of Italian orogenic magmatism. Such an interaction occurred mainly in the upper mantle by addition of crustal material by subduction processes.
Figure 41. Sr-Pb isotopic variation in Italy
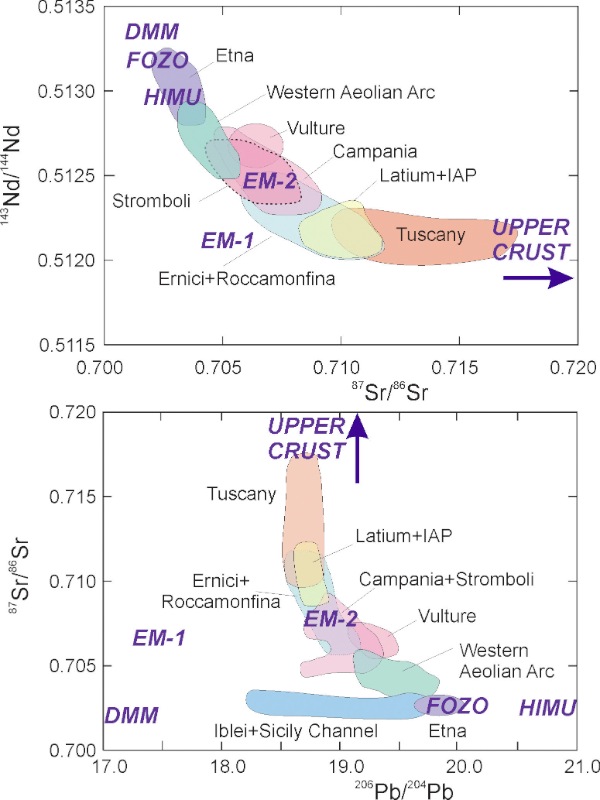
87Sr/86Sr vs. 143Nd/144Nd and 206Pb/204Pb for mafic (MgO > 4 wt%) orogenic rocks in central-southern Italy. Compositions of anorogenic of Etna and Sicily Channel are also shown. DMM (Depleted MORB-type Mantle), HIMU, FOZO, EM-1 and EM-2 are worldwide mantle end-member compositions.
4 - Variations of key trace elements ratios and radiogenic isotopes for orogenic mafic rocks in Italy (Figs. 41, 42) highlight different compositions for various volcanic provinces (Peccerillo, 1999). These have been explained as an effect of different compositions of crustal contaminants (i.e. MORB, MORB+sediments, marls, pelites) in different regions. However, some Italian volcanic rocks show higher incompatible trace element ratios (especially LILE/HFSE; e.g., Th/Ta) than any crustal rock. However, fluids released from subduction zones are able to transport more readily LILE (Rb, Th, U, K, etc.) than HFSE (Ta, Nb, Zr, etc.), and, therefore, have higher LILE/HFSE ratios with respect to the source crustal rocks. Such an increase is indicated by arrows in Fig. 42. Length of arrows signify the degree of LILE over HFSE enrichment, which appears stronger in the south. This supports a stronger role of aqueous fluids in the Aeolian Arc than in the magmatic province of central Italy.
Figure 42. Trace element ratios, central-southern Italy
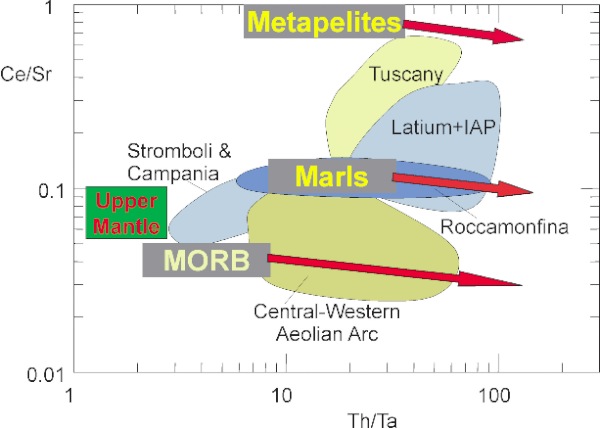
Variations of LILE/LILE (Ce/Sr) and LILE/HFSE (Th/Ta) ratios in mafic (MgO > 3 wt%) orogenic rocks in central-southern Italy. Note strong regionality, which is explained by source contamination by different types of crustal rocks, i.e. MORB, marls, metapelites. Fluids transfer from slab to mantle wedge (arrows) produces small variation of LILE/LILE and a significant increase of LILE/HFSE. Length of arrows indicates the degree of LILE/HFSE enrichments operated by fluids. These are stronger in the Aeolian Arc, suggesting a stronger role of fluids in the mantle metasomatism of this region.
5 - Calcalkaline magmas are dominant in the Aeolian Arc and Tyrrhenian seamounts. This points to large degrees of partial melting in the presence of aqueous fluids, in agreement with evidence arising from LILE/HFSE ratios. Sr-irotope compositins of these magmas are poorly radiogenic, suggesting source contamination by a poorly radiogenic crust, such as MORB. Variations of isotopic compositions along the Aeolian Arc, require an increase in the role of upper crustal contaminants, most likely sediments from the Ionian slab, going from the western to the eastern islands. Similar trace element ratios and radiogenic isotopic signatures for Stromboli rocks and the Campania Province suggest a similar mantle contamination history, and have been interpreted as evidence that the Campania Province represents the northern end of the Aeolian Arc rather than the southern extension of the Roman Province (Peccerillo, 2001a).
6 -The occurrence of dominant potassic alkaline rocks in central Italy reveal stronge degrees of mantle contamination by upper crust. Marly sediments have been suggested as contaminants of mantle wedge in the Roman, Intra Apennine, and Ernici-Roccamonfina provinces. In Tuscany, contaminants with pelitic composition are required by geochemical and isotopic data.
7 - Many compositional characteristics of mafic magmas were likely inherited from pre-metasomatic mantle sources. However, pristine nature of the upper mantle is difficult to constrain because of the intensive modification suffered by metasomatism. The single trend between Etna and the upper crust defined in the Sr-Nd-Pb isotope space by the bulk of orogenic magmas in Italy (Fig. 41) suggests a single type of composition for mantle end-member. This has a composition similar to Etna, which has isotopic signatures close to the so-called FOZO (Focus Zone) mantle composition. The physical significance of FOZO is a main problem of petrology and geochemistry at a global scale (Stracke et al., 2005), and a deep mantle origin has been suggested for Italy (Gasperini et al., 2002; Bell et al., 2004). However, low contents of HFSE in several volcanoes, such as ultrapotassic rocks of the Roman Province, require mantle sources that are depleted in these elements, pointing to a MORB-type pre-metasomatic mantle, in most cases.
The complex history of mantle contamination suggested by petrological and geochemical data is related to several stages of metasomatic events occurred during the late Tertiary to present geodynamic evolution of the Mediterranean area. Such an issue is the focus of other papers in this volume.
Basically, two main subduction processes have been responsible for the origin of Alps and Apennine chain and associate magmatism (Fig. 43). Late Tertiary to Oligocene "Alpine" subduction of the European plate generated a belt of metasomatic mantle along the northern border of the African plate (Fig. 43a). Melting of this metasomatic mantle took place contemporaneously or later than subduction, and at different times in the various zones, depending on local modifications of tectonic and thermal regimes (Peccerillo & Martinotti, 2006; Beltrando et al., 2010 and references therein). Significant contamination by the upper continenental crust affected the mantle wedge beneath the Western Alps and the region that will be later the site of the Tuscany and Roman provinces.
Successively, convergence between Africa and Europe took place by subduction of the Adriatic-Ionian plate beneath the southern European margin (Apennine subduction). This process also generated mantle contamination and magmatism, which initially affected Sardinia and Provence (Fig. 43b) and successively shifted eastward, during opening of the Balearic and Tyrrhenian basins and the rotation of the Italian peninsula (Fig. 43c,d) (e.g., Doglioni et al., 1999). This second stage of orogenic magmatism also had a calcalkaline to ultrapotassic composition as along the Alps, but ultrapotassic magmatism dominating in central Italy (Roman-type magmas; see Peccerillo, 1992) has a distinct composition as the ultrapotassic lamproitic rocks from Western Alps. Roman-Tuscany area, which is a portion of the former Alpine collision zone that migrated eastward (Doglioni et al., 1998), was affected by both Cretaceos-Oligocene "Alpine" and Oligocene to present "Apennine" subduction. Therefore, and its lithospheric upper mantle was subjected to two stages of metasomatism. These superimposed to each other generating a pervasively metasomatised mantle that originated abundant potassic and ultrapotassic magmatism. It is worth recalling that calcalkaline to lamproitic magmatism in Tuscany is different from the equivalent rocks occurring in other provinces of central Italy, and resembles closely to the Oligocene magmatism of Western Alps. The occurrence in the Tuscany-Roman area of two types of ultrapotassic magmatism is considered as an evidence of a two-stage metasomatic modification suffered by the upper mantle in this area (Peccerillo and Martinotti, 2006).
Figure 43. Geodynamic evolution of the Tyrrhenian Sea area
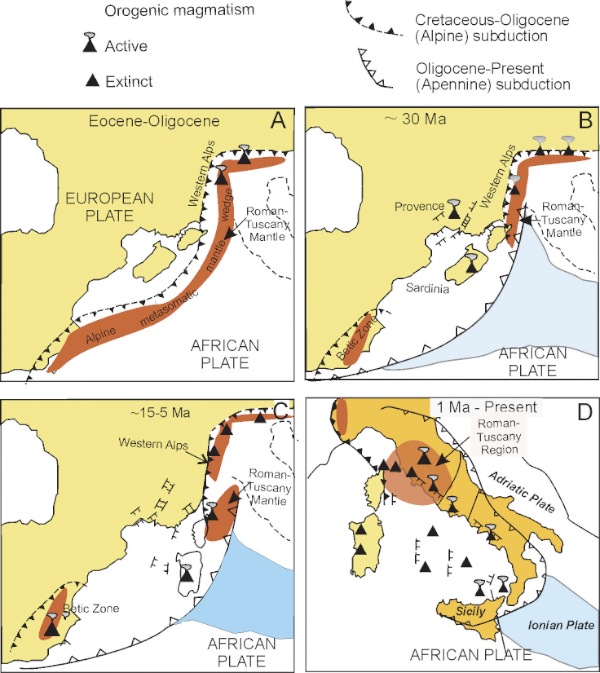
Schematic evolution of the Tyrrhenian Sea area from Eocene-Oligocene to present. For explanation, see text.