Alps
Introduction
Orogenic magmatism along the Alps took place during the Eocene and Oligocene (~ 43-24 Ma). The climax was reached around ~ 32-30 Ma, shortly after the final stages of the continental collision between the European and Adriatic continental margins, which was accomplished at ~ 35-32 Ma (e.g., Berger & Bousquet, 2008; Stampfli & Hochard, 2009; Handy et al., 2010). Although subduction processes appear to have characterized Alpine geodynamics at least since the Upper Cretaceous (~ 90-80 Ma; Berger & Bousquet, 2008; Stampfli & Hochard, 2009; Handy et al., 2010 for reviews), no convincing evidence exists for subduction-related magmatism prior to the middle Eocene. By contrast, collisional to post-collisional magmatism of late Eocene-Oligocene age is widespread in the Alps.
Figure 1. Tectonic map of the Alps
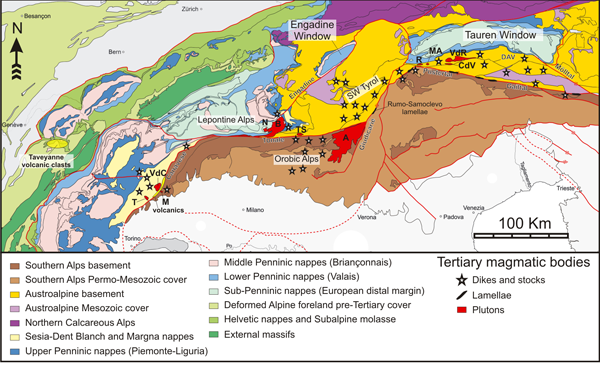
Tectonic map of the Alps (modified after Schmid et al., 2004) with location of the main plutonic bodies (in red) and areas (stars) where small size intrusive bodies occur. T: Traversella; VdC: Valle del Cervo; M: Miagliano; N: Novate; B: Bregaglia (Bergell); TS: Triangia-Sondrio; A: Adamello; R: Rensen; MA: Monte Alto; VdR: Vedrette di Ries; CdV: Cima di Vila; DAV: Deferegger-Anterselva-Valles tectonic line.
The products of the Eocene-Oligocene magmatism are mainly exposed in the vicinity of the Periadriatic Fault System (PFS; Fig. 1), an orogen-parallel, crustal-scale transpressive mylonitic belt that extends from the Valle d'Aosta region in the West to the Pannonian Basin in the East (e.g., Schmid et al. 1989; Rosenberg, 2004). Bodies of variable size emplaced along the PFS from the Western to Eastern Alps mainly consist of plutonic and innumerable basic to acidic dikes. Minor volcanics and volcanoclastic sequences are restricted to the westernmost branch of the PFS (Canavese Line). Variable amounts of volcanic material also occur in clastic formations of the External Alps (Laubscher, 1983; Dal Piaz & Venturelli, 1983; Waibel, 1993).
Plutonic bodies are mainly composed of diorite-tonalite-granodiorite rock associations with calcalkaline (CA) to high-K calcalkaline (HKCA) compositions (according to the chemical nomenclature proposed by Peccerillo & Taylor, 1976). Dikes are largely andesites belonging to the calcalkaline and shoshonitic (SHO) series. However, some important petrographic and geochemical variations occur along the Alpine arc. In particular, the Western Alps are the site of ultrapotassic igneous activity that is absent in the Central and Eastern Alps.
Different models have been proposed for the genesis of the Alpine magmatism, including subduction zone melting, lithospheric extension-related melting, thermal boundary layer detachment and melting of the Alpine crustal root, and slab breakoff (von Blanckenburg et al. 1998 for a review; Beltrando et al. 2010).
Western Alps
Tertiary magmatism in the Western Alps is mostly confined to the Sesia-Lanzo Zone of the Austroalpine domain, with only few dikes and stocks intruded into the Penninic and Southalpine units of the Internal Alps. Volcanic material occurs also in flysch deposits in the External Alps (Fig. 1).
Traversella pluton
The ~ 30 Ma old (biotite K/Ar age; Krummenacher & Evernden, 1960) Traversella pluton intrudes the “Micascisti Eclogitici” unit of the Sesia-Lanzo Zone. It is composed mostly of diorite to quartz-diorite with minor monzonite, formed by various proportions of biotite, augite, amphibole, plagioclase, quartz and K-feldspar. Mafic cumulates of biotite, augite, ilmenite and magnetite also occur, along with abundant inclusions of both igneous and metasedimentary origin. Various granitic and aplitic veins cross cut the main dioritic body (van Marcke de Lummen & Vander Auwera, 1990).
The Travesella plutonic rocks display a HKCA petrologic affinity with some mafic cumulates exhibiting a shoshonitic composition (Fig. 2a). TiO2, FeOtot, MgO, CaO decrease regularly with increasing SiO2 whereas Al2O3, Na2O and P2O5, and some trace elements (e.g., Sr, Ba, LREE) show bell-shaped trends, with an increase in the mafic range and a decrease in silicic compositions. K2O and other incompatible trace elements (e.g., Rb, Th, U, Zr) are positively correlated with SiO2, but aplites generally show the lowest concentrations for most of the trace elements.
Figure 2. Alkali-silica classification diagrams, Western Alps
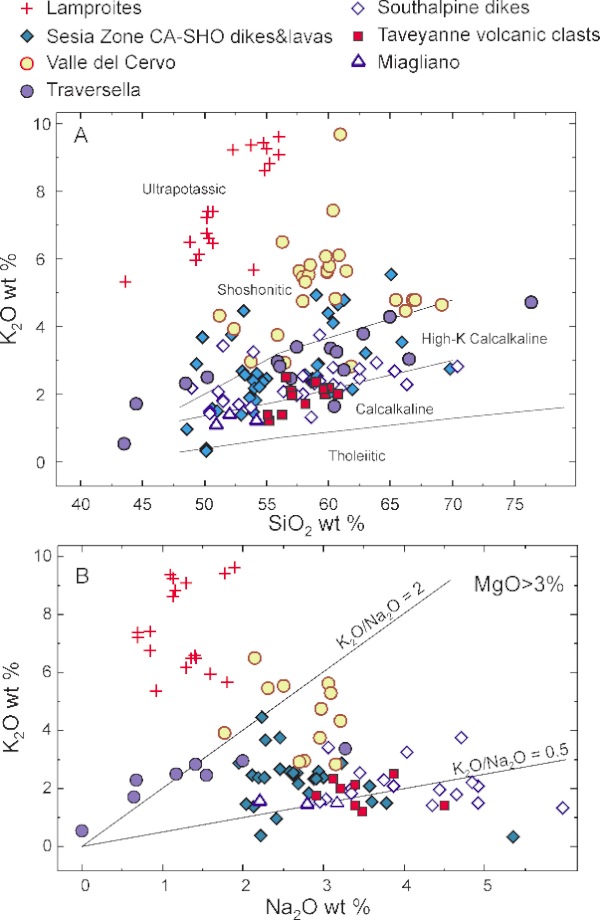
(A) - K2O vs. SiO2 classification diagram for the Western Alps Tertiary igneous rocks; (B) - K2O vs. Na2O diagram for mafic rocks (MgO > 3 wt%) from Western Alps.
Mafic rocks (MgO > 3%) exhibit always K2O/Na2O higher than unity, and have Na2O contents generally lower than 2% (Fig. 2b). They display fractionated incompatible element patterns (Fig. 3) with high enrichments in Rb, Ba, Th, U, LREE, and positive spikes of Th and U anomalies and depletion in Ti and Zr. REE patterns for the mafic samples show variable fractionation (LaNYbN ~ 6.3-13.8; chondrite normalizing values from Sun & McDonough, 1989) with small Eu anomalies.
Figure 3. Spider-diagrams of mafic rocks, Western Alps
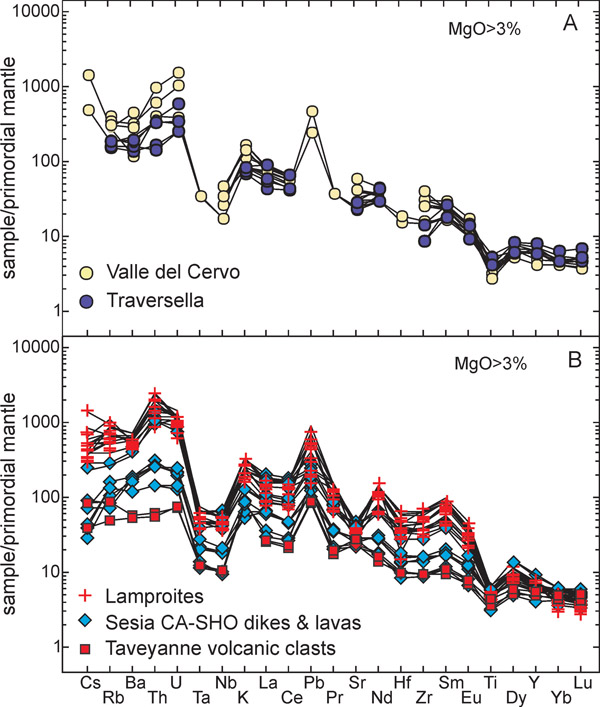
Incompatible element patterns normalized to primordial mantle composition for mafic igneous rocks (MgO > 3%) from the Western Alps.
Dioritic to monzonitic rocks have poorly variable initial 87Sr/86Sr (~ 0.7099-0.7107) and 143Nd/144Nd ( ~ 0.5122) (Fig. 4a). Their lead isotope ratios are rather homogeneous (206Pb/204Pb ~ 18.67-18.69, 207Pb/204Pb ~ 15.64-15.67 and 208Pb/204Pb ~ 38.73-38.86) and coincide with upper crustal values (Fig. 4b,c). Oxygen isotope data have been determined for whole-rocks and some separated minerals. There is an increase of whole-rock δ18O with SiO2 from +5.9‰ of cumulates to +10.5‰ of granitic veins (Fig. 5; van Marcke de Lummen & Vander Auwera, 1990).
On the basis of major, trace element and O and Sr isotope modelling, van Marcke de Lummen & Vander Auwera (1990) proposed that the most evolved rocks of the Traversella pluton derived form the less evolved ones by fractional crystallization and assimilation (AFC) processes. The geochemical characteristics of some granitic veins are compatible with an origin by AFC, whereas others are more likely derived by local partial melting of the country rocks. Low oxygen isotopic ratios of mafic cumulate, imply a crystallisation from uncontaminated mantle-derived melts, which were likely parents of the Traversella pluton
Figure 5. Oxygen isotope variation
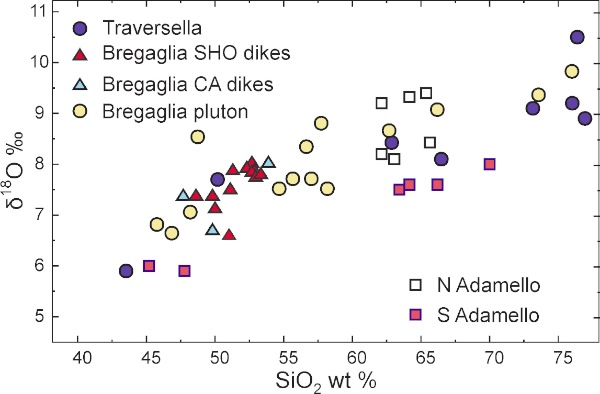
Whole-rock δ18O vs. SiO2 diagram for the Traversella, Bregaglia (Bergell) and the Adamello intrusions.
Valle del Cervo pluton
The Valle del Cervo (Biella) pluton occurs north of Traversella intrusion. It emplaced into the “Micascisti Eclogitici” unit as a broadly concentric structure, with a granitoid complex at the core, surrounded by syenitic and monzonitic rocks. The granitoid complex consists of porphyritic monzogranite to quartz-poor granodiorites. The syenite complex consists of a clinopyroxene-bearing, biotite-hornblende syenite, locally including rounded enclaves of quartz-monzonites. The monzonite complex mainly consists of two-pyroxene-biotite-hornblende quartz-monzonite to mela-syenite, with porphyritic monzodiorite varieties at the contact with the country rocks. Mafic enclaves as well as aplitic and pegmatitic dikes occur in all the three complexes (Fiorentini Potenza, 1959; Bigioggero et al., 1994; Rossetti et al., 2007). Within the metamorphosed Sesia-Lanzo country rocks, several small satellite igneous bodies have been recognized. These include hornblende-biotite monzogabbro, gabbronorite to quartz-diorite, cordierite-bearing quartz-monzodiorite, syenite and plagioclase-quartz-tremolite-tourmaline orbicular rocks. Different types of hydrothermal mineralizations occur both within the Biella stock and at its roof zone (Rossetti et al., 2007).
U-Pb zircon dating of the granodiorite indicates an age for the inner granitoid complex of ~ 31 Ma (Romer et al., 1996). Bigioggero et al. (1994) published Rb/Sr biotite ages of 30-31 Ma for the monzonitic complex and 29-30 Ma for the granitoid complex.
The rocks of the Valle del Cervo pluton define a shoshonitic association, but some syenitic-monzonitic samples and mafic enclaves display an ultrapotassic affinity (Fig 2a). K2O/Na2O ratios for the mafic lithotypes (MgO > 3%) are generally comprised between 1 and 2 with some monzonites displaying K2O/Na2O > 2 (Fig. 2b).
Major element versus SiO2 diagrams display negative correlations for all oxides, except for the alkalies. Incompatible trace element versus SiO2 diagrams show wide scattering. Monzonitic and syenitic samples of the main plutonic mass generally exhibit the highest enrichments in incompatible trace elements (e.g., Rb, Ba, Th, U, Nb, Sr, Zr, LREE). The lowest abundances of incompatible trace elements are generally found in the diorite and monzogabbro satellite bodies.
Diorites to monzonites with MgO > 3% display fractionated incompatible element patterns with enrichments for some of the most incompatible elements (e.g., Cs, Th, U up to more than 1000 times Primordial Mantle values; Sun & McDonough, 1989), positive Th, U and Pb anomalies and pronounced negative spikes of Nb, Ta and Ti (Fig. 3). Ba and Rb are slightly depleted compared to elements with similar incompatibility (e.g., Cs, Th, U). Syenites and granites display incompatible element enrichments and patterns (not shown) that are comparable as the more mafic samples, but also have a distinct Ba negative anomaly. Overall, the Valle del Cervo pluton is significantly more enriched in most of the incompatible trace elements with respect to the Traversella pluton, in accordance also with the more potassic affinity of the former.
The initial 87Sr/86Sr isotopic ratios displayed by the Valle del Cervo pluton are similar in the granitoid and syenite complexes (~ 0.7089-0.7097), but are systematically higher in the monzonite complex (~ 0.7093-0.7113). Initial Nd isotope ratios are less variable and range between 0.51225 and 0.51228 with the lowest values observed in monzonitic samples (Fig. 4a). Pb isotopic ratios are rather homogenous (206Pb/204Pb ratios = 18.72-18.79; 207Pb/204Pb = 15.61-15.70; 208Pb/204Pb = 38.6-38.87); samples from the granitoid complex show the least radiogenic compositions (Fig. 4b,c).
Mainly on the basis of Sr isotope data, Bigioggero et al. (1994) concluded that two different types of magmas with distinct petrochemical affinity were present at Valle del Cervo pluton, one forming the monzonite complex and the other forming the syenite and granitoid complexes. The two magma suites evolved from isotopically different parental melts by combined fractional crystallisation and assimilation processes. The parental magmas of both suites were generated in a heterogeneous upper mantle source, which had been variably metasomatised by subducted upper crustal material.
Miagliano stock
The Miagliano stock is intruded into the gabbroic rocks of the Southalpine Ivrea-Verbano Zone near the Valle del Cervo pluton, south-east of the Canavese Line. It is composed of a monzonitic core with some monzodiorite, surrounded by an outer zone of diorite (Carraro & Ferrara, 1968; Dal Piaz et al., 1979). Carraro & Ferrara (1968) reported Rb/Sr and K/Ar biotite ages ranging between 29-33 Ma.
The few available data show that the Miagliano diorite-monzonite intrusion is calcalkaline in composition (Fig. 2a), with K2O/Na2O ratios around 0.5 (Fig. 2b). P2O5 and incompatible trace element (Rb, Sr, Zr, Nb) concentrations are lower than in similar rocks from the Taversella and Valle del Cervo plutons. Initial 87Sr/86Sr ratios (~ 0.7068-0.7075) are significantly lower than those measured for the Traversella and Valle del Cervo rocks (Fig. 4a).
Western Alps dikes and volcanic rocks
Dikes
Within the Sesia-Lanzo Zone, a large number of dikes occur around the Traversella and Valle del Cervo plutons and, more scarcely, northwards as far as the Ossola valley (Dal Piaz et al., 1979; Beccaluva et al., 1983). Dikes mainly include calc-alkaline to shoshonitic rocks ranging in composition from basalts and basaltic andesites to andesites and latites, with some ultrapotassic mafic dikes classified as lamproites. A few lamproitic dikes also cross cut the Combin unit of the Piemonte Ophiolite Nappe (Dal Piaz et al., 1979; Diamond & Wiedenbeck, 1986), whereas granodiorite porphyritic dikes are reported in the north-eastern margin of the Sesia-Lanzo Zone, near the western margin of the Lepontine Gneiss Dome (Romer et al., 1996). Basalts, basaltic andesites and andesites are variably porphyritic and contains various proportions of hornblende, clinopyroxene and plagioclase phenocrysts and minor biotite; in contrast, shoshonitic basalts have a phenocryst mineralogy dominated by biotite and clinopyroxene with minor hornblende phenocrysts set in a groundmass of K-feldspar and plagioclase. The latites have phenocryst assemblages of biotite, hornblende, plagioclase and K-feldspar. The lamproitic dikes contain abundant phlogopite, clinopyroxene and K-feldspar set in a groundmass mainly composed of K-feldspar and minor clinopyroxene. Some lamproites contain abundant riebeckite–arfvedsonite amphibole (Dal Piaz et al., 1979; Venturelli et al., 1984). Ages obtained for lamproitic dikes range between 30.3 and 32.7 Ma (K/Ar: Dal Piaz et al., 1973; Diamond & Wiedenbeck, 1986; Rb/Sr: Pettke & Diamond, 1997); granodiorite porphyritic dikes from the north-eastern margin of the Sesia-Lanzo Zone gave U/Pb titanite ages of 31.7 and 32.4 Ma (Romer et al., 1996). Recently, Babist et al. (2006) reported a poorly constrained plagioclase-amphibole Rb/Sr isochron age of ~ 44 Ma for an andesitic dike near the Traversella pluton, which would implicate an Eocene onset for the magmatic activity in the internal Western Alps.
A series of porphyritic dikes of uncertain age are found intruding the Southalpine Variscan basement and occasionally also cutting through Lower Jurassic sediments of the Southalpine cover series (Bigioggero et al. 1981). Some of these dikes have phenocryst assemblages of plagioclase and amphibole plus minor biotite and clinopyroxene. Others are characterised by phenocryst assemblages of plagioclase, biotite, minor hornblende and rare quartz set in a groundmass mainly composed of plagioclase and quartz
Volcanic rocks
Between the Sesia-Lanzo Zone and the Ivrea-Verbano Zone, along the Canavese Line (belonging to the PFS), a volcano-sedimentary unit is found laying unconformably on the “Micascisti Eclogitici” and representing part of the non-metamorphic Tertiary cover of the Sesia-Lanzo Zone. It is composed of lava flows, pyroclastic and epiclastic deposits, essentially agglomerates, tuffs, and breccias. Lava flows are represented by andesites and subordinated basaltic andesites with a high-K calc-alkaline affinity and trachyandesites and trachydacites with shoshonitic affinity (Callegari et al., 2004). All rocks are porphyritic and show variable phenocryst mineralogy given by single pyroxene (orthopyroxene), two-pyroxene, two-pyroxene plus hornblende and two-pyroxene plus hornblende and biotite. Olivine occurs in basaltic andesites. Trachyandesites and trachydacites exhibit sanidine and phlogopite or biotite as phenocrystic phases together with plagioclase, augite, Fe-Ti oxides, apatite, and rare orthopyroxene, the latter being restricted to trachyandesites. Groundmass minerals consist of sanidine, quartz, clinopyroxene and opaque grains in a minute-microgranular matrix (Callegari et al., 2004).
Hunziker (1974), Scheuring et al. (1974) and Zingg et al. (1976) found total rock K/Ar ages ranging between 33.3 and 29.5 Ma. These dates were recently confirmed by a precise U-Pb zircon age determination yielding a value of 32.6 Ma (Kapferer at al., 2009).
Geochemistry of dike and volcanic rocks
The dike and volcanic rocks in the Western Alps range from calcalkaline to shoshonitic and ultrapotassic (Fig. 2a). Mafic ultrapotassic dikes have a lamproitic affinity (Venturelli et al., 1984; Peccerillo & Martinotti, 2006) and are variably enriched in silica (SiO2 ~ 44-56%). As all lamproites, they are characterized by relatively low CaO (~ 3.1-8.4%), Al2O3 (~ 8.6-12.0%) and Na2O (~ 0.7-1.9%), and high K2O/Na2O (~ 3.1-10.5) and K2O/ Al2O3 (~ 0.4-1.1). They also show high MgO (~ 7.0-13.1%), Ni (~ 93-460 ppm) and Cr (~ 309-933 ppm) denoting a mantle origin. FeOtot, MgO, CaO together with most of the compatible elements (e.g., Sc, V, Cr, Co) decrease markedly with increasing silica content, whereas TiO2 and, to a lesser extent, Na2O increase. Moreover, K2O and several incompatible trace elements (e.g., Rb, Th, U, Nb, Pb, Zr) exhibit steep positive correlations with SiO2. Incompatible trace element patterns normalized to primordial mantle compositions (Fig. 3) are strongly fractionated with very high enrichments in Cs, Rb, Th, U and Pb, distinct Ta, Nb, and Ti negative anomalies and slight depletion in Zr and Hf. Sr is depleted relative to the LREE. REE patterns show high enrichments in LREE (La ~ 200-600 times chondrite) relative to HREE (LaNYbN ~ 18.9-48.2), small Eu negative anomalies and typically upward-convex LREE patterns.
The basaltic to latitic dikes and lavas associated with lamproites in the Sesia-Lanzo Zone, are less enriched in potassium and have a high-K calcalkaline to shoshonitic affinity, with only a few calcalkaline dikes (Fig. 2a). The dikes from the Southalpine domain mostly have HKCA affinity, with minor CA and SHO occurrences (Fig. 2a). Dikes and lavas from both areas generally exhibit comparable concentrations and trends for most major elements, with dikes from the Southalpine domain showing slightly higher Na2O and lower FeOtot compared to dikes and lavas from the Sesia-Lanzo zone.
Compared to lamproites, CA and SHO rocks have markedly higher Al2O3 and Na2O and lower TiO2, MgO and P2O5. Moreover, incompatible trace elements but also Ni, Cr are much more enriched in lamproites. Primordial mantle-normalized spider-diagrams for the most mafic rocks (MgO > 3%) display similar shape, but incompatible trace element abundances increase from CA to SHO and to lamproitic rocks.
In summary, available data show that there is an increase in incompatible element contents from calcalkaline to ultrapotassic rocks. However, the mantle-normalised pattern of all these rocks is remarkably similar.
Lamproitic dikes have extremely high and variable initial Sr isotope ratios (~ 0.7121-0.7216) and low initial Nd isotope ratios (~ 0.51199-0.51219; Fig. 4a). The calcalkaline to shoshonitic dikes display much lower 87Sr/86Sr (~ 0.7059-0.7122), and higher 143Nd/144Nd than lamproites (Fig. 4a). Granodioritic dikes from the north-eastern part of the Sesia-Lanzo zone display initial 87Sr/86Sr around 0.7145.
Overall, Sr-Nd isotopic signatures are far away from typical mantle composition, suggesting an important though variable role of upper crustal components in the origin of these magmas. Lamproites exhibit a positive correlation between 87Sr/86Sr and SiO2. However, it is unlikely that such a feature depends on shallow level evolution processes such as bulk assimilation of crustal rock or AFC. These processes should generate a sharp decrease of compatible elements, a feature that is not observed in the Western Alps lamproites. Therefore, it is likely that both the high values of 87Sr/86Sr and their increase with silica reflect pristine compositional characteristics of the primary melts.
Pb isotope ratios of lamproites and CA-SHO dikes and lavas show similar initial values (Fig. 4b,c). They are moderately radiogenic and close to upper crustal values (206Pb/204Pb = 18.58-18.75; 207Pb/204Pb = 15.64-15.72; 208Pb/204Pb = 38.74-39.08). One basaltic calcalkaline dikes has been reported with distinct Pb isotope compositions (206Pb/204Pb=18.84, 207Pb/204Pb=15.60, 208Pb/204Pb=38.19; Fig. 4b,c). This sample also displays the lowest 87Sr/86Sr (0.7059) and highest 143Nd/144Nd (~ 0.51242) as well very high MgO, Cr and Ni contents.
Volcanic clasts in flysch deposits
In the External Western and Central Alps, the Oligocene flysch deposits of the Helvetic-Dauphinois Zone, are characterized by volcanic clast-rich layers containing lava fragments mainly of basaltic andesitic and andesitic composition with minor dacites and rhyolites, as well as isolated igneous mineral fragments such as amphibole, clinopyroxene, plagioclase and biotite. Plagioclase, clinopyroxene and minor magnetite are the most common mineral assemblages found in the basaltic andesites. Andesites show phenocryst mineral assemblages dominated by plagioclase plus clino- and orthopyroxene some hornblende and magnetite. Dacites have phenocrysts of plagioclase, amphibole and biotite with subordinated clinopyroxene. Rhyolites are scarce and mainly represented by quartz-biotite-sanidine volcanic fragments with pumiceous textures (Ruffini et al., 1997).
Ar/Ar analyses on amphiboles from the Champsaur Sandstone yielded two plateau ages of 32.5 Ma and 34.3 Ma (Boyet et al. 2001), while Ar/Ar dates from amphibole separates of the Taveyanne Sandstone cluster between 30.5 and 32.5 Ma (Fischer & Villa, 1990; Ruffini et al., 1997; Boyet et al., 2001).
The clasts have calcalkaline and high-K calcalkaline affinity (Fig. 2a). Variation trends of major elements versus SiO2 mostly overlap with those of calcalkaline-shoshonitic rocks outcropping in the Internal Western Alps. The mafic samples (MgO > 3%) have low K2O/ Na2O ~ 0.3-0.6 (Fig. 2b). Incompatible element abundances plot generally close the lower limits of the calcalkaline and high-K calcalkaline rocks from the Southern Alps and Sesia-Lanzo Zone. Primordial mantle normalized diagrams (Fig. 3a) reveal fractionated incompatible trace element patterns, similar to the ones displayed by the calcalkaline rocks from the Internal Western Alps, with negative anomalies in Nb, Ta and Ti and positive spikes of Pb and Sr. Initial 87Sr/86Sr ratios (~ 0.7057-0.7075) are lower, whereas initial 143Nd/144Nd (~ 0.51277-0.51244) ratios are higher than other Western Alps Tertiary igneous rocks (Fig. 4a). Pb isotope composition are highly variable and include the lowest values reported for the whole Tertiary Alpine magmatism (206Pb/204Pb ~ 18.33-18.80, 207Pb/204Pb ~ 15.55-15.65 and 208Pb/204Pb ~ 38.29-38.79; Fig. 4b,c). δ18O values measured on clinopyroxene separates range between +5.9-6.1‰ (Boyet et al., 2001), close to typical mantle values.
Petrogenesis of Western Alps orogenic magmatism
Tertiary orogenic igneous rocks in the Western Alps have variable degrees of enrichment in potassium, ranging from calcalkaline to ultrapotassic lamproitic. Most of the occurrences consist of intrusive bodies of variable size, from dikes to small plutons. A few effusive products are found in the Sesia-Lanzo zone. Calcalkaline basaltic andesites, andesites to dacite and rhyolite are found as clasts in the external Alps flysch deposits, testifying to the occurrence of volcanic structures that have been dismantled by erosion.
Contents of incompatible elements increase from calcalkaline to lamproitic rocks. The same trend is also shown by Sr isotopic ratios, whereas 143Nd/144Nd has an opposite tendency. Lead isotopes are poorly variable. All radiogenic isotope ratios are intermediate between crust and mantle, and sometimes well within the range of typical upper crustal rocks. Yet, all the rocks are of ultimate mantle origin, inasmuch as the most mafic members have high to very high MgO, Mg# and ferromagnesian element contents. Finally, incompatible element patterns of Western Alps orogenic rocks are very similar in shape to each other, although absolute abundances of elements change strongly. These patterns are characterised by negative anomalies of HFSE, but also by a relative deficiency in Sr and, in some cases, Ba. Overall, they resemble patterns of some upper crustal rocks, such as metapelites and granites (Peccerillo & Martinotti, 2006). Therefore, the bulk of trace element and radiogenic isotope data clearly point to the contribution of both mantle and upper crustal components in the origin of these magmas (Venturelli et al., 1984; Peccerillo & Martinotti, 2006; Owen, 2008; Prelević et al., 2008; Conticelli et al., 2009a). In principle, mantle-crust interaction may have occurred by assimilation of crustal rocks during of magma emplacement. However, this would imply some sort of increase of crustal-like signatures with decreasing MgO and other compatible elements, a feature that is not observed in the rocks. Therefore, there is a general agreement that, although magma contamination was occurring in several cases, the bulk of mantle-crust interaction occurred by addition of upper crustal material to the mantle wedge during the Cretaceous to Oligocene subduction of the European plate beneath the northern African margin. The presence in the Western Alps of deeply subducted upper crustal rock (Dora Maira massif; Compagnoni 2003) represents a compelling evidence supporting this hypothesis.
The ultrapotassic dikes display the highest incompatible element abundances along with the highest Sr- and lowest Nd-isotope ratios. Therefore, these rocks reveal the strongest participation of upper crustal material to magma genesis. Shoshonitic and calcalkaline rocks have less extreme compositions than lamproites, testifying to a lesser contribution of crustal end-member to magma origin. This can be explained by assuming that different amounts of upper crustal material contaminated various sectors of the mantle wedge during subduction. Melting of such a heterogeneous source gave different types of magmas displaying similar patterns but variable incompatible trace element abundances and radiogenic isotope signatures (Peccerillo & Martinotti, 2006).
Data reported previously have shown that there are, however, also important differences in the abundances of some major elements among the Western Alps rocks. In general, lamproites have lower Al2O3, CaO and Na2O than coexisting shoshonitic and calcalkaline rocks. Low abundances of these elements suggest that the source rocks were depleted in these components. This, in turn, suggests that the mantle source of lamproitic magma was represented by a harzburgite, i.e. a peridotite depleted in clinopyroxene. Such a composition might be determined by an older melting event and extraction of basaltic magma. In contrast, higher Al2O3, CaO and Na2O of calcalkaline and shoshonitic rocks are consistent with partial melting of less depleted (higher Al2O3 and Na2O) mantle peridotite (lherzolite), which had not been affected by previous melting events (Venturelli et al., 1984). Therefore, a scenario for the origin of calcalkaline to lamproitic magmatism in the Western Alps would be the one in which a harzburgitic to lherzolitic mantle wedge was heterogeneously contaminated by upper crustal material from the subducting European plate. Melting of various proportions of these sources gave different types of orogenic magmas.
Another hypothesis, not necessarily alternative, could be that variable compositions are the effect of different degrees of partial melting of the metasomatic mantle wedge. According to this hypothesis, upper crustal material introduced into the upper mantle generated veins that infiltrated depleted mantle rocks. Low degrees of partial melting would preferentially affect the vein, giving ultrapotassic magmas with strong crustal signatures. Increasing partial melting would involve normal mantle rocks producing depleted liquids that dilute early formed melts to give calcalkaline and shoshonitic magmas (Conticelli et al., 2009b).
The crustal material which was responsible for mantle modification was likely metapelitic s.l. in composition, as discussed earlier. This might be represented by 1) oceanic sediments brought down by subduction of European continental crust during the Alpine orogeny (Venturelli et al., 1984); 2) crustal material eroded from the acretionary prism of the overriding Adria continental margin by the low-angle subducting European slab (Peccerillo & Martinotti, 2006); 3) fluids or melts derived from pelagic sediments subducted during the closure of the Alpine Tethys Ocean in the late Cretaceous to early Tertiary (Owen, 2008); and 4) more in general, subduction of siliciclastic sediments combined to variable proportions of sedimentary carbonate component (Prelevic et al., 2008; Conticelli et al., 2009a).
Melting was both coeval and younger than subduction processes. In particular, the bulk of dike magmatism took place during thinning of the underlying lithosphere and the resulting upwelling of asthenosphere, which may be related to slab breakoff or to episodic retreat of the subduction zone hinge, due to slab rollback (von Blanckenburg & Davies, 1995; Beltrando et al. 2010).
Central Alps
Magmatism in the Central Alps is mainly represented by the Bregaglia (Bergell) pluton, intruding Penninic and Austroalpine units at the eastern margin of the Lepontine Dome (Fig. 1). It is a composite structure consisting mainly of a biotite-hornblende-tonalite margin and a biotite-granodiorite core. Other rocks include some gabbros and ultramafic cumulates (hornblendites and pyroxenites), mafic dikes, aplites and some pegmatites. A separate peraluminous intrusion, the Novate granite, crosscuts fabrics of the Bregaglia tonalite (Gulson, 1973; Trommsdorff & Nievergelt, 1983; von Blanckenburg et al., 1992). According to zircon, allanite and titanite U-Th-Pb dating, ages of the Bregaglia main plutonic body range between ~ 33 Ma and 30 Ma, and decrease from gabbros and tonalites to the inner granodiorite (von Blanckenburg et al., 1992; Oberli et al., 2004; Gregory et al., 2009).
The Novate stock is a garnet-bearing two-mica granite apparently not genetically related to the main Bregaglia pluton. Monazite and zircon U-Pb dating gave ages of ~ 26-24 Ma (Köppel & Grünenfelder, 1975; Liati et al., 2000).
Swarms of mafic dikes crosscut both the Bregaglia intrusives and the regional metamorphic and folded country rocks (Suretta nappe, Margna nappe and Malenco ophiolitic serpentinite) to the north and southeast of the pluton (Nievergelt & Dietrich, 1977; Gautschi & Montrasio, 1978; Wenk, 1980; Diethelm, 1990). The dikes cutting the Bregaglia country rocks are mainly basalts and basaltic andesites. No radiometric ages have been published, but some dikes show thermal metamorphism when occurring within the Bregaglia contact aureole, and probably represent the first phase of magmatism in the region (Nievergelt & Dietrich, 1977; Gautschi & Montrasio, 1978; Wenk, 1980). Mafic dikes within the Bregaglia pluton were emplaced when the main plutonic body was only partially solidified (Diethelm, 1990). Some of these dikes are lamprophyres with hornblende, K-feldspar, plagioclase, clinopyroxene and biotite.
Granitic boulders from the Bregaglia massif have been recovered in the Oligocene-Miocene molasse of the Po Plane (Gonfolite Lombarda Group; Gulson, 1973; Oschidari & Ziegler, 1992), indicating that the exhumation and erosion of the intrusive massif must have been rapid.
Geochemistry and petrology
The Bregaglia plutonic rocks are calcalkaline and high-K calcalkaline. Mafic dykes range from tholeiitic to potassic alkaline (lamprophyres) (Fig. 6a). The gabbro-tonalite-granodiorite intrusives and the tholeiitic to high-K calcalkaline dikes display similar contents for several major elements, although alkalies show larger variations and scattering for dikes. K2O/Na2O ratios for the tholeiitic-HKCA mafic rocks (MgO > 3%) are frequently below 0.5 whereas shoshonitic lamprophyres have K2O/Na2O up to 5 (Fig. 6b). However, these high ratios in some lamprophyres could not indicate ultrapotassic affinities, but may be related to diffusion of alkalies into lamprophyric magmas from residual melts of partially crystallised intruded granitoids (e.g., Blundy & Sparks, 1992).
Figure 6. Alkali-silica classification diagrams, Central Alps
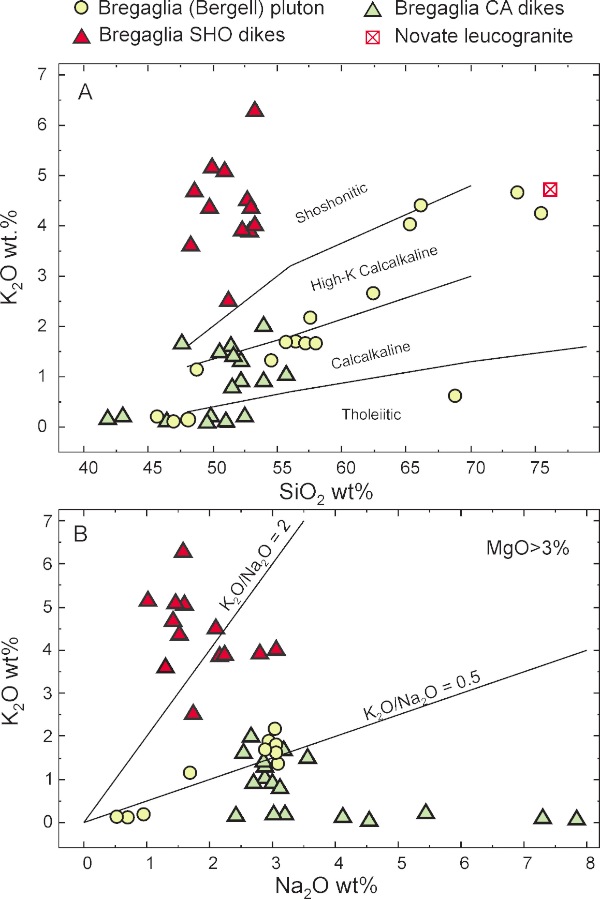
A - K2O vs. SiO2 classification diagram for the Bregaglia igneous complex and Novate granite; B and K2O vs. Na2O diagram for Bregaglia mafic rocks (MgO > 3%).
Incompatible element patterns normalized to primordial mantle composition of mafic plutonic rocks (Fig. 7) are fractionated with enrichments in Large Ion Lithophile Elements (LILE: Rb, Ba, LREE, etc.) with respect to High Field-Strength Elements (HFSE: Zr, Nb, Hf, etc.).
The tholeiitic to HKCA dikes display similar to somewhat less enriched incompatible element patterns as the mafic plutonic rocks (Fig. 7). Potassic dikes are moderately to strongly enriched in incompatible element in respect to other dikes and plutonic rocks. However, also MgO, FeO and ferromagnesian trace elements (Ni ~ 48-164 ppm; Cr ~ 193-612 ppm) are higher. Incompatible element patterns (Fig. 7) are fractionated, with Nb and Ti negative anomalies and slight depletion in Ba, Th and Sr.
Figure 7. Spider-diagrams, Central Alps
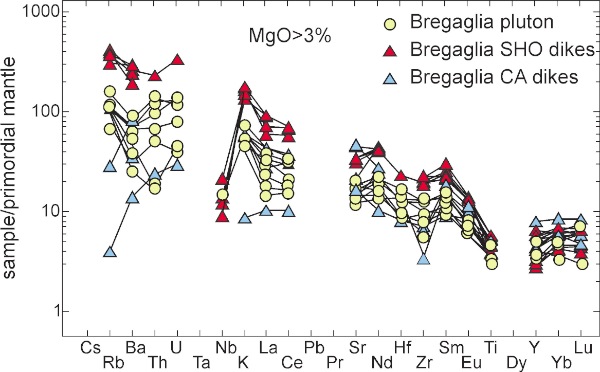
Incompatible element patterns normalized to primordial mantle composition for mafic rocks (MgO > 3%) from the Bregaglia igneous complex.
The Bregaglia pluton and associated dikes display a wide range of initial 87Sr/86Sr values, which increase with SiO2 from ~ 0.7055 to ~ 0.7160. A smooth curved (mixing) trend between mantle and crust is defined in the Sr-Nd isotope space (Fig. 14a). All these feature clearly point to important contributions of mantle and crust to the origin of these magmas. Initial Sr, Nd and Hf istopic compositions reported for one shoshonitic dike (87Sr/86Sr ~ 0.7073, 143Nd/144Nd ~ 0.51241 and 176Hf/177Hf ~ 0.28275; Stille et al., 1989; von Blanckenburg et al., 1992) also point to the same conclusion.
Pb isotope compositions for the Bregaglia tonalite-granodiorite suite define very small variations (206Pb/204Pb ~ 18.72-18.80, 207Pb/204Pb ~ 15.68-15.72 and 208Pb/204Pb ~ 38.75-38.98; Fig. 14b,c), and fall close or within the field of upper crustal rocks. Slightly higher values have been measured in pegmatites.
Whole-rock oxygen isotope compositions for the plutonic and hypabyssal rocks area variable (Diethelm, 1990; von Blanckenburg et al., 1992; Fig. 5). The cumulates and mafic dikes show δ18O ranging between +6.6‰ and +8.0‰, with the lowest values displayed by the cumulates and tholeiitic dikes. Oxygen isotope compositions increase progressively from ~ +7.5‰ to +9.9‰ passing from tonalites to aplites.
The Novate leucogranite shows lower Nd-isotope ratios (143Nd/144Nd ~ 0.51211-0.51215) than Bregaglia pluton (Fig. 14a), whereas initial 87Sr/86Sr (~ 0.7092-0.7106) and Pb isotopic signatures fall in the same range (Fig. 14b,c).
On the basis of Sr, Nd and O isotope geochemical data, von Blanckenburg et al. (1992) concluded that the Bregaglia magmas were formed by: (1) partial melting of an enriched lithospheric mantle that had been contaminated by crustal material, from which mafic magmas represented by mafic dikes, were extracted; and (2) subsequent simultaneous fractional crystallisation and contamination of the uprising magma. This second stage involved significant melting of the heterogeneous Alpine lower to middle crust to produce the main intermediate intrusive bodies, which typically show an increase in Sr-O isotopic signatures with increasing differentiation. From trace element data on brown amphibole and its clinopyroxene inclusions, Tiepolo et al. (2002) calculated that parental liquid compositions were characterized by marked enrichment of LILE, B, U and Th over HFSE and REE. Therefore, they proposed an origin for the Bregaglia parental melts by small degrees of batch melting of spinel-bearing MORB-type source metasomatized during Alpine and/or pre-Alpine times. Melting was triggered by B-rich fluids released by subducting oceanic or continental lithosphere. However, there was also open-system evolution dufing emplacement. This would explain the relatively high initial 87Sr/86Sr and δ18O displayed by the mafic rocks.
According to von Blanckenburg et al. (1992), the shoshonitic lamprophyres that intruded the partly solidified granodiorite, originated instead by low degree partial melting of a deep garnet-lherzolitic mantle source, strongly contaminated by subducted sediments.
The ~ 25 Ma Novate granite is not cogenetic with the Bregaglia body, but rather derived by partial melting of crustal rocks during late-Alpine decompression (Gulson, 1973; von Blanckenburg et al., 1992; Liati et al., 2000).
In conclusion, geochemical and isotopic data on rocks from Central Alps, suggest strong interaction between mantle and crust. However, in this sector, the role of mantle contamination by uppper crustal material seems much less strong than in the Western Alps, as testified also by overall lower Sr isotopic ratios of mafic rocks in the central sector. In contrast, shallow level magma contamination appears strong, as suggested by a general increase of Sr and oxygen isotopic data with increasing magma evolution. The role of the crust during intrusion of magma in the Central Alps is also highlighted by the occurrence of peraluminous crustal anatectic melts, which are rare or absent in the West.
Eastern Alps
At the western margin of the Eastern Alps, orogenic magmatism occurred in a wide area both south and north of the PFS. In the Southern Alps, the Adamello batholith is found together with a series of dikes and stocks occurring more to the west in the Orobic Alps. In the south-western Tyrol, numerous dikes and a few larger stocks are found intruding the Austroalpine units.
Moving east, the Tertiary magmatism is again localized along the Austroalpine-Southalpine boundary in the proximity of the PFS and most of the igneous activity occurred in the Austroalpine domain. South of the Tauren Window, the eats-west elongated plutonic bodies of Rensen, Monte Alto (Altenberg), Cima di Vila (Zinsnock) and Vedrette di Ries (Rieserferner) are found north of the Deferegger-Anterselva-Valles (DAV) tectonic line and very thin and elongated intrusive bodies occur along the northern sector of the Giudicarie line and along the Pusteria (Pustertal)-Gailtal line of the PFS. Numerous dikes also occur in a broad area to the south of the Tauren Window and volcanic to subvolcanic clasts are found in molasse deposits of the external Eastern Alps. Finally, intrusive magmatism continues in the easternmost Alps along the Periadriatic Zone, in Austria and Slovenia, or even more eastward, up to the Transdanubian Range (Kovács et al., 2007). Here, only Pohorje Mts. and Karavanke plutons will be shortly described (Pamic & Palinkas, 2000; Bellieni et al., 2010).
Adamello batholith
The Adamello batholith is the largest of the Tertiary intrusions along the PFS. It is located at the intersection between two important faults belonging to the PFS, i.e. the late- to post-magmatic Tonale Line and the Miocene Giudicarie Line (Fig. 1). The batholith intrudes the South Alpine Variscan basement and its non-metamorphic Permo-Triassic cover rocks and can be roughly subdivided into four individual plutons: Re di Castello, Adamello, Avio, and Presanella (Callegari, 1983). The individual plutons are composite bodies and were emplaced sequentially from the oldest units in the south (Re di Castello) to the youngest in the north (Presanella), from ~ 43 to 29 Ma (K/Ar and Rb/Sr ages: Del Moro et al., 1983a; amphibole Ar/Ar ages: Villa, 1983; zircon U/Pb ages: Hansmann et al., 1983; Hansmann & Oberli, 1991; Mayer et al., 2003; Schaltegger et al. 2009). The plutons are composed of prevailing hornblende-biotite-bearing tonalite, sometimes grading to granodiorite, with minor amounts of ultramafic cumulates (wherlites, hornblendites), gabbro, diorite, quartz-diorite, trondhjemite and granite. A few garnet-bearing tonalites have also been reported. The mafic rocks are mainly found at the borders of the batholith and primarily in the oldest and southernmost Re di Castello plutonic unit (Ulmer et al., 1983; Blundy & Sparks 1992; Tiepolo & Tribuzio, 2005). Microgranular mafic enclaves (MME) are ubiquitous (Blundy & Sparks, 1992) as well as swarms of different generations of dikes, which crosscut all the plutonic rocks. The mafic dikes are mainly represented by porphyritic to aphyric spessartites (phenocrysts of olivine, clinopyroxene and hornblende with a plagioclase-hornblende groundmass) grading to gabbroic porphyrites (Ulmer et al., 1983). Crustal xenoliths are mainly observed in the felsic rock types and are more abundant in the northern intrusions.
Geochemistry and petrology
Magmas of the Adamello batholith range from calcalkaline to high-K calcalkaline, passing from mafic to evolved compositions (Fig. 8a). Microgranular Mafic Enclaves (MME) have more potassic compositions
Major element abundances are variable, with MgO rapidly decreasing in the range SiO2 ~ 40-50%, from ultramafic cumulates to gabbros; CaO and FeOtot define smooth negative trends with silica. Mafic dikes have higher MgO than gabbros suggesting they may represent near-primary magmas (Ulmer et al., 1983).
K2O and K2O/Na2O ratios for the most mafic samples (MgO > 3%; Fig. 8b) are generally lower for samples from the Re di Castello pluton, with K2O/Na2O mostly below ~ 0.5, whereas rocks from the northern plutons display ratios chiefly in the range ~ 0.5-1.2. The differences depend on higher K2O in the northern plutons.
Compatible trace elements (e.g., Cr, Co, Ni) versus SiO2 exhibit curved fractionation trends similar to MgO. Excluding the extremely differentiated aplitic and pegmatitic samples, most of the incompatible trace elements (e.g., Rb, Ba, Th, Nb, LREE) increase more or less regularly with SiO2, whereas Sr, Zr and HREE show broadly bell-shaped trends. Samples form the southern Adamello show somewhat lower Rb and higher Sr, Ba and LREE concentrations than in he north. A number of mafic enclaves show pronounced enrichments in certain elements (e.g., K, Ba, Rb and Nb) relative to other Adamello rocks with similar SiO2. This has been related to equilibration of the mafic inclusions with interstitial melts in the host granitoid (Blundy & Sparks, 1992).
Figure 8. Alkali-silica diagrams for Adamello and Orobic Alps
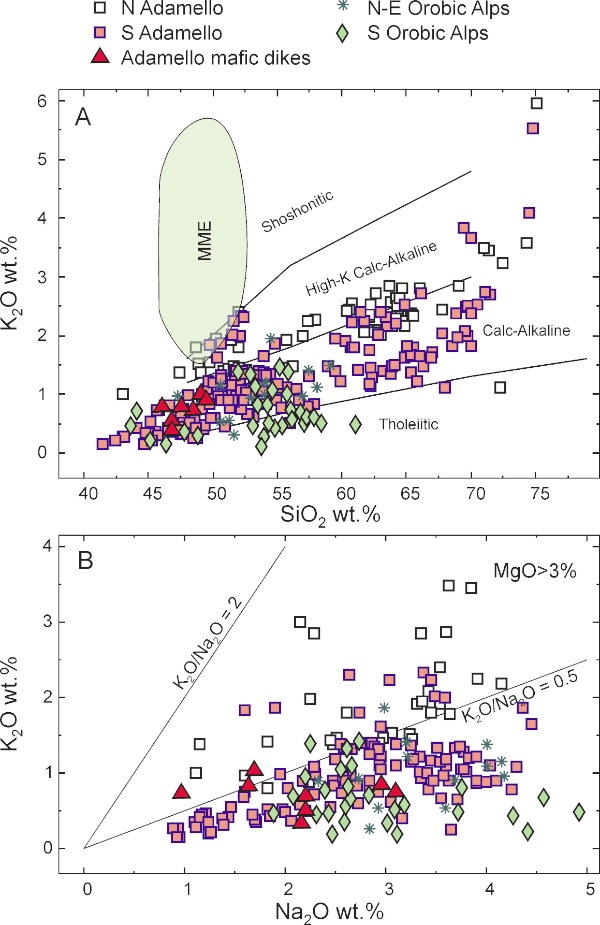
A - K2O vs. SiO2 diagram for the Adamello plutonic rocks and microgranular mafic enclaves (MME), and for Orobic Alps; B - K2O vs. Na2O for mafic rocks.
Incompatible trace elements of mafic rocks (MgO > 3%; Fig. 9) exhibit fractionated patterns with high enrichments in Ba, Rb, Th and U, Pb spikes, troughs at Nb and minor depletion in Ti. REE patterns reveal variable enrichments and fractionation. The Blumone olivine-gabbros have almost flat patterns (LaNYbN < 5) and show the lowest enrichments in LREE (La ~10 times chondrite), whereas other mafic rocks have La reaching ~100 time chondritic abundances.
Figure 9. Spider-diagrams of Adamello
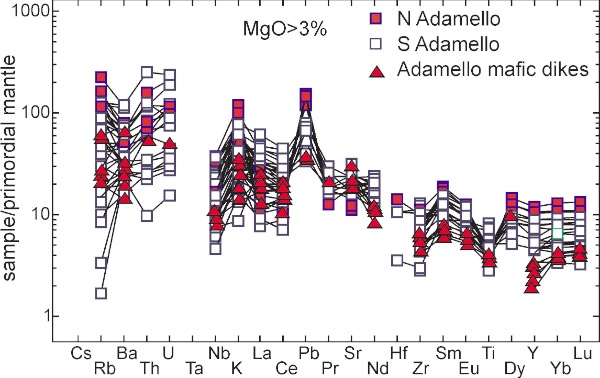
Incompatible element patterns normalized to primordial mantle composition for mafic rocks (MgO > 3%) from the Adamello intrusions.
At the batholith scale, whole-rock initial Sr isotope ratios do not exhibit any particular correlation with SiO2, but show a broad positive correlation with K and Rb. However, positive 87Sr/86Sr vs. SiO2 correlations are sometimes observed for single plutonic units. In general, initial 87Sr/86Sr increases from south to north, with the Re di Castello pluton showing less radiogenic compositions (87Sr/86Sr ~ 0.703-0.707) than the northern plutons (e.g., Adamello, Avio and Presanella; 87Sr/86Sr ~ 0.707-0.712). Northward increase of Sr isotope ratios is parallel to increase in some incompatible elements (e.g., K, Rb). The mafic dikes display initial 87Sr/86Sr ~ 0.7041-0.7064 (Cortecci et al., 1979; Dupuy et al., 1982).
Only a few whole-rock Nd and Pb isotope analyses have been published, mostly for southern Adamello rocks. 143Nd/144Nd for the Re di Castello pluton and one granodiorite from the Adamello pluton ranges from 0.51278 to 0.5121, showing a smooth negative trend with Sr isotopes, partly overlapping the mantle-crust mixing field of the Bregaglia complex (Fig. 14a). Pb isotope ratios of the Re di Castello pluton show relatively low 206Pb/204Pb (~ 18.47-18.58), with only extremely differentiated sample exhibiting more radiogenic values. 207Pb/204Pb and 208Pb/204Pb fall within the range displayed by the bulk of the Alpine magmatism (Fig. 14b,c) (Cortecci et al., 1979; Dupuy et al., 1982; Kagami et al., 1991).
Oxygen isotope compositions increase from δ18O ~ +5.9 to +8.0‰ values of the southern Re di Castello pluton to δ18O ~ +8.1 to +9.4‰ of the northern plutons (Fig. 5).
The northward increase of initial 87Sr/86Sr, δ18O and some incompatible element abundances (Cortecci et al., 1979; Dupuy et al., 1982; Del Moro et al., 1983a,b;Kagami et al., 1991) has been interpreted as indicating assimilation plus fractional crystallization (AFC) as a main evolutionary process during evolution of the Adamello massif, with the amount of contamination becoming progressively larger from south to north, and with time. Mg-tholeiitic to picritic parental magmas similar to that forming the mafic dikes, were suggested on the basis of mineral and bulk rock chemistry (Ulmer et al., 1983). These magmas were generated in a garnet-lherzolitic upper mantle, which had been metasomatically modified by fluids/melts released from subducting oceanic crust (Ulmer et al., 1983). However, the involvement of a sedimentary component during source metasomatism was suggested by Tiepolo & Tribuzio (2005). Finally, a metasomatized spinel-lherzolite source, similar to the one inferred for the Bregaglia parental melts suggested for Adamello by Tiepolo et al. (2002).
Orobic Alps magmatism
In the Southalpine domain west of the Adamello batholith (Orobic Alps; Fig. 1), several small bodies are intruded into the Southalpine crystalline basement and in the Permian to Lower Jurassic sedimentary cover (Crespi & Gandini, 1960; Casati et al., 1976; De Michele & Zezza, 1978; De Michele et al., 1983; Beccaluva et al., 1983; Fantoni et al., 1999). These consist of prevailing basaltic to andesitic dikes, some dacitic dikes, and minor gabbroic-dioritic laccoliths and stocks. In the central Valtellina area, immediately north of the Tonale line, the Triangia-Sondrio biotite-amphibole tonalite to granodioritie stock intrudes into Austroalpine basement rocks.
Basaltic-andesitic dikes, some bearing biotite, located in the northern (Valtellina area) and eastern (Val Camonica area) margins of the Orobic Alps, are probably genetically related to the Triangia-Sondrio and Adamello intrusions, respectively (Liborio & Mottana, 1969). However, no radiometric dating and very few geochemical studies have been undertaken on these dikes. Poorly constrained hornblende and whole rock K-Ar ages ranging between 64-36 Ma have been published by Zanchi et al. (1990) and Fantoni et al. (1999) for andesitic dikes and gabbro-diorite stocks from the Bergamo area, in the southern Orobic Alps. For the same rocks, more reliable middle to late Eocene U-Pb zircon ages have been recently reported by Zanchetta et al. (2009). These dates coincide with emplacement ages obtained for the oldest products of the Adamello massif.
Geochemistry and petrology
The few data on the Orobic Alps magmatism reveal predominant tholeiitic-calcalkaline affinities (Fig. 8a). Major and trace element variations showsome scattering, with MgO, TiO2, FeOtot and CaO decreasing more or less constantly with magma differentiation. Trace element data on rocks from the northern-eastern Orobic Alps display positive correlations for Ba, Rb and Zr with SiO2, overlapping with values reported for the Adamello rocks. REE fractionation and LREE enrichments (La ~10-30 times chondrite) exhibited by dioritic rocks from the southern Orobic Alps is relatively higher (LaNYbN ~ 21-30) than in similar rocks from the Adamello batholith.
South-Western Tyrol magmatism
North of the Adamello batholith, in the south-western Tyrol area (Fig. 1), numerous dikes and a few larger quartz-diorite stocks intrude the Austroalpine basement rocks (Ötztal-Stubai and Ortler-Campo nappes) or sometimes the Mesozoic sedimentary sequences (e.g., Ortler Group; Gatto et al. 1976, Beccaluva et al., 1979; Dal Piaz et al., 1988; Purtscheller & Mogessie, 1988; Mair & Purtscheller, 1995). Some dikes are also found intruding Southalpine rocks (e.g., Permian Bressanone, Brixen, granite; Purtscheller & Mogessie, 1988).
The dikes are mainly basaltic andesites and andesites with subordinate basalts, dacites and rhyolites. Basaltic andesites, andesites and the dioritic stocks contain hornblende, calcic plagioclase with minor biotite, clino- and orthopyroxene, K-feldspar and quartz. Most dikes contain also crustal xenoliths and mafic enclaves, sometimes showing cumulate texture (Mair & Purtscheller, 1995). Magmatic garnet is observed in some andesitic to rhyolitic dikes from the Merano area along the Periadriatic Line (Gatto et al., 1976; Purtscheller & Mogessie, 1988). Dal Piaz et al. (1988) reported Rb/Sr biotite ages for the quartz-dioritic stocks in the range ~ 32-30 Ma. A similar U/Pb zircon age of ~ 31.9 Ma was obtained by Müller et al. (2001) for a garnet-muscovite-bearing granitic dike. However, Gatto et al. (1976) obtained for andesitic and dacitic dikes K/Ar total rock ages of ~ 89 Ma, ~ 48 Ma and ~ 32 Ma. If correct, these ages would represent the only evidence for Cretaceous subduction-related magmatism in the Alps.
Very thin and elongated dioritic-tonalitic bodies occur in the Eastern Alps along the Austroalpine-Southalpine boundary, in proximity of the northern Giudicarie and Pusteria-Gailtal lines of the PFS. These bodies have been traditionally reported as tonalite “lamellae” (Dal Piaz, 1926). They often display a foliated texture and contain mafic enclaves (Martin et al., 1993 and reference therein). Rb/Sr ages obtained for the “lamellae” located along the northern Giudicarie Line (Rumo, Samoclevo and Mulles), north of the Adamello batholith, are in the range ~ 32-28 Ma (Sassi et al., 1985; Martin et al., 1993).
Geochemistry and petrology
Dikes and stocks form the south-western Tyrol display mainly calcalkaline to shoshonitic affinity. The “lamellae” are calc-alkaline (Fig. 10a).
Most of the Austroalpine calcalkaline and high-K calcalkaline dikes and stocks have low TiO2 (<1%), whereas higher values (TiO2 ~2.5 %) are displayed by some of the very mafic (MgO ~ 10-11%) high-K calcalkaline and shoshonitic rocks. The tonalite “lamellae” have intermediate Ti contents, similar to the tonalitic rocks from the Adamello. A few high-TiO2 (~1.6-1.7%) andesitic-latitic dikes contain up to 7% Na2O. The least evolved intrusives (MgO > 3%) have K2O/Na2O ratios increasing progressively from calcalkaline to shoshonitic samples, but never exceeding about 2 (Fig. 10b). The “lamellae” have ratios ~ 0.5 (Fig. 10b), similar to Adamello and Bregaglia tonalitic rocks.
Trace elements are rather scattered, with some of the highly incompatible elements (e.g., Rb) increasing constantly with SiO2, and Cr and Ni showing an opposite trend. In general, shoshonitic rocks together with some HKCA samples exhibit the highest concentrations for several incompatible elements.
Figure 10. Alkali-silica diagrams of south-western Tyrol magmatism, Eastern Alps
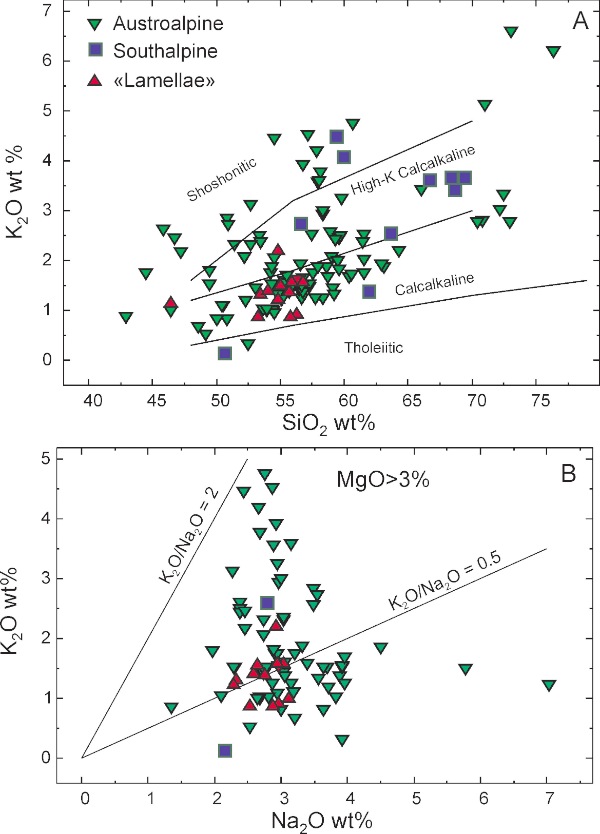
A - K2O vs. SiO2 classification diagram, and B - K2O vs. Na2O diagram for south-western Tyrol Tertiary igneous rocks.
Primordial mantle normalized diagrams (Fig. 11) for calcalkaline-shoshonitic mafic rocks (MgO > 3%) reveal similar fractionated patterns with enrichments of LILE, Pb positive spikes and Nb, Ta and Ti negative anomalies. Moreover, they commonly show Ba negative anomalies. REE patterns show variable fractionation (LaNYbN ~ 3.1-46.1), generally increasing from calcalkaline to shoshonitic rocks. The “lamellae” show REE patterns similar to the Adamello and Bregaglia calcalkaline rocks with relatively low fractionation (LaNYbN ~ 5.4-6.0).
Figure 11. Spider-diagrams for south-western Tyrol, Eastern Alps
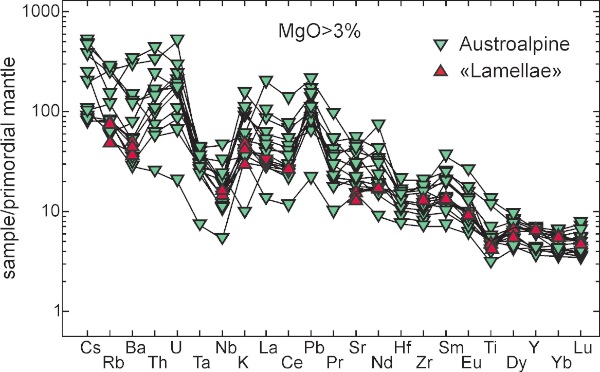
Incompatible element patterns normalized to primordial mantle composition for mafic rocks (MgO > 3%) from the south-western Tyrol area.
Initial Sr isotope ratios are extremely variable and show a rough tendency to increase with SiO2. The Southalpine dikes have higher 87Sr/86Sr (~ 0.7148-0.7203) than the Austroalpine dikes and stocks (87Sr/86Sr ~ 0.7051-0.7125) and display scattering in Sr-Nd isotope space (Fig. 14a). The “lamellae” have high initial 87Sr/86Sr ~ 0.7081-0.7109, comparable to values reported for tonalitic rocks from the northern Adamello plutons and Bregaglia massif.
Most authors agree that the widespread, mostly andesitic, magmatism occurring in the south-western Tyrol area is related to subduction processes (e.g., Gatto et al., 1976; Beccaluva et al., 1979; Dal Piaz et al., 1988; Macera et al., 2008). Based on geochemical and Sr isotopic constraints, Dal Piaz et al. (1988), proposed that at least two mantle derived parental magmas, respectively with calcalkaline and high-K calcalkaline-shoshonitic composition, were involved in the petrogenesis of dikes and stocks of the Austroalpine domain in the south-western Tyrol area. According to these authors, parental magma were generated in heterogeneous mantle sources, which were affected by different degrees of mantle metasomatism induced by fluid/melts released from an oceanic lithosphere during Cretaceous subduction. Mantle partial melting took place by thermal restoration occurred during Oligocene extensional processes. Magma evolution processes took place by fractional crystallisation and heterogeneous assimilation of various amounts and types of crustal material. These determined high initial 87Sr/86Sr of the igneous products as well as scattering of trace element and Sr isotope values, a feature that was increased by post-magmatic elemental transfer by fluid phases. According to Purtscheller & Mogessie (1988) the dikes found in the Southern Alps, just east of the Giudicarie line, could be cogenetic with the nearby calcalkaline-shoshonitic rocks from the Austroalpine domain, but suffered higher crustal contamination during differentiation.
Figure 12. Alkali-silica classification diagrams, Eastern Alps
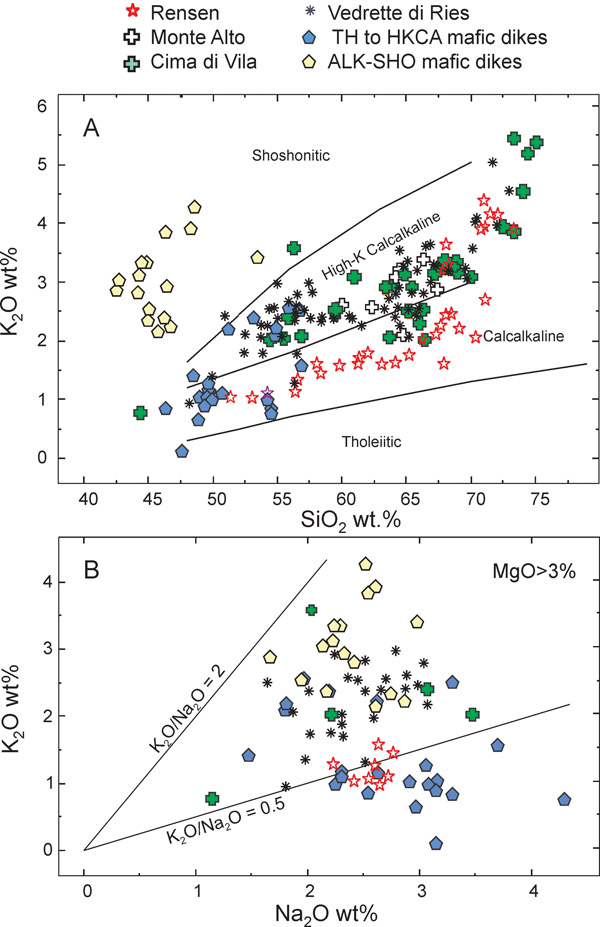
A - K2O vs. SiO2 classification diagram; B - K2O vs. Na2O diagram for the plutons (Rensen, Monte Alto, Vedrette di Ries and Cima di Vila) and mafic dikes located south of the Tauren Window.
Rensen and Monte Alto (Altenberg) plutons
The Rensen pluton is mainly composed of granodiorites with minor tonalites. Quartz-diorites occur as small elongated bodies within the tonalites and granodiorites; two-mica granites with a gneissic texture make up a small mass. Tonalites and granodiorites are made of plagioclase, biotite, epidote and quartz with minor amphibole, K-feldspar and rare muscovite. Garnet, typically enclosed in plagioclase, is rarely observed in quartz-diorites (Bellieni et al., 1984; Barth et al., 1989; Bellieni et al., 1991). Allanite Th/Pb and zircon U/Pb age determinations for quartz-diorites, tonalites and leucogranites give similar ages of 31.7 to 30.8 Ma (Barth et al., 1989).
The Monte Alto stock, located east of the Rensen massif (Fig. 1), is dominated by granodiorites with sporadic tonalites (Bellieni et al., 1984). A Rb/Sr age determined by whole rock plus biotite isochron is around 24-25 Ma (Borsi et al., 1979; recalculated by Sassi et al., 1985).
Geochemistry and petrology
The Rensen plutonic rock association displays a smooth calcalkaline fractionation trend from quartz-diorite to granodiorite with the leucogranite showing a high-K calcalkaline affinity; the Monte Alto tonalites and granodiorites are mostly HKCA (Fig. 12a).
Figure 13. Spider-diagrams, Eastern Alps
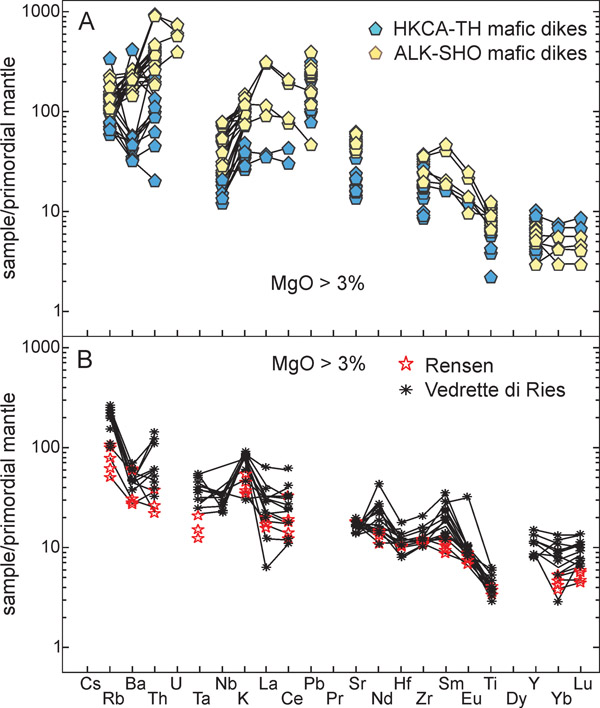
Incompatible element patterns normalized to primordial mantle composition for mafic rocks (MgO > 3%) from the Rensen and Vedrette di Ries plutons and for alkaline (ALK)-shoshonitic (SHO) mafic dikes located south of the Tauren Window.
Rensen and Monte Alto display smooth negative trends of TiO2, Al2O3, MgO, FeOtot vs. silica, and positive trends for Na2O. Monte Alto is more enriched in LILE (e.g., Rb, Sr, Th, LREE) with respect to the Rensen tonalites and granodiorites. Rensen exhibits positive trends for Rb, Ba and Ta vs. silica, with a marked increase in Rb and Ta passing from tonalite-granodiorite to leucogranite. Primordial mantle-normalized trace element diagrams for Rensen dioritic rocks with MgO > 3 % reveal fractionated patterns with moderate enrichments of LILE and Th over the REE and HFSE, troughs at Nb and Ti, small Sr positive anomalies and, frequently, Ba negative anomalies (Fig. 13). REE patterns for the Rensen mafic rocks show rather low LREE/HREE fractionation with LaNYbN mostly ~ 3.7-5.5.
Quartz-diorites to granodiorites from Rensen show poorly variable and relatively high initial 87Sr/86Sr ~0.7075-0.7081, whereas an abrupt increase in the Sr isotope ratios is observed in leucogranites (87Sr/86Sr ~0.7095-0.7110). Initial Nd isotope ratios decrease from 0.51236 to 0.51225, passing from quartz-diorite to leucogranite and some tonalites (Fig. 14a). Monte Alto displays initial 87Sr/86Sr similar or higher than the Rensen leucogranites (~ 0.7108-0.7113).
The different rocks from Rensen show rather homogeneous crustal-like Pb isotope compositions falling within the range defined by other Alpine plutonic rocks. The leucogranites show slightly higher 207Pb/204Pb and 208Pb/204Pb with respect to dioritic-tonalitic rocks (Fig. 14b,c).
Geochemical, isotopic and geochronological data suggest that Monte Alto and Rensen plutons are not comagmatic. According to Bellieni et al. (1984), high Sr contents and high HREE fractionation of Monte Alto rocks suggest an origin by melting of plagioclase-free, garnet-bearing deep crustal rocks, possibly followed by some degree of fractionation at shallower levels. An origin by crustal anatexis is also corroborated by the very high initial 87Sr/86Sr displayed by the Monte Alto tonalite-granodiorite. On the other hand, extensive interaction between mafic mantle-derived magmas and crustal material has been invoked in the genesis of the Rensen plutonic association (Barth et al., 1989; Bellieni et al., 1991). A multistage polybaric fractional crystallisation of a single type of dioritic magma, accompanied by variable interaction with the intruded crust, was suggested for the evolution of Rensen. This determined variable Sr isotopic signatures and different trends of compatible vs. incompatible elements (Bellieni et al., 1991).
Figure 14. Radiogenic isotope compositions, Central and Eastern Alps
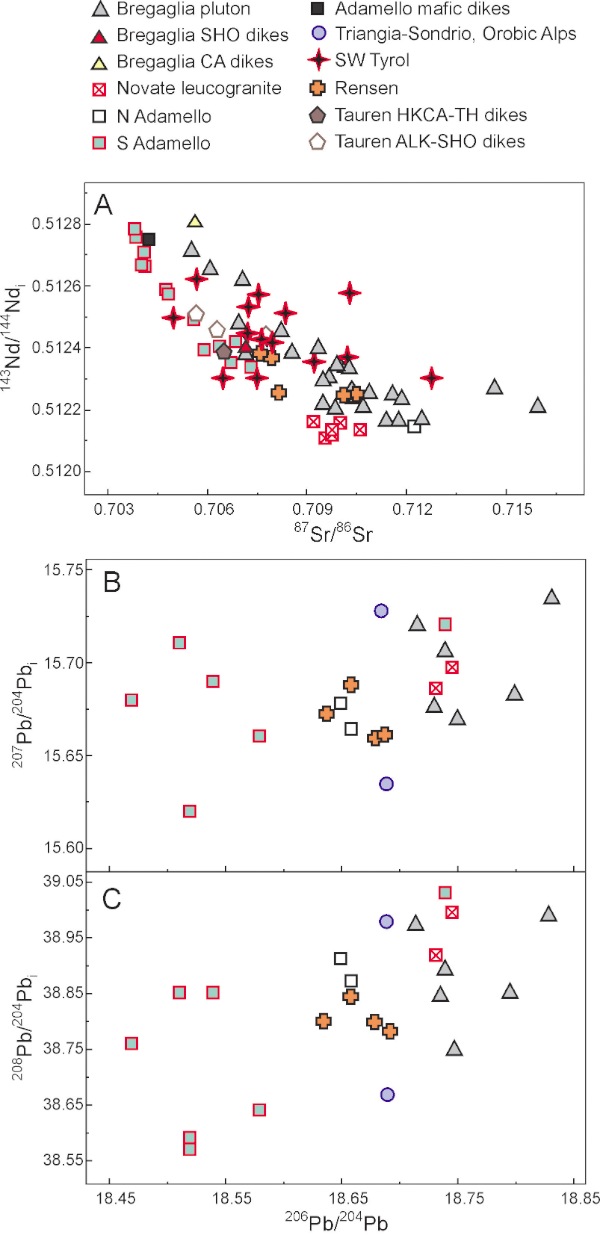
Sr-Nd-Pb isotopic compositions of Tertiary igneous rocks from Central and Eastern Alps.
The leucogranites show a strong crustal affinity both in terms of initial Sr-Nd isotopic compositions and occurrence of inherited pre-magmatic components in its zircon population. Accordingly, the bulk of this rock has been considered as crustal anatectic in origin (Barth et al., 1989; Bellieni et al., 1991).
Vedrette di Ries (Rieserferner) and Cima di Vila (Zinsnock) plutons
The Vedrette di Ries plutonic complex is composed mainly of granodiorite and tonalite consisting of various proportions of plagioclase, quartz, K-feldspar, hornblende and biotite. Garnet occurs in most tonalites and in some granodiorites. Small masses of granites and diorites, the latter containing some magmatic garnet, are distributed within granodiorites and tonalites. Dioritic to tonalitic microgranular enclaves, as well as metamorphic xenoliths are frequently found all over the pluton (Bellieni et al., 1981; Cesare et al., 2004). U-Pb ages of ~ 31.8-32.2 Ma were obtained on allanite by Romer & Siegesmund (2003), whereas a Rb-Sr whole-rock isochron yielded an age of ~ 31 Ma (Borsi et al., 1979; recalculated by Sassi et al., 1985).
The entire plutonic complex is cut by acidic (pegmatites and aplites) to basic lamprophyric dikes. The lamprophyres, cut both the plutonic rocks, including the aplite and pegmatite dikes, and the metamorphic country rocks and are probably not part of the Vedrette di Ries magmatic cycle (see below).
The Cima di Vila pluton is exposed along the DAV line (Fig. 1), close to the southern border of the larger Vedrette di Ries pluton and is mainly made up of biotite granodiorite surrounded by biotite-amphibole tonalite. Tonalites and granodiorites are cut by granitic and aplitic dikes and contain microgranular mafic enclaves and a few metamorphic xenoliths. Rare ultramafic enclaves with hornblenditic composition occur within the tonalitic rocks (Bellieni et al., 1989; Bellieni et al., 1996). A total rock and biotite isochron Rb/Sr age of ~ 29.5 Ma was published by Borsi et al. (1979).
Geochemistry and petrology
The Vedrette di Ries and Cima di Vila plutons display a common high-K calcalkaline affinity (Fig. 12a) and overlapping trends for major elements. The least evolved rocks (MgO > 3%) from both plutons, represented mostly by microgranular enclaves, exhibit variable but similar K2O/Na2O ratios (~ 0.4-1.8; Fig. 12b). These values are overall much higher than the Resen pluton.
Despite the almost identical major element characteristics the Vedrette di Ries and Cima di Vila plutons display important differences in terms of some trace element and Sr isotope compositions. Incompatible trace element patterns (Fig. 13) for selected mafic rocks (MgO > 3%) from the Vedrette di Ries pluton reveal elevated enrichments in the highly incompatible elements with Nb, Ta, Ti and small Sr negative anomalies. Moreover, general depletion in Ba and Th relatively to Rb and high K/LREE are observed.
Vedrette di Ries exhibits poorly variable 87Sr/86Sr values (~ 0.709-0.711), with only some aplitic dikes reaching 87Sr/86Sr ~ 0.715.
Cima di Vila shows a positive 87Sr/86Sr vs. SiO2 trend (87Sr/86Sr ~ 0.7058-0.7113), and only the most evolved samples display 87Sr/86Sr values overlapping the Vedrette di Ries pluton.
Based on trace element modelling, Bellieni et al. (1981) ruled out the possibility that rock suite may be the product of crustal melting as previously was proposed by Borsi et al. (1979) on the basis of Sr isotopic data. It was suggest that a mantle-derived parental melts went through a two-stage fractional crystallization process, with high pressure separation of garnet+hornblende followed by lower pressures of hornblende+plagioclase. Crustal assimilation also took place during fractionation (Bellieni et al., 1981).
Mixing between mafic mantle-derived melts and felsic crustal melts generated by melting within the deep crust was suggested as leading mechanism for Cima di Vila magmas by Bellieni et al. (1996). Granitic and aplitic derivative liquids were successively formed by fractional crystallisation and/or in situ separation of residual melt from an extensively crystallised body.
Dikes south of the Tauren Window
Numerous dikes occur in the area south of the Tauren Window and west of the Mölltal Line (Fig. 1). They are mostly intruded into the polymetamorphic Austroalpine basement, with a few also crosscutting the Austroalpine Mesozoic cover and the Southalpine basement along the Gailtal Line (Deutsch, 1984; Müller et al., 1992; Müller et al., 2000; Trepmann et al., 2004). Ages (K/Ar and Rb/Sr) are scattered and mostly fall between about 40 and 24 Ma (Deutsch, 1984; Steenken et al., 2000).
At least two generations of dikes have been distinguished: 1) porphyritic tonalitic (trondhjemitic) and granodioritic dikes that are not observed within the main Tertiary plutonic bodies; these are locally deformed and show a greenschist facies metamorphic overprint (Müller et al., 2000; Trepmann et al., 2004); 2) unfoliated and unmetamorphosed mafic dikes that locally cross-cut the main plutonic bodies as well as aplites and pegmatites (e.g., Vedrette di Ries; Deutsch, 1984; Steenken et al., 2000; Müller et al., 1992). Other dikes consist of aplites and pegmatites and are associated with intrusive rocks described above.
The tonalitic to granodioritic dikes are characterized by phenocrysts of magmatic garnet and/or muscovite (e.g., Trepmann et al., 2004) and gave U/Pb zircon ages ~ 31-32.5 Ma (Müller et al., 2000; Müller et al., 2001) and slightly younger Rb/Sr ages in the range 29.9-30.9 Ma (Müller et al., 2000). Among the mafic dikes, different groups have been distinguished. These include 1) porphyritic to aphyric CA-HKCA basaltic to andesitic dikes containing hornblende, plagioclase and minor augite and biotite; 2) amphibole-bearing and mica-bearing shoshonitic lamprophyres; 3) nepheline-normative to olivine-diopside-hyperstene-normative alkalibasaltic dikes containing kaersutite, Ti-pargasite/hastingsite, Ti-biotite, Ti-augite and alkalifeldspar (Deutsch, 1984; Müller et al., 1992).
Limited geochemical studies have been undertaken for the deformed and slightly metamorphosed tonalitic-granodioritic dikes, whereas the mafic dikes were subjected to more comprehensive studies of major, trace element and Sr-Nd isotope compositions. Because of this data bias, attention will be focused on the mafic dikes in the following section.
Geochemistry and petrology
Major element abundances are variable, with alkaline (ALK) dikes showing the lowest silica and highest TiO2 (~1.6-2.5%) and P2O5 (up to 1%). The subalkaline-transitional dikes range from tholeiitic to shoshonitic (Fig. 12a). A group of subalkaline samples is distinguished by high TiO2 (~2%), similar to the alkaline ones, together with relatively high FeOtot (~11%). K2O/Na2O ratios (Fig. 12b) are generally below 0.5 for the tholeiitic-calcalkaline products and comprised mostly between ~0.5-1.5 for the alkaline and high-K calcalkaline-shoshonitic dikes.
In general the mafic calcalkaline-shoshonitic dikes from south of the Tauren Window show major element compositions comparable with the basic magmatism from south-western Tyrol, except for slightly higher TiO2 and lower Al2O3 for the former.
The Na-alkaline and high-K calcalkaline-shoshonitic products show commonly higher concentration in most of the incompatible elements with respect to the tholeiitic-calcalkaline dikes. Alkaline and shoshonitic dikes have similar fractionated incompatible trace element patterns (Fig. 13) with negative anomalies of Nb, and spikes in Th and U. Tholeiitic-calcalkaline and high-K calcalkaline samples generally show greater troughs at Nb and Ti and often display Ba negative anomalies. REE patterns are variably fractionated.
The alkaline dikes display lower whole-rock and amphibole 87Sr/86Sr (~ 0.7056-0.7069) compared to the subalkaline dikes (87Sr/86Sr ~ 0.7065-0.7146). In the subalkaline rocks, the Sr isotope ratios determined an early crystallizing hornblende are generally lower (~ 0.7065-0.7080) compared to the whole-rock values (~ 0.7078-0.7145), suggesting magma contamination during crystallization. Initial Nd isotope ratios, only measured on amphibole, are higher for the alkaline samples (~ 0.51239-0.51245), but still away from typical mantle values.
According to Deutsch (1984), the mineralogical, geochemical and isotopic data displayed by the alkaline and subalkaline dikes are compatible with magma origin from an enriched sub-continental mantle variably metasomatized by LILE- and LREE-rich fluids. The high and variable 87Sr/86Sr particularly in the subalkaline dikes, indicates, a major contribution of crustal material in early evolutionary stages (Deutsch, 1984).
Müller et al. (1992), proposed that mantle metasomatism was achieved during Cretaceous subduction and melting occurred during a phase of extensional tectonics, post-dating continent-continent collision. Moreover, they suggested that the alkaline magma types may reflect a deeper mantle source due to asthenospheric upwelling.
Karavanke and Pohorje intrusions
The Pohorje Mts. and Karavanke plutons (Austria, Slovenia) represent the easternmost intrusions of the Periadriatic Zone. They are composed of dominant tonalites with subordinate granodiorites and rare diorites, showing CA and HKCA affinity (Pamic & Palinkas, 2000, with references; Bellieni et al., 2010). Ages are 28-30 Ma (Kovács et al., 2007). Major and trace element concentrations are not far from other intermediate to acid rocks of the Eastern Alps. Initial 87Sr/86Sr ratios range from 0.70656 to 0.70750 for Pohorje, whereas Karavanke rocks how values around 0.7075 (Pamic & Palinkas, 2000). Variation of major, trace element and Sr- and O-isotope data suggest that the rock suite of these plutons were formed by AFC processses starting from an olivine tholeiite parental magma. Based on element modelling, it has been suggested that parent magma were formed by melting of a slightly metasomatized garnet peridotite (Pamic & Palinkas, 2000).
External Eastern Alps flysch deposits
Fragments of volcanic or subvolcanic rocks occur as pebbles in alluvial conglomerates of late Oligocene to middle Miocene age in the Eastern Alps. According to Brügel et al. (2000), these clasts represent erosional products of volcanic edifices located in the vicinity of the PFS and genetically related to the widespread Oligocene plutonic activity of the Eastern Alps. They are porphyritic andesites and dacites composed mainly of plagioclase, amphibole, biotite and quartz, and showing high-K calcalkaline and shoshonitic affinity (Brügel et al., 2000). Whole rock K/Ar ages in the range 24 to 40 Ma and a more precise amphibole Ar/Ar age of 32.3 Ma have been found (Brügel et al., 2000).