Discussion: towards a comprehensive picture of the Central Mediterranean history
The experimental data summarized in this paper suggest several implications for the tectonic history of the Central Mediterranean subduction zone (CMSz). It is tempting to merge them into a three-dimensional evolutionary model that will offer a “modeling” picture of the CMSz. For this purpose, five key moments in the evolution of the CMSz will be dynamically explained based on our previous ad-hoc experimental results and combined with some of the natural observables (tectonic data and reconstructions are extensively described in Faccenna et al. 1999, Faccenna et al. 2001a, Faccenna et al. 2001b, Faccenna et al. 2004, Faccenna et al. 2005, Faccenna et al. 2007). To qualitatively visualize the proposed evolutionary scenario, we additionally present a novel regional model that merges the distinct modeling components described in the previous section. In particular, this gravity-driven model evolves in a restricted mantle convection (i.e., impermeable upper-lower mantle discontinuity), and it is characterized by an initial laterally heterogeneous (i.e., non-cylindrical) geometry resembling the Mesozoic paleogeographic setting generally agreed upon for the Central Mediterranean (Figure 11 a,d), with a small trapezoidal oceanic basin trapped between continental shoulders (Le Pichon 1982, Malinverno & Ryan 1986).
Figure 11. Evolution of a regional model characterized by a laterally heterogeneous geometry resembling the CMSz Mesozoic paleogeographic setting.
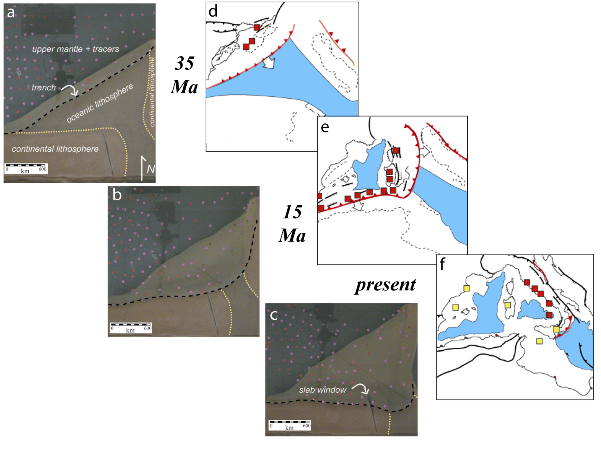
Panels a-c: Top views of three stages of evolution of a regional model characterized by an initial laterally heterogeneous geometry resembling the Mesozoic paleogeographic setting generally agreed upon for the Central Mediterranean. Pinkish and brownish silly putties are representative of continental and oceanic lithosphere, respectively. The lithosphere is locally pre-cut as analog of the weak region enhancing the formation of the slab window. Panels d-f: Tectonic reconstruction of the evolution of subduction and back-arc extension in the central Mediterranean (from Faccenna et al. 2004, 2007). Three crucial phases are shown: 35 Ma, 15 Ma, present. Red and yellow squares indicate subduction-related and anorogenic volcanism, respectively.
Phase I (50?- 35 Ma): SUBDUCTION INITIATION
The first signature of subduction (not modeled in the regional experiment but extensively described above) is experimentally marked by a rapid and localized uplifting of the continental plate. The natural prototype is represented by large-scale disconformities and a sedimentary hiatus sandwiched within passive margin sequences (Cohen 1982), and it is accompanied by flysch deposition and high pressure-low temperature metamorphisms, as extensively recognized in the Central Mediterranean during the early Paleocene (e.g., Jolivet et al. 1998, Rossetti et al. 2004, Vignaroli et al. 2008). The age of initiation of the westward dipping subduction is difficult to establish in the Central Mediterranean and several contrasting models have been proposed. Several models, in fact, proposed that the present-day westward subduction initiated after the break-off of a previous eastward dipping subduction. The timing of this event has been put at the Cretaceous–Tertiary boundary (65 Ma; Dercourt et al. 1986), the Palaeocene (50 Ma; Boccaletti et al. 1971) or the Oligocene (30 Ma; Doglioni et al. 1997). Here, we will focus only the process concerning the present-day westward subduction history.
In our models, the lithospheric subduction can be enhanced by either the slow plate convergence or the lateral pressure produced by the collapse of the continental margin. Both mechanisms are present in the Central Mediterranean (e.g., Le Pichon 1982, Dewey et al. 1989), resulting in the critical amount of gravitationally unstable lithosphere that is dynamically necessary to overcome the resisting forces and, in turn, to actively start the subduction process. Paleo-reconstructions (Beccaluva et al. 1989, Faccenna et al. 2001b) support this interpretation and indicate that 300-400 km of cold lithosphere subducted with a rather shallow dip in this period, reaching a depth of 150 km. The slab deepening allowed the development of arc volcanism, as recognized in the Sardinia-Provençal regions, with products dated up to 38 Ma (Lustrino et al. 2009). Considering the few mm/yr (<1 cm/yr for the normal component of convergence), this amount of subduction should be achieved in at least 10 Ma. The north-westward subduction should then started at least 45 Ma, more probably around 50 Ma (Lustrino et al. 2009). In agreement with this reconstruction, the age of HP-LT metamorphism in Calabria spans over an interval of age ranging between 45-50 Ma to 30 Ma (Rossetti et al. 2004).
After the transition from a Rayleigh-Taylor instability to a self-sustained subduction, the NW-dipping CMSz extended for more than 1500 km from southern Iberia to the Ligurian region, consuming the land-locked Jurassic oceanic basin (Le Pichon et al. 1988). This geometry was used in our regional experimental model (Figure 11a-d).
Phase II (30 - 16 Ma): SUBDUCTION INTO THE UPPER MANTLE
Our modeling results predicted that just after the initiation of subduction, the rollback exponentially increased during the slab’s movement into the upper mantle because of the progressively higher slab-pull driving action. This mechanism likely happened in the CMSz, enhanced by a slightly decreased African rate of motion (Jolivet & Faccenna 2000). An additional minor contribution could have been offered from the peculiar paleogeographic setting of the area, allowing for the subduction of a gradually narrower slab (Figure 11a). As we experimentally showed, trench velocity was inversely related to the slab width (see also Morra et al. 2006 and Schellart et al. 2007). Tectonic reconstructions support the hypothesis of an exponential increase in the rollback velocity for the CMSz. We propose that the maximum rates were reached when the slab arrived at the southern Sardinia margin (Faccenna et al. 1999 The rapid retreat of the slab favors the opening of back-arc basins (Uyeda & Kanamori 1979, Dewey 1980), and is a mechanism commonly proposed to explain the origin of the Central Mediterranean back-arc basins (e.g., Malinverno & Ryan 1986; Royden 1993; Faccenna et al. 2001b). A first episode of extension, which led to the formation of the Liguro-Provençal basin (Figure 1), occurred from 30-16 Ma and was accompanied by a 25-30° counter-clockwise rotation of the Corsica-Sardinia-Calabria block (van der Voo 1993).
In this phase, the continental lithosphere was localized at the shoulders of the subducting oceanic basin arriving at the trench (Figure 11; the Apenninic and the Maghrebian foreland thrust belts; e.g., Dercourt et al. 1986). Experimental data illustrated how, under these conditions, part of the buoyant lithosphere sank in the upper mantle that was being pulled at depth by the lower oceanic (i.e., heavier) part of the slab. This process experimentally produced the slowdown and verticalization of the slab (Figure 10c-d). We can infer that a similar mechanism occurred in the CMSz after the scraping of the crustal layers and their delamination from the denser, deeper mantle material (Serri et al. 1993, Jolivet 1999, Calvert et al. 2000). This speculation is supported by a detailed tectonic reconstruction made along an E-W cross-section from the Apennines to the Gulf of Lyon, recognizing that the subduction velocity progressively decreased in time until now (Faccenna et al., 2001b). An additional constraint is offered by the seismic signature of the current northern Apenninic slab, which illustrates an activity limited to shallow depths (90 km, (Selvaggi & Amato 1992) and an almost continuous/vertical velocity anomaly dipping toward the W-SW (e.g. Spakman et al. 1993, Lucente et al. 1999, Piromallo & Morelli 2003).
The laterally heterogeneous setting is likely the main origin of the Calabrian orocline and allowed for differential horizontal movements of neighborhood lithospheric blocks (see Cifelli and Mattei in this volume for further information on the argument). In our view, it is interesting to highlight how the first smooth experimental arcs were formed in front of the oceanic seaway at the beginning of this phase (Figure 11). These data are in agreement with Gattacceca & Speranza (2002) who found that a significant counter-clockwise rotation of the Apennines occurred just after the initial opening of the Liguro-Provençal basin. However, a more significant counter-clockwise rotation of the Apennines has been recognized only from the early Pleistocene (Sagnotti 1992, Scheepers et al. 1993) , when, under our interpretation (and qualitatively recorded by our regional model; Figure 11), the northern block was considerably slowed by continental subduction differentiated from the southern oceanic fast-retreating lithosphere.
Phase III (16 - 10 Ma): SLAB INTERACTION WITH THE 660-KM DISCONTINUITY
Our models showed that once the slab started to interact with the 660-km discontinuity, the subduction slowed for a significant amount of time. However, this happened only when the viscosity contrast was larger than 10 and imposed between the upper and the lower mantle layers (see Funiciello et al., 2003 for further details on vertical unconstrained systems), inhibiting the direct penetration of the descending slab. The restricted mantle convection could be a key reason for the peculiar behavior recorded in the CMSz between 16 and 10 Ma. In this interval, it has been recognized that no noteworthy changes took place on the surface (see Faccenna et al. 2001b and references therein) independently of possible kinematic variations produced by the African plate, which gradually continued its slow convergence (Figure 2). The hypothesis is further strengthened by the following: a) tomographic images showing that the deepest portion of the slab is currently stagnant on the 660-km discontinuity (e.g., Spakman et al. 1993, Lucente et al. 1999, Piromallo & Morelli 2003); b) paleo-reconstructions, which start from the present-day configuration and adopt a balancing technique constrained by natural observables, recognized that the slab should have approached the upper/lower mantle limit during this interval (Faccenna et al. 2001a). As an additional natural consequence of a nearly fixed subduction configuration, the Liguro-Provencal back-arc opening stopped (Bache et al., 2009), as did the associated rotation of the Sardinia-Corsica block (van der Voo 1993).
Phase IV (10 Ma - Today): SLAB NARROWING
We experimentally observed how a slab distributed along the upper mantle depth creates an important physical barrier to the mantle, influencing its three-dimensional circulation. In particular, we described how the slowed-down process can restart only after the mantle circulation is deeply re-organized, assuming a predominant toroidal form (Figure 6,7). A similar behavior should have characterized the CMSz in the Tortonian. The combination of the continental African (Tell and Apulia; e.g., Casero & Roure 1994, Chiarabba et al. 2008) lithospheric subduction at the shoulders of the system and a vigorous lateral flow enhanced the progressively lateral fragmentation of the Calabrian slab (Figure 11). Once broken, this small slab was free to retreat towards the SW independently of the rest of the eastern, larger, Algerian slab.
In our cylindrical models, the subduction behavior was steady-state from this time, as justified by the equilibrium between active and resisting forces. Moreover, the locus of the back-arc extension jumped trenchward following the current steeper configuration. The proposed experimental scenario partially resembles what was geologically recorded in the CMSz since the Tortonian. After the period of inactivity, a second extensional episode formed the Tyrrhenian basin, and a new spreading center jumped towards the trench.
However, the natural context has been complicated by the presence of short-lived episodes of oceanic accretion, which separated small back-arc basins during the early Pliocene (~ 4.3-2.6 Ma, Vavilov basin; Kastens & Mascle 1990) and the early Pleistocene (2-1- Ma, Marsili basin; Patacca et al. 1990, Nicolosi et al. 2006). Despite the inherent oversimplifications of our experimental set-up, these models offer useful suggestions that support the hypothesis that the episodicity of the Tyrrhenian back-arc spreading is related to the progressive narrowing of the Calabrian slab (Wortel & Spakman 2000, Faccenna et al. 2005, Chiarabba et al. 2008). In Figure 9, we show that an abrupt decrease of subducting plate width results in a pulse of acceleration of the trench retreat velocity, as the balance between driving and resisting forces acting on the slab is temporarily modified. A similar mechanism likely worked in nature, where the enlargement of the slab window operated discretely. In the late Messinian, the slab window resulting from the propagation of slab tearing shifted eastward about 300 km from the active portion of the foreland-thrust front (Argnani et al. 1987, Casero & Roure 1994). A further slab window enlargement of about 400 km was finally achieved at about 1-0.8 Ma ago (Faccenna et al. 2005). The N-S trending eastern plate boundary also experienced lateral propagation of slab detachment (Govers & Wortel 2005). Slab break-off initiated in the northern part of the subduction zone at 8-9 Ma as a result of the subduction of the continental lithosphere and then migrated in a southeastern direction (Wortel & Spakman 2000).
Finally, we have experimentally shown how a narrow slab experiences a vigorous toroidal mantle circulation. Under this condition, the sub-slab material turns around the slab edges and easily enters the back-arc domain because of the following: a) smaller lateral pathway; and b) faster mantle motion (i.e., the velocity of the mantle corresponds to the trench velocity, which is inversely related to the slab width). The main natural evidence of this behavior is represented by the SKS splitting pattern of the CMSz (Civiello & Margheriti 2004, Baccheschi et al. 2007, 2008). The NS-oriented fast directions, φ, in western Sicily have a high angle with respect to the thrust front and could be related to the lateral return flow around the western slab edge (e.g., Faccenna et al. 2005). The signal recorded at the eastern side of the Calabrian slab, on the contrary, follows the arcuate shape of the subducting lithosphere, which is analogous to the general trend of φ recorded along the belt. This pattern does not exclude the presence of a slab window below the southern Apennine, as detected by tomography (e.g., Faccenna et al. 2007)), because the anisotropic signal could be dominated by lithospheric deformation (Jolivet et al. 2009). In fact, the experimental test matched the orientation of the splitting anisotropy, justifying the orientation of the NS trend in western Sicily, but further tests are necessary to provide a quantitative comparison. The geochemical signature also agrees with this model (Trua et al. 2003) , indicating that the back-arc mantle has been progressively contaminated by inflow of fertile (African) mantle material. In the southern Tyrrhenian region, magmatism has preserved the imprinting of a mantle metasomatized by the subduction of crustal material before the lateral rupture of the slab occurred (El Bakkali et al. 1998, Coulon et al. 2002).After a while, the amount of mantle material flowing inside the back-arc region increased, changing the composition of the erupted magmas from predominantly calc-alkaline to alkaline (Faccenna et al. 2005). At the site of the initial break (northern Tunisia), the calc-alkaline rhyolite suite of Nefza and Galite islands (14-8 Ma, Savelli 2002) was replaced in the Upper Miocene (about 8-6 Ma) with the (Na)-alkaline basalt of Nefza and Mogodos. The enlargement of the slab window occurred in the late Messinian, further feeding the system. Consequently, the alkaline Aceste seamount was emplaced just west of the calk-alkaline Anchise seamount during the Early Pliocene (Beccaluva et al. 1994), while a new phase of anorogenic alkaline magmatism was also registered in Sardinia (Savelli 2002).
Phase V (Tomorrow): TOWARD A PASSIVE DEBLOBBING?
Despite the inherent oversimplifications of our regional model, it could be used to speculate about the future scenario that will likely characterize the CMSz in geological times. We qualitatively find that the reduction of the active portion of the slab beyond a critical dimension (<3.5 cm, corresponding to about 200 km) finally produces the locking of the trench. The slab becomes too small to actively subduct and passively steepens under its own weight. After the slab attains a vertical geometry, it evolves into a viscous deblobbing, facilitated by the adopted rheology of the system.
A similar evolution could be starting in the CMSz, as currently demonstrated by the lack of evidence for active spreading, when compared with the Neogene-Quaternary estimates of 50-70 mm/yr for the back-arc rate (D’Agostino & Selvaggi 2004). However, the low Africa-Eurasia convergence rates (not simulated in our model) provide an additional component to promote subduction, even under these conditions, and they only translate into subduction velocities.