Results
Northern Apennines
The Northern Apennines have been studied in details since the ‘80s and today they yield a very dense dataset obtained by different low-temperature thermochonological techniques: zircon fission-track (ZFT), apatite fission-track (AFT) and (U-Th)/He on apatite (AHe) (Fig. 7). Furthermore, a huge number of samples both from surface and bore-holes have been analyzed by the vitrinite reflectance technique (Fig. 8). These data have been integrated by some clay mineralogy analyses on sediments of the Cervarola succession (Botti et al., 2004; Aldega and Eberl, 2005; Fig. 9). Within the pile of Ligurian and Tuscan nappes, the coal rank increases generally from top to bottom, to reach the low-grade metamorphism in the lowermost nappe. Ro% values in the Ligurian nappe vary from 0.5 at surface to 1.8% in the Quara1d and Sestola1 wells (Reutter et al., 1983; Anelli et al., 1994). KI data from the Ligurian Nappe collected near Chiavari range from 0.25°Δ2θ in the Cravasco/Voltaggio unit to 0.49°Δ2θ in the Bracco/Val Graveglia unit suggesting a significant and gradual increase in metamorphic grade from the highest to the lowest unit within the tectonic pile (Leoni et al., 1996). The pressure conditions - estimated through the illite b0 parameter - range from 2-3 kbar (Bracco/Val Greaveglia unit) to ca. 7 Kbar (Cravasco/Voltaggio unit; Leoni et al., 1996).
Data from wells in the Macigno, Cervarola and Marnoso-Arenacea successions show values up to advanced organic metamorphism whereas at surface both vitrinite reflectance and thermochronology data show a general decrease of the burial conditions from Tyrrhenian to Adriatic coast. For each of the thermochronometer it is thus possible to go from totally reset to partially reset regions. This regional trend is disturbed only locally by young post-coalification tectonics. Some post-orogenic heating is connected with the magmatic activity of Late Miocene to Pleistocene age in Tuscany. Except for these post-orogenic thermal events, the main coalification cause can be generally ascribed to nappes emplacement during the Apennine orogeny in the Miocene. This feature is evident both in the central region of the chain (e.g., Botti et al., 2004) than in the external area where the maturity profile of the Palazzuolo1 well is nearly continuous across main thrusts (Anelli et al., 1994). On the other hand, late orogenic extensional tectonics is clearly younger than time of maximum burial throughout the chain.
Figure 7. Distribution of thermochronological data in the Northern Apennines
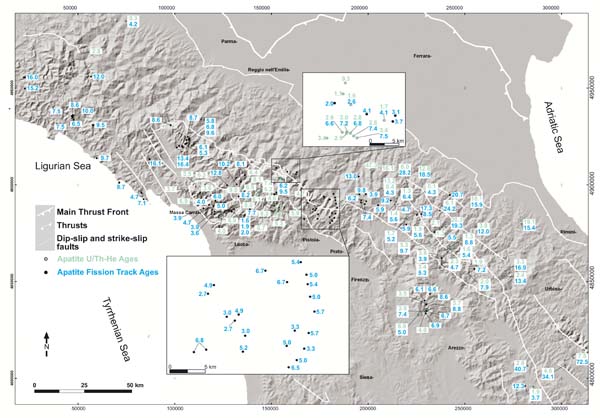
Digital Elevation Model of the Northern Apennines showing the distribution od apatite fission track and U/Th-He data. Simplified main geological features from APAT (2004) and Thomson et al. (in press). Coordinates in UTM European Datum 1950 33N.
Figure 8. Distribution of organic matter thermal maturity data in the Northern Apennines
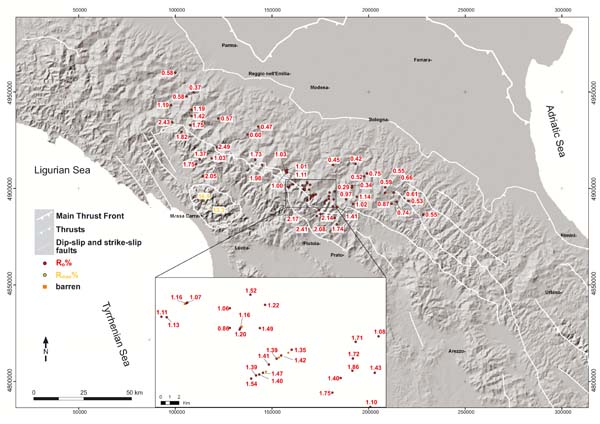
Digital Elevation Model of the Northern Apennines showing the distribution of vitrinite reflectance data. Simplified main geological features from APAT (2004) and Thomson et al. (in press). Coordinates in UTM European Datum 1950 33N.
Figure 9. Distribution of illite contents in mixed layers I-S data in the Northern Apennines
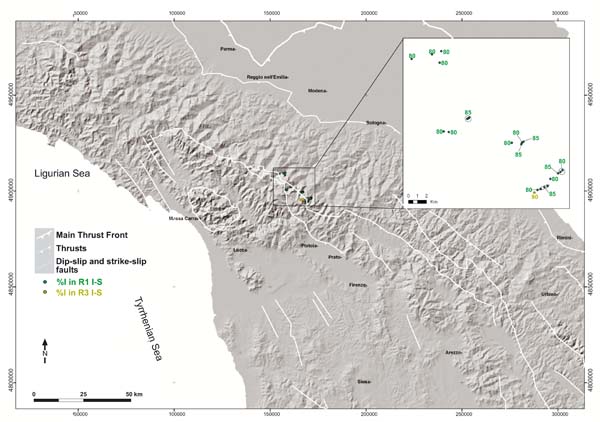
Digital Elevation Model of the Northern Apennines showing the distribution of illite contents in mixed layers I-S. Simplified main geological features from APAT (2004) and Thomson et al. (in press). Coordinates in UTM European Datum 1950 33N.
The shape of the maximum paleo-isotherms is nearly parallel to the chain axis with the noticeable exception of the Alpi Apuane window. This area represents the lowermost unit of the chain and therefore exhumed from larger depths than the surrounding units. Peak P-T conditions have been evaluated at 0.6-0.8 Gpa and 420-500°C (Massa unit; Molli et al., 2002). The gap in burial conditions with the sediments out of the window is quite relevant as here maximum temperatures never exceeded the total annealing temperature of fission tracks in zircon.
The less buried sediments are presently exposed along the thrust fronts, on the pro-side of the chain, where the AHe system has been locally not reset (i.e. burial temperatures were lower than about 60°C) and the Ro% data are in the order of 0.5%. The positions of reset fronts is not well defined as total resetting of apatite in both the AHe and AFT systems is not only influenced by temperature, but also by factors such as variation in apatite chemistry and radiation damage, and because of the low dip of this front, thus influenced by local topography. It is important to note that most of the data were obtained on the foredeep units involved in the thrust belt whereas only a few data are from the Ligurian and Epiligurian units. The thermal history of these latter units is very different from the underlying foredeep thrust belt but, although the few existing constraints, in general rocks were affected by low burial temperatures during formation of the Apennine wedge.
The exhumation history follows the same pattern observed for the burial conditions. In fact, both AFT and AHe techniques indicate a general eastward decrease of the exhumation ages (Fig. 10). Close to the topographic front, older detrital and mixed AHe and AFT ages occur, which then rapidly decrease to fully reset ages towards the core of the orogen. More in detail, the youngest AHe ages (ca. 1 to 2 Ma) have been detected close to the core of the range (Mt. Falterona and Mt. Cimone areas) whereas youngest AFT at about 4 Ma close to the reset front.
Figure 10. Map of contoured AHe and AFT ages
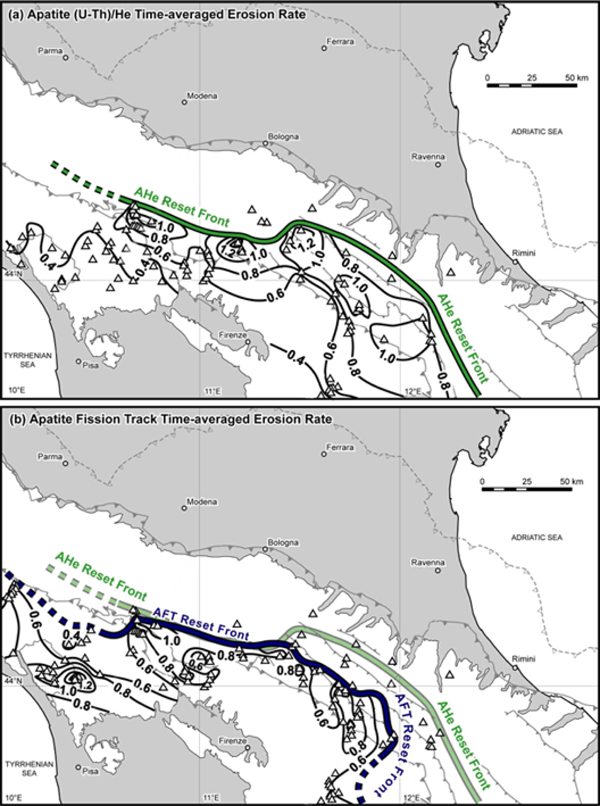
Map of contoured AHe and AFT ages, with approximate position of reset fronts (where ages are significantly younger than stratigraphic age, and show no evidence of mixed ages) - For AHe between 6 and 7 Ma, for AFT between 10 and 12 Ma. a: AHe time averaged erosion rate; b: AFT time averaged erosion rate. After Thomson et al. (in press) modified.
The onset of exhumation of the chain is recorded from the oldest ages, located west of the topographic divide. Here AFT data range up to about 13 Ma but it is not clear if some ages few million years older are related to partially or totally reset samples. However, a similar age has been detected by ZFT on the Alpi Apuane rocks, therefore marking to the Middle Miocene the cooling at about 240°C. As time of maximum burial for the Macigno Formation is constrained at 15-20 Ma, initial exhumation of the Apuane was coeval with tectonic thickening higher in the wedge. By 5 Ma the Apuane rocks were exhumed to 70°C and 2 km. Exhumation of the Ligurian unit is poorly constrained because of the low amount of data available, mostly characterized by not reset ages.
Variation of exhumation ages across the chain results of course in a variation of exhumation processes during time. If the long-term exhumation rate seems to be of about 0.7 km/m.y., episodes of enhanced erosion rates up to 1 km/m.y. have been recognized both in the contractional pro-side of the belt and in the core of the orogen. It is noteworthy that these rates are significatively higher than the drainage basin erosion rates of 0.2-0.6 km/m.y. determined by cosmogenic isotopes (Cyr and Granger, 2008).
Central Apennines
Thermochronological dataset is here very limited as only a few AFT ages have been obtained from the Gran Sasso Range and Laga Basin (on both pre-orogenic and syn-orogenic deposits; Rusciadelli et al., 2005; Fig.11). However, more abundant is the dataset related to thermal indicators of maximum burial such as vitrinite reflectance (Fig.12), illite content in mixed layers I-S (Fig. 13) and Kübler Index (Fig. 14) (subordinately T max from pyrolisis, fluorescence colours, Thermal Alteration Index, not in the maps) that were mainly acquired on syn-orogenic siliciclastics and subordinately on pre-orogenic clayey and marly portions of pelagic basin successions (Molise, Sannio, Sabina and Umbria-Marche pelagic basins; Corrado, 1994; 1995; Corrado et al., 1998; 2003; Di Bucci et al., 1996, Rusciadelli et al., 2005; Aldega et al., 2007a). Mean vitrinite reflectance data from the Meso-Cenozoic pelagic successions (Sabina basin, Latium) at the top of the thrust stack of the Central Apennines indicate the immature stage of hydrocarbon generation (Ro% = 0.25-0.30%). Vitrinite reflectance might be suppressed by the influence of abundant amorphous matter and marine phytoplacton in palinofacies where woody particles may be less than 20%. Nevertheless reflectance data are in reasonable agreement with low T.A.I. values (ranging between 1.2 and 2.0) and bright fluorescence colours, described in details by Corrado (1994, 1995).
For the Umbria-Marche basin, the Montagna dei Fiori structure shows low thermal maturity levels (about Ro% = 0.40% for the Bonarelli level, illite contents in mixed-layers I-S between 40 and 64% and KI data ranging from 0.89 to 1.03 °∆2θ for the pre-orogenic succession). On the other hand, along the Gran Sasso range, fission tracks on three samples have been totally reset as the stratigraphic age (Late Triassic-Early Jurassic) is much older than the AFT age (27-40 Ma; Rusciadelli et al., 2005). Rusciadelli et al. (2005) try to do some thermal modelling on these ages by integration with some vitrinite reflectance data. Their results seems to indicate an exhumation phase in the last 5 m.y., confirming the geological evidence of the existence of a Middle Pliocene unconformity (Crescenti et al., 2004).
Thermal constraints for the Molise and Sannio Basins were provided only by X-ray diffraction (Corrado et al., 1998). The Upper Oligocene(?)-Lower Miocene varicoloured clays show two different types of mixed layers I-S: diagenetic smectite-rich I-S that are almost present in all samples, and detrital illite-rich I-S. The predominance of discrete smectite and small amounts of random-ordered mixed layers I-S with illite content between 10 to 50% were recorded for the Molise succession whereas smectite-rich I-S with illite layers between 20 and 40% were observed for the Sannio succession. These data indicate the early diagenetic zone and low burial termperatures according to Merriman and Frey (1999).
Syn-orogenic siliciclastics are from Latium, Abruzzi, Molise and Marche regions. They are mainly preserved at the footwall of regional thrust sheets that are progressively younger from hinterland to foreland with ages bracketing between the Upper Tortonian and the Messinian. Organic palinofacies are generally rich in woody continental particles, generally exceeding 60%, where indigenous vitrinite macerals are clearly distinguishable.
Figure 11. Distribution of thermochronological data in the Central Apennines
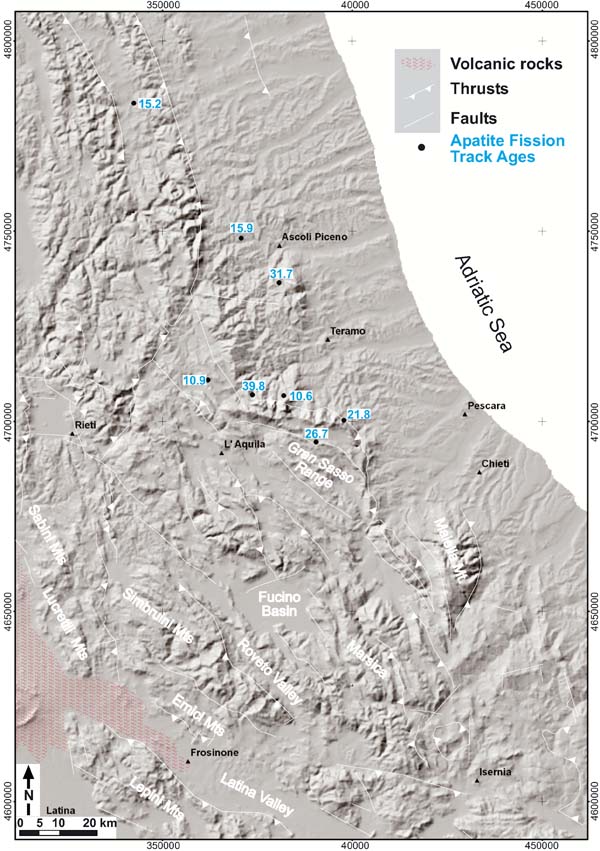
Digital Elevation Model of the Central Apennines showing the distribution of apatite fission track data. Simplified main geological features from APAT (2004) and Corrado et al. 2003. Coordinates in UTM European Datum 1950 33N.
Figure 12. Distribution of organic matter thermal maturity data in the Central Apennines
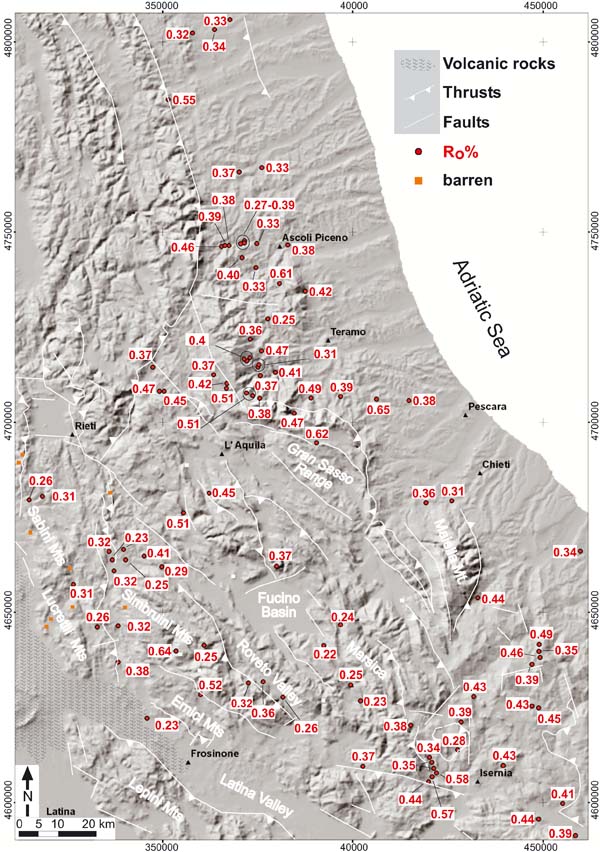
Digital Elevation Model of the Central Apennines showing the distribution of mean vitrinite reflectance data. Simplified main geological features from APAT (2004) and Corrado et al. 2003. Coordinates in UTM European Datum 1950 33N.
Figure 13. Distribution of illite content in mixed layer I-S data in the Central Apennines
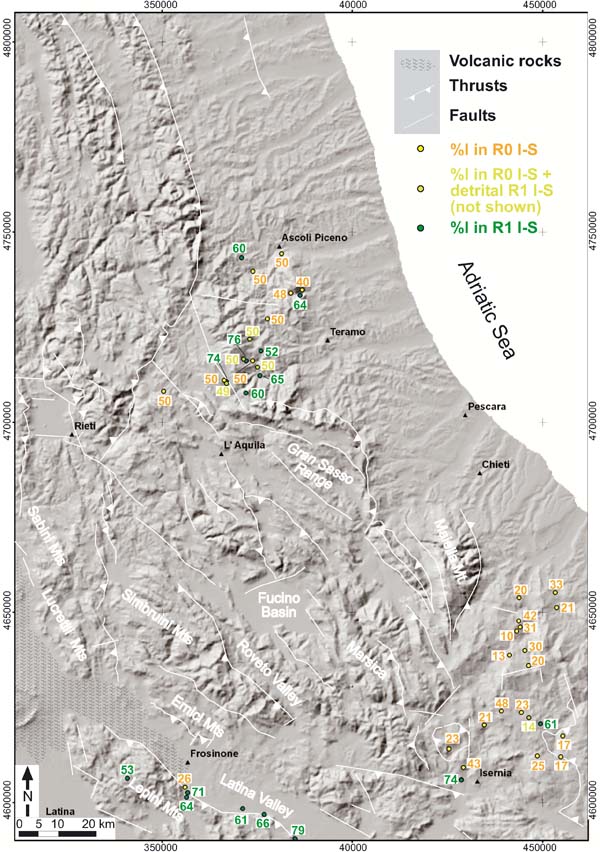
Digital Elevation Model of the Central Apennines showing the distribution of illite content in mixed layers I-S. Simplified main geological features from APAT (2004) and Corrado et al. 2003. Coordinates in UTM European Datum 1950 33N.
Figure 14. Distribution of Kübler Index data in the Central Apennines
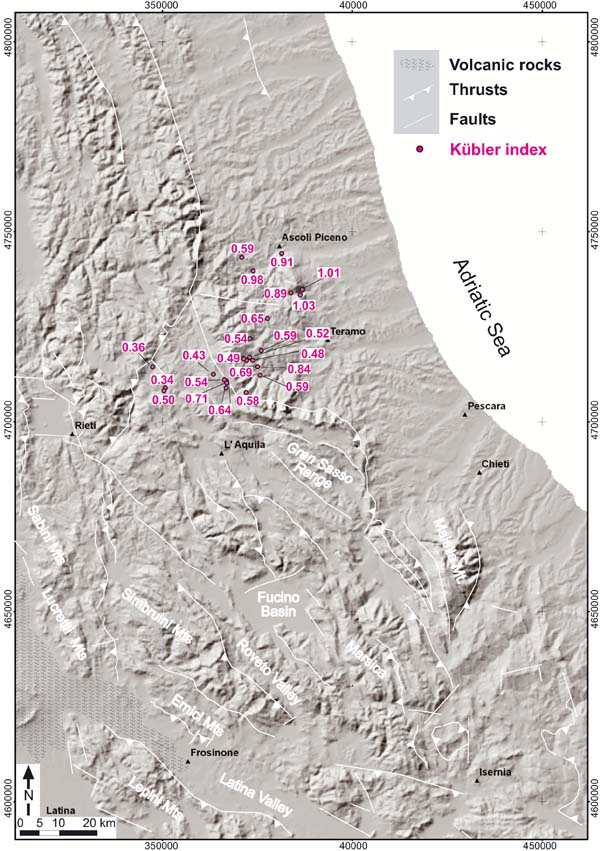
Digital Elevation Model of the Central Apennines showing the distribution of Kübler Index data. Simplified main geological features from APAT (2004) and Corrado et al. 2003. Coordinates in UTM European Datum 1950 33N.
Upper Tortonian foredeep and Lower Messinian? thrust-top basin deposits cropping out along the Latina Valley at the footwall of the Volsci Range display low levels of thermal maturity with vitrinite reflectance values ranging from 0.25 to 0.40% in the immature stage of hydrocarbon generation. Lower Messinian siliciclastics cropping out in the Roveto Valley at the footwall of the Simbruini-Ernici ridge and to the East of the Olevano-Antrodoco line at the footwall of the Sabina structures in pelagic facies, show palinofacies with vitrinite reflectance of indigenous macerals between 0.25 and 0.35%. Lower Messinian siliciclastics cropping out in the narrow valleys to the West and to the East of the Montagna Grande carbonate ridge show an extremely low thermal maturity with values ranging between about 0.2 and 0.3%. Slightly higher values (between 0.3 and 0.4%) are detected to the south of the ridge at the footwall of the Meta Mountains carbonate thrust sheet as result of tectonic loading.
Lower Messinian foredeep deposits on top of the pelagic Molise basin that extensively crop out to the East and to the North of the structures of the Latium-Abruzzi carbonate platform show slightly higher values than those to the West with about 0.6% at the base and 0.3% at the top of the siliciclastic succession. T.A.I. values are between 2.3 and 2.5.
In the Laga Basin, Messinian siliciclastics show increasing vitrinite reflectance as function of depth (from 0.31 to 0.6%) in the basin depocentre and values of 0.45-0.5% in the immediate footwall of the Gran Sasso Range and of the Sibillini thrust front. Tmax data (412-446°C) and diagenetic random-ordered and short ordered I-S with an illite content ranging from 50 to 76% mirror the same spatial distribution confirming vitrinite reflectance data (Aldega et al., 2007a). On the other hand, KI data (from 0.34 to 0.84 °∆2θ) indicate more evolved stages of thermal maturity when compared to organic and other inorganic thermal parameters. These data mainly refer to detrital 10 Å phases, either metamorphic or sedimentary, associated with the uplift of the Alpine-Apennine chain. In this case, KI do provide information on provenance and thermal conditions of the source rock and cannot be used for the reconstruction of the thermal history of the Laga Basin. The AFT dataset (Rusciadelli et al., 2005), given the “young” depositional age, indicate that burial temperatures never exceeded the PAZ range. Therefore, only detrital ages could been obtained and they can be interpreted in terms of erosion of a source rock more than bedrock exhumation.
Low maturity levels characterise the pre-orogenic successions in carbonate platform, slope and pelagic basin facies. Organic and inorganic thermal parameters suggest that both frictional heating and tectonic loading that commonly enhance thermal maturity in thrust belt systems were unimportant for the acquired levels of diagenesis of the pre-orogenic succession (Bustin, 1981; Underwood et al., 1988). In addition, sedimentary burial during subsidence and foredeep migration which is a major cause of thermal maturity increase during mountain building was also relatively ineffective in pre-orogenic facies maturation as suggested by field analysis. The low maturity of the slope (Sabini-Reatini Mts.) and carbonate platform (Simbruini-Ernici Mt.) successions strongly suggests that the present-day lack of turbiditic deposits in these areas is due to the deposition of reduced volumes of these deposits or, at least to non deposition.
For the syn-orogenic siliciclastic deposits, organic and inorganic indicators show a substantial thermal immaturity, with only few local exceptions that exceed Ro values of 0.6%, at the front of the carbonate core of the belt. These results indicate that the main cause of thermal evolution of the siliciclastic deposits is sedimentary burial. Occurrence of relevant tectonic loading (e.g., Gran Sasso area), now totally removed, or shear heating concentrated along regional strike-slip fault zones are very locally testified in the Central Apennines.
Southern Apennines
The dataset includes ~144 samples for XRD analysis, 24 samples for vitrinite reflectance and 18 for AFT ages (Figs. 15-18). XRD and AFT analyses were performed on the sedimentary cover of the ophiolite-bearing Ligurian units, on Miocene sediments on top of the Mesozoic Apennine platform (Bifurto, Monte Sierio, Castelvetere and Gorgoglione Formations), on the Lagonegro Units (Monte Facito, Calcari con Selce, Scisti Silicei, Galestri Formations) and on the Messinian transgessive cycle of the Apulian Platform. Most vitrinite reflectance data are from siliciclastic sediments and from the Monte Facito Fm. Thermal and thermochronological constraints indicate that the Southern Apennines can be divided into two distinct thermotectonic plates: an upper plate that records limited burial and only minor heating, and a lower plate that has been extensively tectonically buried and heated.
Figure 15. Distribution of illite content in mixed layer I-S data in the Southern Apennines
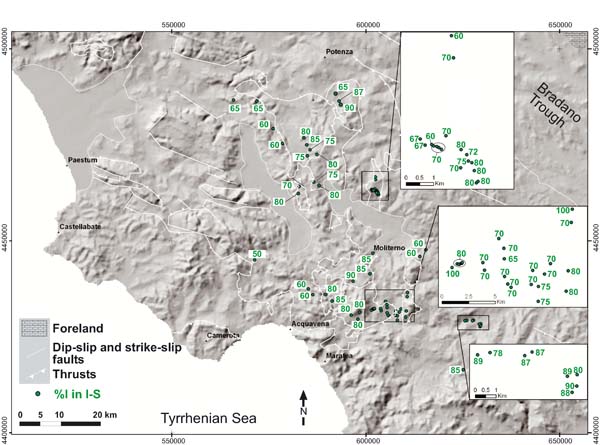
Digital Elevation Model of the Southern Apennines showing the distribution of illite content in mixed layers I-S. Simplified main geological features from APAT (2004) and Patacca and Scandone (2007). Coordinates in UTM European Datum 1950 33N.
Figure 16. Distribution of Kübler Index data in the Southern Apennines
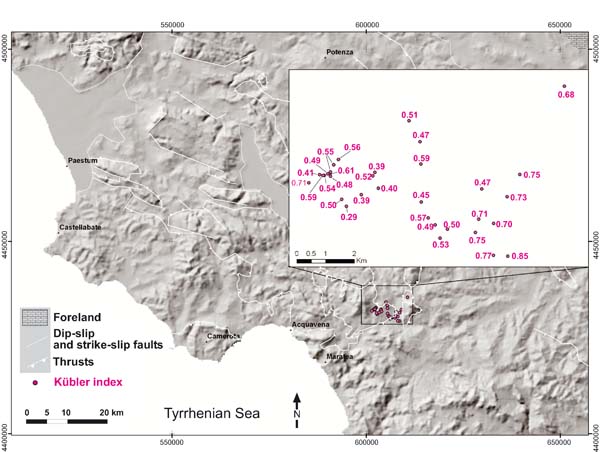
Digital Elevation Model of the Southern Apennines showing the distribution of Kübler Index data. Simplified main geological features from APAT (2004) and Patacca and Scandone (2007). Coordinates in UTM European Datum 1950 33N.
Figure 17. Distribution of organic matter thermal maturity data in the Southern Apennines
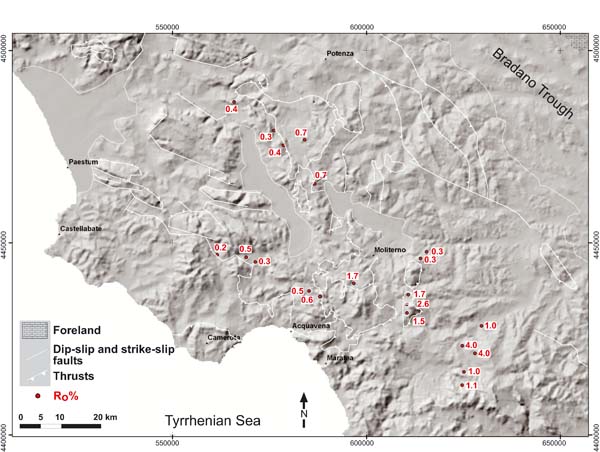
Digital Elevation Model of the Southern Apennines showing the distribution of mean vitrinite reflectance data. Simplified main geological features from APAT (2004) and Patacca and Scandone (2007). Coordinates in UTM European Datum 1950.
Figure 18. Distribution of thermochronological data in the Southern Apennines
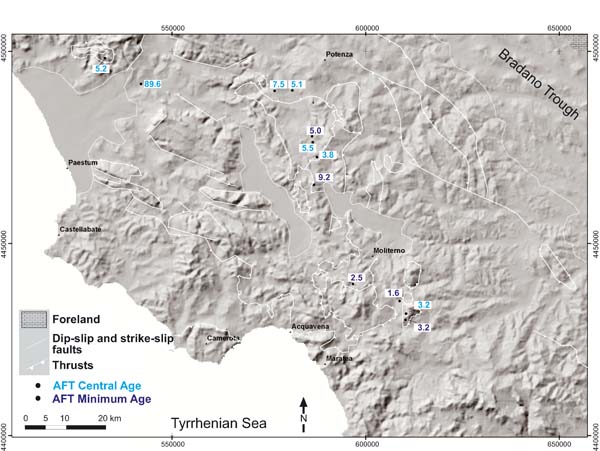
Digital Elevation Model of the Southern Apennines showing the distribution of apatite fission track data. Simplified main geological features from APAT (2004) and Patacca and Scandone (2007). Coordinates in UTM European Datum 1950.
The upper plate consists of the rocks of the Apennine Platform domain the tectonically overlying “internal” units (Ligurian and Sicilide). The former are characterized by illite contents in mixed layer I-S (50<%I in I-S<70) and Ro% values (0.25<Ro%<0.55) in the range of the early diagenetic zone and the immature to early mature stage of hydrocarbon generation and by no annealing of AFTs. Thermal modelling (Corrado et al., 2005) suggests the minor effect of burial (never exceeding 2 km) for the rocks of the Apennine Platform domain. A cooling age of 86.9 ± 11.8 Ma from a sample of the overlying Sicilide unit – which is older than the stratigraphic age – can be interpreted in terms of erosion of a source rock and is consistent with the maximum temperatures calculated for the Apenninc Platform domain. To the South, rocks from the Apennine Platform Domain (Pollino ridge) experienced higher levels of thermal maturity exceeding the PAZ range with an age of exhumation of 6.0 ± 1.1 Ma (Invernizzi et al., 2008). The Ligurian unit (Loc. San Severino Lucano village) shows an illite content in mixed layer I-S in the range of 62-89% and Ro% values of about 1.02% indicating early to late diagenetic conditions and mature stage of hydrocarbon generations with a cooling age of 11.4± 1.2 Ma (Invernizzi et al., 2008).
The lower plate includes rocks of the Lagonegro Basin, the Monte Croce, and the Apulian Platform domains. For the Lagonegro Basin successions, organic and inorganic thermal indicators show an increase of thermal maturity as a function of depth. Illite crystallinity, expressed as Kübler Index, ranges from 0.45 to 0.86 °∆2θ , illite content in mixed layer I-S from 60 to 99% and Ro% from 1.06 to 1.76% recording late diagenetic conditions and overmature hydrocarbon generation (Aldega et al., 2003; Corrado et al., 2005). KI data lower than 0.45 °∆2θ are affected by detrital K-white mica and cannot be considered for grade determinations.
For the Miocene siliciclastic deposits transgressive on the Lagonegro unit, data show a general decrease in thermal maturity between the mature and the immature stage of hydrocarbon generation from the west (constrained by mineralogical and organic matter data) to the east (constrained by organic matter data). The data from Monte Alpi, representing the unique outcrop of Apulian Platform rocks within the thrust belt consistently fall into the late diagenetic zone and late mature stage of hydrocarbon generation and indicate burial depths in excess of 5 km (Corrado et al.,, 2002; Mazzoli et al.,, 2006). Data from productive wells drilled in southern Apennines show lower thermal maturity for the Apulian Platform Domain in the range of 0.5-1.35 Ro% (Scotti, 2003). As opposed to the rocks belonging to the upper plate, all samples from the lower plate were affected, during the Neogene, by maximum temperatures higher than total annealing temperature. Therefore, ages reflect time of recent cooling through the isotherm ~110°C, effectively dating the exhumation of the previously buried successions. Thermal modelling (Aldega et al., 2003; 2005) constrained by mixed layered clay minerals and vitrinite reflectance indicate slight differences in thermal maturity along the strike of the chain related to different amounts of tectonic loads and in the timing of exhumation. Calculated maximum temperatures are between 124-142°C at the top and ~160-171°C at the base of the Lagonegro succession. Generally, AFT data indicate a late Miocene (<10 Ma) onset of exhumation of previously deeply buried rocks in the southern Apennines. In particular, a substantial decreasing of exhumation ages is shown from the North to the South of the Agri River Valley. For the Lagonegro Basin rocks, AFT ages to the North (5.1-3.8 Ma) are ~2 Ma older than those observed to the South (3.2-2.5 Ma) with important implications for oil generation (Mazzoli et al., 2008). In the northern sector, thinning of the allochthonous units have started before their emplacement onto the Apulian Platform carbonates with respect to the southern area where cooling ages display an exhumation following the deep burial of the Apulian Platform. For this reason, productive oil wells are located only to the North of the High Agri River Valley.
A possible evolutionary scenario based on these data is reported in Figure 19 (see also the section on the geological setting of the Southern Apennines).
Figure 19. Interpreted tectonic evolution of the Southern Apennines
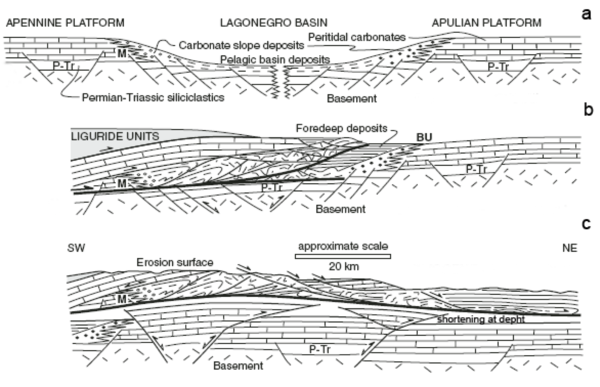
Simplified sketches showing the tectonic evolution of the Southern Apennines. Carbonate slope facies associated with the eastern margin of the Apennine Platform and western margin of the Apulian Platform are shown with white and black dots, respectively. The location of the eastern margin (M) of the Apennine Platform is held fixed through the three panels for reference. a: Mesozoic setting of the continental margin subsequently telescoped in the Southern Apennines. The Liguride oceanic domain was located to the west of the Apennine Platform. The Lagonegro Basin is shown in reduced width (note that the original width of the basin remains poorly constrained); b: “closure” of the Lagonegro Basin, initiating buttressing of the allochthonous wedge against the western inherited rifted margin of the Apulian Platform (BU); c: following emplacement of the allochthonous wedge above the westernmost portion of the Apulian Platform, gravitational readjustments dominate within the allochthonous wedge, triggering denudation and tectonic exhumation (assisted by erosion). Active shortening migrated to the underlying Apulian crust, producing basement-involved inversion at depth. After Mazzoli et al. (2008), modified.
Calabria
The dataset includes published data on low temperature chronological indicators such as apatite and zircon fission track by Thomson, (1994, 1998; Fig. 20). They provide low temperature and time constraints on the late orogenic cooling and exhumation history of the Calabrian crystalline basement rocks. The AFT ages display a relatively narrow scatter of ages between 40±5 Ma and 7±1 Ma. A general trend is observed with younger AFT ages in the lower and inner basements units, and older AFT in the tectonically higher and external units. The ZFT ages show a much wider age scatter between 299±21 Ma and 14±1 Ma. Fission track data reveal that both Alpine and high-grade Hercynian metamorphic rocks experienced a phase of increased cooling rates between 35 Ma and 15 Ma. Cooling has ceased in the majority of the basement rocks by 11 Ma which is the maximum age of the overlying sedimentary rocks throughout most of the Calabrian Arc (Van Dijk and Okkes, 1991). The period of increased cooling during the Oligocene-Miocene was interpreted as the result of two coeval exhumational processes: extensional tectonism (Platt and Compagnoni, 1990) and erosion (Bonardi et al., 1980) which affect the late tectonic evolution of the Calabrian Arc.
Figure 20. Distribution of thermochronological data in Calabria
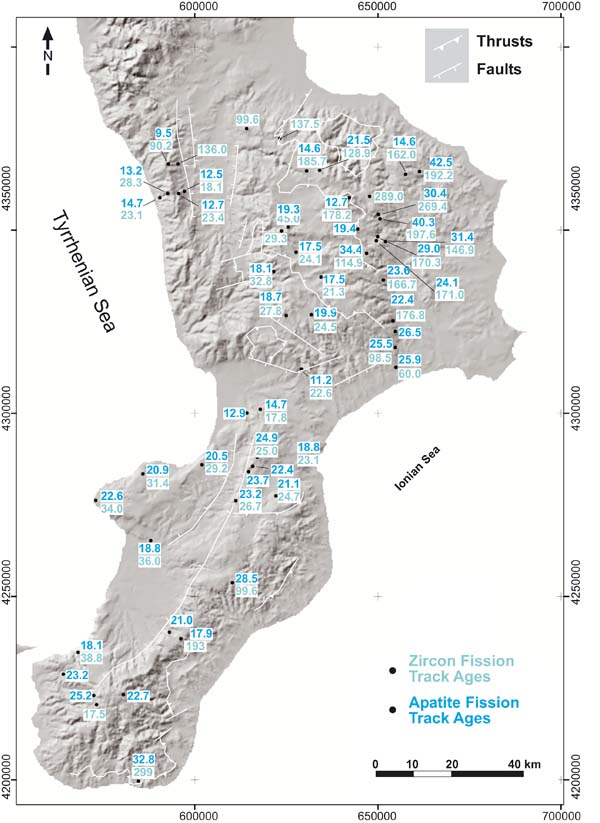
Digital Elevation Model of Calabria showing the distribution of apatite and zircon fission track data. Simplified main geological features from APAT (2004). Coordinates in UTM European Datum 1950 33N.
Thermal constraints on the sedimentary units of the Calabrian block are lacking. Available mineralogical data (%I in I-S and KI) are from Perri et al. (2008) who constrained the burial evolution of continental redbed conglomerates, sandstones, shallow-marine carbonates and turbidites of the Longobucco Group sequence (Hettangian-Toarcian) in the northeastern margin of the Sila Mountains. They observed low expandable mixed layers I-S and KI values between 0.50 and 0.86 °∆2θ estimating maximum temperatures ranging from 100 to 160°C and a lithostatic/tectonic loading in excess of 4km.
Eastern Sicily
The dataset includes 330 data. They correspond to illite content in mixed layer I-S determinations (190), vitrinite reflectance measurements (95) and AFT dating (45) (Figs. 21-23). Mostly the north-eastern sector of Sicily has been investigated to detect levels of thermal maturity and exhumation ages of the Apenninic-Maghrebian orogen. Vitrinite reflectance data are from siliciclastic sediments of the Sicilide accretionary wedge, the overlying thrust-top and foredeep deposits and from the Stilo-Capo d’Orlando Formation. XRD analysis have been performed on the different structural units constituting the accretionary wedge and related thrust-top deposits and on Africa-passive margin units. AFT data mainly refer to crystalline and metamorphic units of the Peloritani Mts and to the Sicilide accretionary wedge. The integration of organic and inorganic thermal and thermochronological indicators allowed us to distinguish three main areas at different structural level which experienced variable tectonic loads and exhumed in different times: the Sicilide accretionary wedge, the Africa-passive margin units and the thrust-top and foredeep deposits.
Figure 21. Distribution of organic matter thermal maturity data in the Eastern Sicily
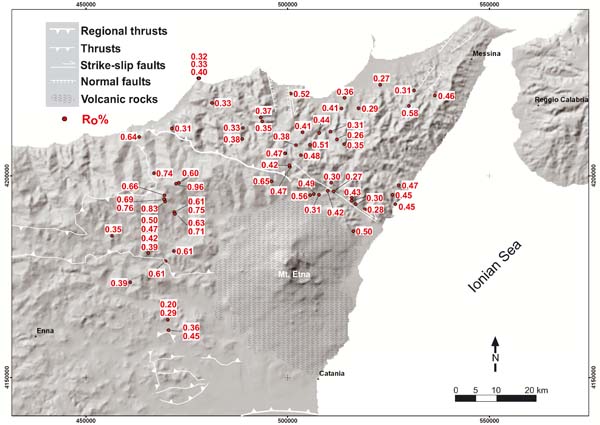
Digital Elevation Model of the Eastern Sicily showing the distribution of mean vitrinite reflectance data. Simplified main geological features from APAT (2004), Carbone et al. (1990) and Lentini et al. (2000). Coordinates in UTM European Datum 1950.
Figure 22. Distribution of thermochronological data in the Eastern Sicily
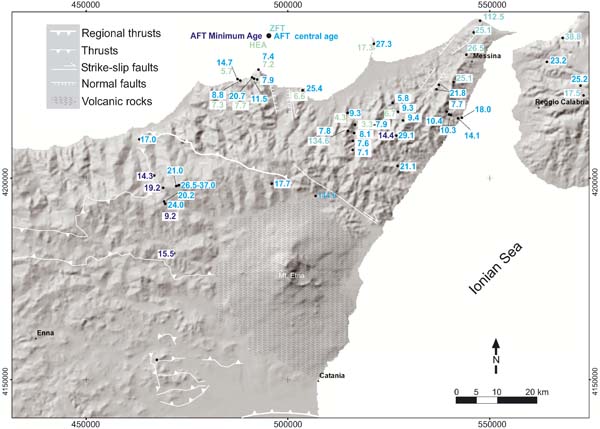
Digital Elevation Model of the Eastern Sicily showing the distribution of apatite fission track data. Simplified main geological features from APAT (2004), Carbone et al. (1990) and Lentini et al. (2000). Coordinates in UTM European Datum 1950.
Figure 23. Distribution of illite content in mixed layers I-S data in the Eastern Sicily
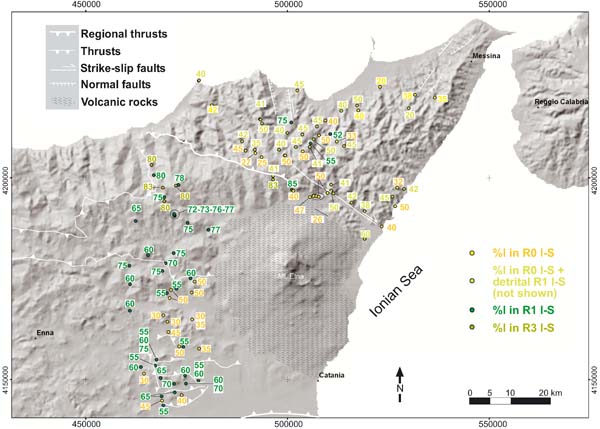
Digital Elevation Model of the Eastern Sicily showing the distribution of illite content in mixed layers I-S discussed in the text. Simplified main geological features from APAT (2004), Carbone et al. (1990) and Lentini et al. (2000). Coordinates in UTM European Datum 1950 33N.
Figure 24. Evolution of burial-exhumation of the Sicilide accretionary wedge in Eastern Sicily
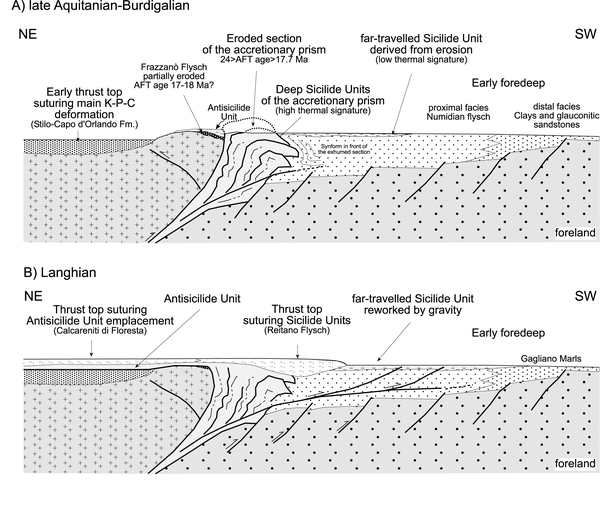
Schematic evolution of burial-exhumation of the accretionary wedge made up of Sicilide Units of the Apenninic-Maghrebian orogen in Eastern Sicily in Aquitatian-Langhian times after Corrado et al. (2009, modified). Not to scale.
In particular, within the Sicilide accretionary wedge, sectors with different thermal signature and evolution can be further identified. A warmer part of the wedge made up of the Mount Soro and Troina units and two colder rims constituted by the far-travelled Sicilide and Antisicilide units. The former are characterized by the highest values of vitrinite reflectance (0.6-0.96%) and illite content in mixed layer I-S (60-85%) corresponding to the first stages of the late diagenetic zone and the catagenesis of hydrocarbon generation (Aldega et al., 2007b; Corrado et al., 2009). This maturity level was acquired before the deposition of the unconformable thrust-top deposits of the Reitano Flysch that show low maturity levels (Ro%<0.5%) and is due to the tectonic load provided by the remains of the previously exhumed Peloritani Mts. This jump in thermal maturity suggests that Mt. Soro and Troina units’ thermal evolution was achieved before Burdigalian times. AFT data, together with the paleotemperature estimates from vitrinite-reflectance data and clay mineral-based geothermometers, indicate that fission tracks were partially to totally annealed during wedge accretion and that the subsequent exhumation occurred mainly in Burdigalian times (17.7-20.2 Ma; Corrado et al., 2009). The "far-travelled" and Antisicilide units are characterized by low Ro% values (0.41-0.56% for the Antisicilide units), illite contents in mixed layer I-S between 25 and 50% and by no annealing of fission track indicating low levels of thermal maturity in early diagenetic conditions. A cooling age of 144 ± 51 Ma for a sample from the Antisicilide Unit, older than the stratigraphic age confirms low temperatures and provides information on the cooling history of source rocks rather than on the exhumation of the sediment. This data distribution suggests differences in the Cenozoic tectonics of the Sicilide accretionary wedge (Fig. 24). The Mount Soro and Troina units were involved into trench development before early Miocene times and acquired a late diagenetic signature due to thrust-stacking at deep levels whereas the far-traveled Sicilide and Antisicilide units kept their early diagenetic signature at shallower or outer structural levels of the accretionary wedge and were remobilized since late Aquitanian-Burdigalian times by gravity-driven processes (Corrado et al., 2009).
Figure 25. Evolution of burial-exhumation of the Peloritani Mts. since middle Miocene
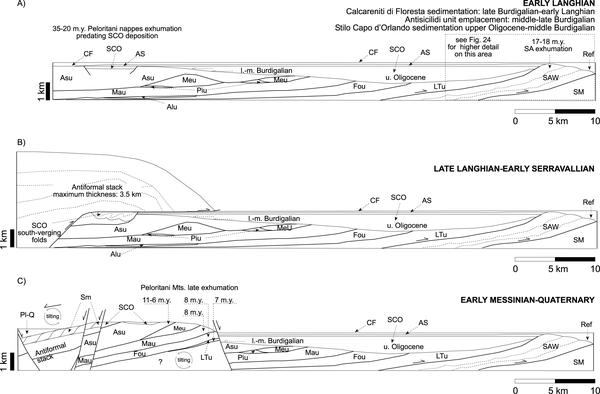
Schematic evolution of burial-exhumation of the Peloritani Mts. since middle Miocene. a) early Langhian; b) late Langhian-(?)early Serravallian; c) early Messinian-Quaternary. Acronyms - Pl-Q: Pliocene-Quaternary deposits; Ref: Reitano Flysch; SAW: Sicilide Accretionary Wedge; Sm: Serravallian-Messinian siliciclastic deposits; AS: Antisicilide unit; CF: Calcareniti di Floresta; SCO: Stilo-Capo d’Orlando Fm.; Internal Zone (nappes from top to base) - Asu: Apromonte unit; Meu: Mela unit; Piu: Piraino unit; Mau: Mandanici unit; Alu: Alì-Montagnareale unit, Fou: Fondachelli unit; LTu: Longi-Taormina unit. External Zone - SM: Sicilian Maghrebids. After Aldega et al. (in press, modified).
For the Africa passive margin units in the Mt. Judica area, thermal constraints from mixed layered clay minerals indicate a thermal evolution in the first stages of the late diagenetic zone with increasing illite layers in I-S as a function of depth from 55 to 75%. This trend is observed in each thrust sheet of the Mt. Judica succession suggesting that internal stacking did not overprint the acquired thermal maturity. Thermal modelling suggests the effect of tectonic burial (~3 km), nowadays eroded, as the main factor for the acquired levels of diagenesis since late Miocene-early Pliocene times. The range of maximum temperatures experienced by the Africa passive margin units (Monte Judica succession) can be constrained between 100 (top) and 125°C (bottom).
Syn-orogenic sediments in early and late thrust-top settings (Reitano Flysch; Pliocene clays and Terravecchia Formation; Stilo-Capo d’Orlando Formation) show the lowest Ro% values (0.20-0.48%) and illite contents in mixed layer I-S (20-50%) consistent with the early diagenetic zone and in the immature stage of hydrocarbon generation. Sedimentary burial is considered to be the main factor responsible for the acquired thermal maturity. Only a small area exposed in the northern sector of the Peloritani Mts. display higher Ro% values than those identified in the depocentre of the Stilo-Capo d’Orlando Formation. These values (0.46-0.58%) are too high considering the Stilo-Capo d’Orlando thickness in that sector and they suggest greater thermal imprint and tectonic signature. This interpretation is strongly supported by AFT analysis performed on the crystalline substratum of the basin which indicates, for an intermediate portion of the Peloritani Mts., temperatures of 80-120°C partially to totally annealing the fission tracks after the Stilo-Capo d’Orlando sedimentation (Balestrieri et al., 2008). Young AFT ages (<15 Ma) and thermal indicators constrain a thermal anomaly in the north-eastern area of the Peloritani Mts. characterized by short duration and a localized area of influence that is the result of compressive reactivation at the rear of the chain with emplacement of an out-of-sequence south-verging thrust stack (Aldega et al., in press; Fig. 25). The main exhumation phase of the Peloritani Mts. is ascribed between 35 Ma and 20 Ma with significant erosion and marked by the sedimentary record of the Stilo-Capo d’Orlando Fm in Oligocene-Miocene times.