What we know
Plate kinematics
Africa started to move against the southern European paleo-continental margin during Early Cretaceous, when the first rifting stages in southern Pangea gave room to oceanization processes in the southern Atlantic Ocean (e.g., Dercourt et al., 1986; Eagles, 2007; and references therein). The Tethyan Ocean, located between European and Africa continental plates, started to be consumed with different subduction polarities and complex geometries, also due to the presence of still poorly constrained and debated continental micro-plates in between (Iberia, Adria, AlKaPeCa, Briançonnais, Sesia-Lanzo, and so on). The vectors of the first stage Africa-Europe relative motions are not known in detail and different models have been proposed in the literature. During the Paleogene, a N-NE motion of Africa against Europe (assumed to be fixed) was proposed (Dewey et al., 1989; Mazzoli and Helman, 1994; Rosenbaum et al., 2002a).
Figure 1. Topography-bathymetry and geological map of the Mediterranean area.
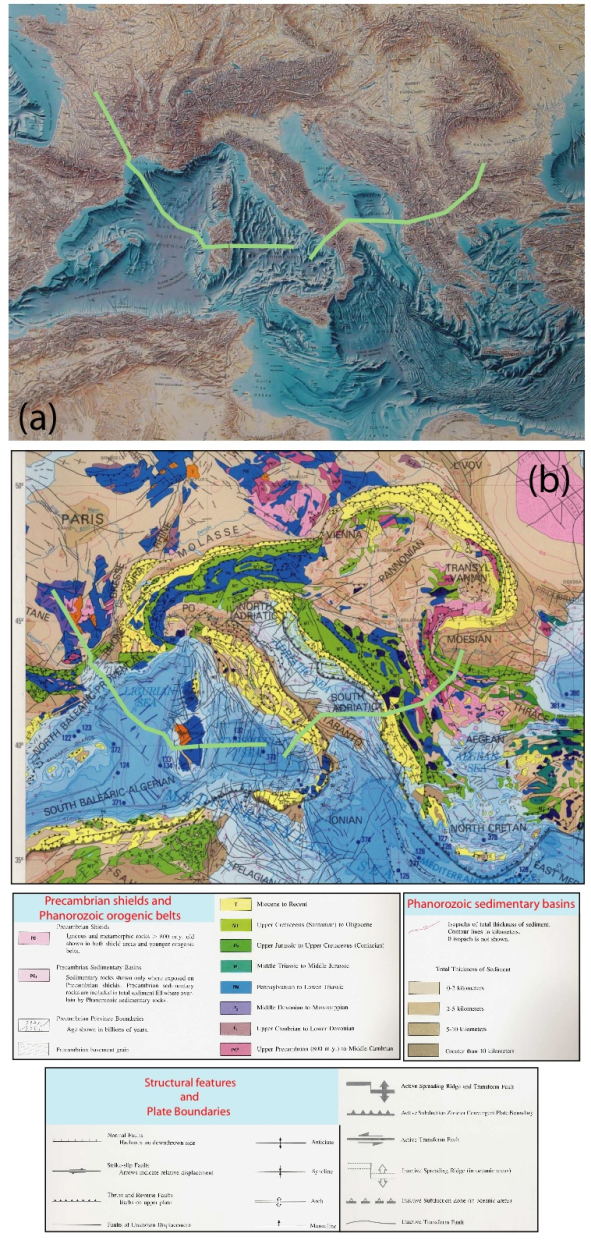
(a) Topography-bathymetry (after Tanguy de Rémur) and (b) geological map (After EXXON Prooduction Research Company, 1994) of the Mediterranean area. The trace of the cross section of Fig. 2 is shown.
A major change happened during the Eocene, when convergence rates reduced to <1 cm/yr and the convergence direction changed to N-NW (e.g., Rosenbaum et al., 2002a, 2002b, and references therein). Recent space geodesy data confirm this main frame, where Africa has about 4-5 mm/yr of N-S component of convergence relative to Europe (e.g., Devoti et al., 2008). To complicate the scenario, geodynamic evidence suggests that Africa and the intervening microplates have been affected by simultaneous rotations (i.e., rotation and sub-rotations, Cuffaro et al., 2008) since at least the Early Oligocene. This represents a key kinematic constraint to understand the evolution of tectonic settings in the Mediterranean Area.
Figure 2. Lithospheric scale cross-section through the Central Mediterranean
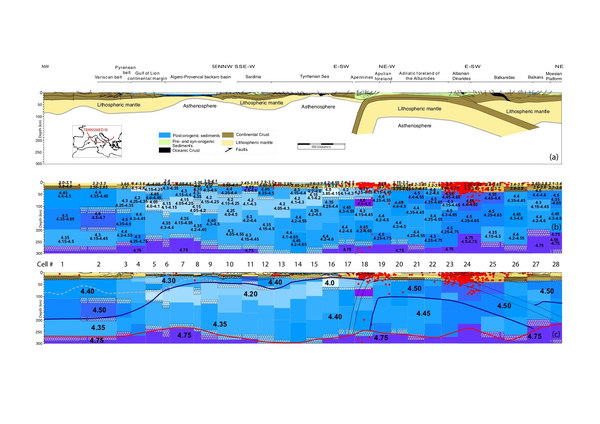
a) Lithospheric scale cross-section through the Central Mediterranean (simplified and redrawn from the TRANSMED III geotraverse; Carminati et al., 2004). (b) Lithosphere-asthenosphere system along the trace of the TRANSMED III geotraverse: the tomographic cross section was obtained from selected solutions and related seismicity (body waves magnitude greater or equal to 3.0). The VS and its range of variability in km/s are printed on each layer. Numbers in italic denote the velocities in the crustal layers. The hypocenters are denoted by dots. (c) Overlap between the TRANSMED III regional cross-sections and the Vs tomography (after Panza et al., 2007).
The nature, size and kinematics of the Adriatic area is also debated. Paleomagnetism, propagation of transverse waves refracted below the Mohorovicic discontinuity, paleoclimatology and palinology suggest that Adria belongs structurally and kinematically to Africa (e.g., Mantovani et al., 1990, Channell, 1996; Muttoni et al., 1996; Mele, 2001), whereas historical seismicity, geodetic and seismic evidence suggest that Adria is now an independent microplate within the Africa-Eurasia plate boundary zone (Anderson and Jackson, 1987; Nocquet and Calais, 2003). In the following, Adria is considered, as a first approximation, separated from Africa during the time of opening of the Ionian oceanic basin, whose age is still poorly constrained. (Fig. 3; Catalano et al., 2001). Nevertheless, for sake of simplicity and lack of better constraints, the Adriatic plate is assumed to have moved coherently with Africa in the movie attached to this article.
Figure 3. Simplified structural map of Italy (modified from Consiglio Nazionale delle Ricerche, 1992; Scrocca, 2006).
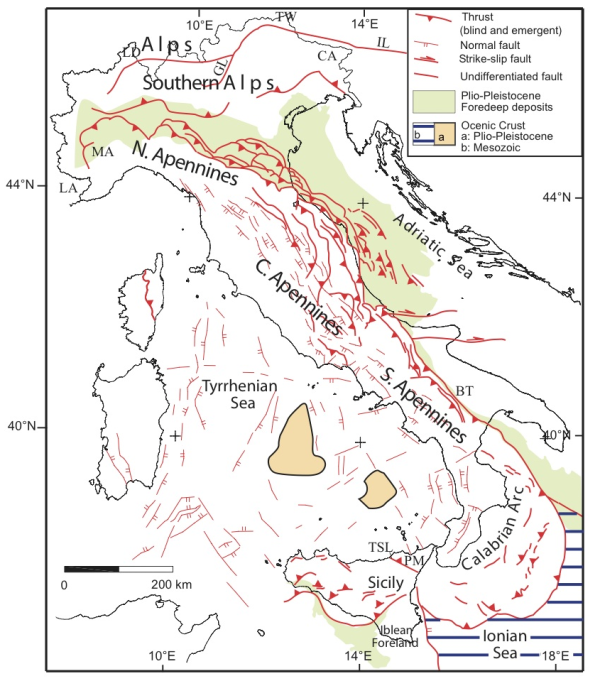
LA: Ligurian Alps; MA: Monferrato Apennines; IL: Insubric Line; CA: Carnian Alps; GL: Giudicarie Line; LD: Lepontine dome; TW: Tauern Window; PM: Peloritani Mountains; BT: Bradanic trough; TSL: Taormina-Sangineto Line.
Tectonic and magmatic constraints
Italy is a tectonically (Fig. 3) and magmatologically active region, as testified by the distribution of earthquakes (Fig. 4) and igneous activity. Earthquakes show prevalent compressional focal mechanisms at the fronts of Alps and Apennines and extensional mechanisms along the Apennines backbone. This picture is confirmed by in-situ stress measurements (e.g., borehole breakouts; Montone et al., 2004). As it will be discussed later, Alps and Apennines show markedly different characters (Carminati et al., 2004a). The Apennines tectonics partly overlap the Alpine structures in the Ligurian Alps-Monferrato Apennines (Fig. 3; Laubscher, 1971; Schumacher and Laubscher, 1996; Molli et al., this volume). The different evolution of the Alpine and Apenninic belts is mirrored by the different geometry of the respective foredeep basins (shallow in the Alps and deep in the Apennines), strongly controlled by the dip of the foreland monocline (shallow in the Alps and steeper in the Apennines; Mariotti and Doglioni, 2000). The geometry of the compressional structures is mainly controlled by active geodynamic processes and paleogeography (distribution of structural highs and lows, inherited from the Mesozoic rifting). Salients (where the tectonic front is more advanced) and steeply dipping regional monoclines (e.g., the top of the basement) are normally associated with Mesozoic basins, characterized by thick successions and deep basal decollements. Recesses (where the front is less advanced) and less steep regional monoclines are rather associated with inherited Mesozoic structural highs. Some of the geophysical constraints (heat flow distribution, crustal and lithospheric thickness, Bouguer anomaly) available for the Italian area are summarized in Figs. 5 and 6.
Magmatism is rather controlled by lithospheric scale discontinuities, as clearly evidenced by the roughly linear alignment of the Cenozoic igneous rocks of the Alps, and by active geodynamic processes, as observed in Sardinia, Sicily, along the Italian peninsula, the south-eastern Mediterranean and the Sicily Channel.
Figure 4. Earthquake hypocentres and Centroid moment sensor solutions
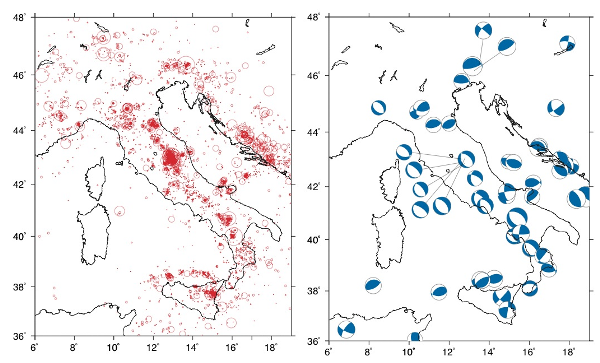
a) Earthquake hypocentres recorded by the Italian telemetered seismic network (ITSN) in the years 1989-1998 whose magnitudes are greater than 2.4. Data from INGV (http://www.ingv.it). b) Centroid moment tensor solutions available for the Italian area. Only earthquakes shallower than 40 km were selected. Data from the Harvard CMT Catalog (http://www.seismology.harvard.edu/CMTsearch.html).
Figure 5. Crustal and lithospheric thickness
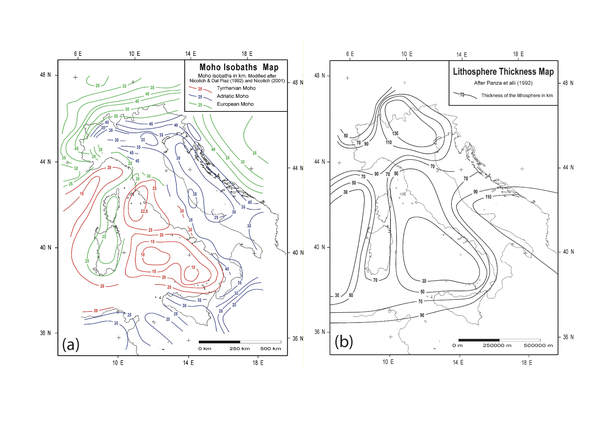
(a) Crustal and (b) lithospheric thickness, (after Nicolich and Dal Piaz, 1992; Nicolich, 2001; Panza et al., 1992, in Scrocca et al., 2003).
Figure 6. Bouguer anomalies and heat flow density
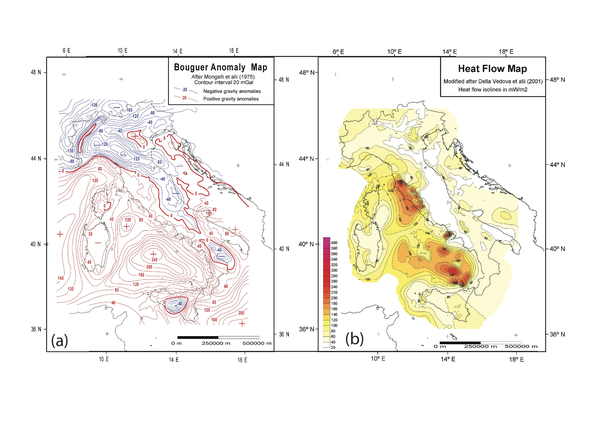
(a) Bouguer anomalies and (b) heat flow density of the Italian area (after Mongelli, 1975; Cataldi et al., 1995; Della Vedova et al., 2001).
Alps
The Alps (Figs. 3 and 7) show a double-vergence growth, with the involvement of large volumes of basement and the exhumation of metamorphic rocks. These rocks belong to the European (Helvetic domain in the literature), oceanic (Penninic domain), and African realms. The Africa-derived rocks belong actually to the Adriatic (also known as Apulian) micro-plate and are called Austroalpine and Southalpine domains, if cropping out south and north of the Insubric Line respectively. The western and central Alps are characterized by a discontinuous belt of crystalline massifs, which disappear eastward in the Pannonian Basin, being buried under thousands of meters of Neogene sediments (Fügenschuh and Schmid, 2003). The foredeep of the Alps has the deepest depocenter to the north of the central Alps (Fig. 8). Lithospheric-scale cross sections through the Central and Western Alps are provided in Figs. 7. The Southern Alps (Fig. 7) are a south-verging thick-skinned fold-and-thrust belt composed of basement and cover rocks belonging to the Adriatic microplate, which were affected at most by very low-grade metamorphism during the Alpine orogeny (e.g., Carminati et al., 1997 and references therein). The Southern Alps are separated from the north-verging Alpine, mainly metamorphic, orogen by the Insubric Line, a dextral transpressive mylonitic-to-cataclastic belt active since Oligocene (e.g., Rosenberg, 2004 and refences therein). In the Alps, water divide and highest peaks are roughly coincident (Fig. 9).
The onset of compressional deformation in the Alps during Late Cretaceous-Paleocene drove to Europe-verging nappe formation associated with high-pressure metamorphism (eclogites and eclogite-facies continental rocks; Dal Piaz et al., 1972; Spalla et al., 1996; Berger and Bousquet, 2008) and to deposition of coeval flysch (Polino et al., 1990). High P/T (e.g., Ernst, 1971) and ultra-high pressure (e.g., Chopin, 1984) metamorphic rocks and flysch deposits are mainly found in the Penninic accretionary prism and in the Alpine Corsica units (e.g., Spalla et al., 1996; Molli et al., 2008 for reviews). Compressive deformations are recorded, at this stage, also in the Southern Alps (e.g., Doglioni and Bosellini, 1987; Carminati and Siletto, 1997; Carminati et al., 1997). These deformations were interpreted as the result of the oceanic subduction stage.
Figure 7. Lithospheric scale cross sections through Alps and Apennines
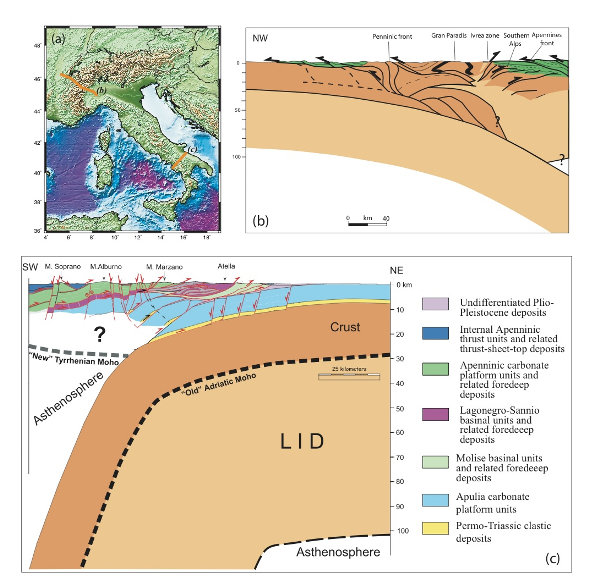
Lithospheric scale cross sections through the (b) western Alps (redrawn and modified after Roure et al., 1990b) and (c) southern Apennines (modified after Scrocca et al., 2005). The traces of the sections are shown in panel a. Panel c also shows the vertical motions along the transect and their sources.
Following the diachronous Eocene-Oligocene? Adria-Europe collision, north of the Insubric Line the Penninic nappes were thrust north onto the foreland forcing the detachment of sediments from their original substratum (the European plate), leading to the formation of the Helvetic nappes. Early Eocene ages for the collision in the Central-Eastern Alps are supported by the Paleocene-Early Eocene age of the youger flysch (sediments interpreted as deposited in trench environment) deposits (Stampfli et al., 1998) and by the 42-40 Ma Ar-Ar ages for the pressure peak metamorphism in the rocks belonging to the subducted oceanic realm (Wiederkehr et al., 2009). Such pressure peak is expected to postdate by a few Ma the subduction of the rocks. On the contrary, in the Western Alps, the youngest flysches were dated as Late Eocene to possibly Early Oligocene in age (Bagnoud et al., 1998). Young ages (Late Eocene-Oligocene) for collision are also supported by the young ages (as young as 35 Ma) of ductile shear zones developed at high-pressure metamorphic (i.e., tipically related to subduction preocesses) conditions, obtained from rocks belonging to the oceanic and to the European realms (Beltrando et al., 2010). Post-collisional shortening has been evaluated to be about 200 km in a N-S transect crossing central Switzerland (Schmid et al., 1995). Contemporaneous southward thrusting and associated folding occurred south of the Insubric Line, and shortening amounted to ~55 km in the central Southern Alps (Schönborn, 1992) and ~40-55 km in the Eastern Southern Alps (Castellarin et al., 2006). From the Miocene onwards, shortening in the Southern Alps outweighted the one occurred north of the Insubric Line. In the eastern Southern Alps and in the Carnian Alps (Fig. 3), interference occurred between the NW-SE-trending thrusts related to the pre-Neogene (Cretaceous-Paleogene?) forebelt of the Dinarides subduction and the ~E-W Neogene-Quaternary thrusts related to the structuration of the retrobelt of the Alp (i.e., the Southern Alps; Doglioni, 1987; Merlini et al., 2002; Ponton et al., 2010).
Figure 8. Alps and Apennines subduction zones and orogens
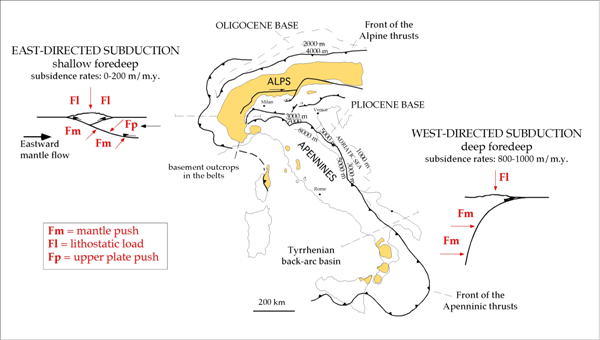
Alps and Apennines are orogens with very different characters, which may have resulted from markedly different subduction zones. The Alps have a shallow foredeep (the deepest depocenter has the Oligocene base at about 4 km), broad outcrops of metamorphic rocks (in yellow), and high structural and morphologic relief. The Apennines have deep foredeep (the deepest depocenter has the Pliocene base at about 8.5 km), few outcrops of basement rocks (partly inherited from earlier Alpine phase), low structural and morphologic elevation, and the Tyrrhenian back-arc basin. These differences mimic asymmetries of Pacific east-directed Chilean and west-directed Marianas subductions. For E-NE-directed subduction foredeep and trench, the origin may be interpreted as controlled by the lithostatic load and the downward component of upper plate push, minus the upward component of the "eastward" mantle flow (upper left panel). For the W-directed subduction where subsidence rate is much faster (>1 mm/yr), the foredeep origin may rather be interpreted as due to the horizontal mantle push and the lithostatic load [panel in the lower right (modified after Doglioni, 1994)].
In the central Southern Alps the post-Oligocene shortening was mainly accommodated by thrusting and folding along the Milano Belt, sealed by the ~6 Myr-old Messinian unconformity and buried under the Pliocene-Pleistocene sediments of the Po Plain (Figs. 3 and 7; Pieri and Groppi, 1981). Pliocene deformation was accomplished by out-of-sequence (i.e., positioned in more internal positions with respect to the outernmost front) thrusts (Schönborn, 1992). East of the Giudicarie Line (Fig. 3), that represents a sinistral transpressional tectonic lineament connected to the larger Insubric Line, the front of the Southern Alps crops out. With the exception of the NW-SE trending front of the Carnian Prealps (Merlini et al., 2002), such a front is segmented by transfer faults (e.g., Doglioni, 1992). In the eastern portions of the Southern Alps, N-S shortening is well documented from the Miocene to the present with an in-sequence thrust arrangement (Castellarin and Vai, 1981; Benedetti et al., 2000).
In the Alps (north of the Insubric Line), collision-related (Barrovian-type) metamorphism (Dal Piaz et al., 1972; Dal Piaz, 2001) has been recognized in the external zones north of the axial part of the chain, as well as in the central Alps (Lepontine dome) and in the Tauern window of the eastern Alps (Fig. 3 and 7; Bousquet et al., 2008). In the external zones, where only lower greenschist facies conditions were reached, metamorphism resulted from burial associated with nappe emplacement, thrusting and folding (e.g., Burkhard and Goy-Eggenberger 2001; Ferreiro-Maehlmann, 2001). The Lepontine area is characterized by widespread amphibolite facies metamorphism, but granulite facies conditions and migmatization were locally reached (Berger et al., 2005). The Lepontine metamorphism progressively decreases from upper-amphibolite facies conditions in the south (where it is truncated by the Insubric line, juxtaposing such high-grade rocks with rocks of the southern Alps that at most experienced Alpine anchimetamorphism) to greenschist facies conditions moving to the north (Streckeisen et al. 1974; Todd and Engi 1997; Engi et al., 2004; Berger et al., 2005).
Apatite fission track data from the central-western Alps show that erosion and exhumation concentrated in the internal sectors of the belt and in the Lepontine dome in Late Oligocene to Early Miocene time, whereas, in the last 15-2 Ma exhumation migrated to more external positions (i.e., northward), in the area of the external crystalline massifs (Fügenschuh and Schmid, 2003; Garzanti and Malusà, 2008). On the contrary, erosion was more stationary in the Eastern Alps, where it created a narrower metamorphic belt (Rosenberg and Berger, 2009).
Figure 9. Alps and Apennines water divide
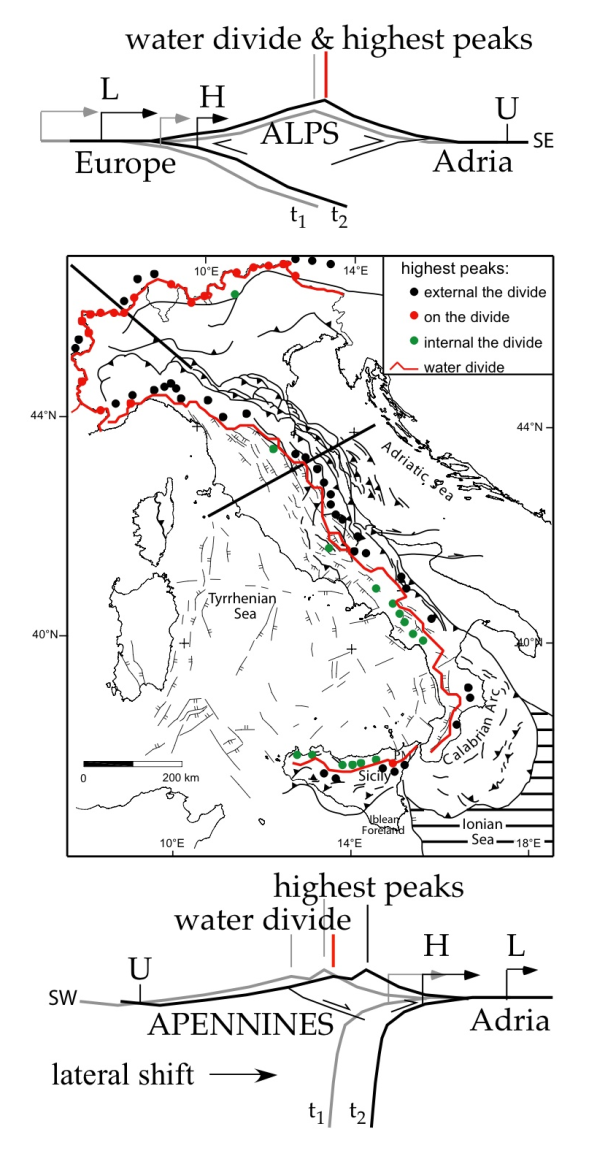
Alps and Apennines have a different relationship in the location and evolution of the water divide. In the Alps the water divide is more closely coincident with the highest peaks of the orogen. In the Apennines the water divide and the highest peaks are frequently shifted apart. The highest peaks often located to the east, apart for those zones where the subduction retreat is practically stopped and/or where the lithological contrast dominates the erosion rate (e.g., the Southern Apennines).
Figure 10. Total Alkali vs. Silica (TAS) diagram (Le Bas et al., 1986) for the Cenozoic igneous rocks of Italy.
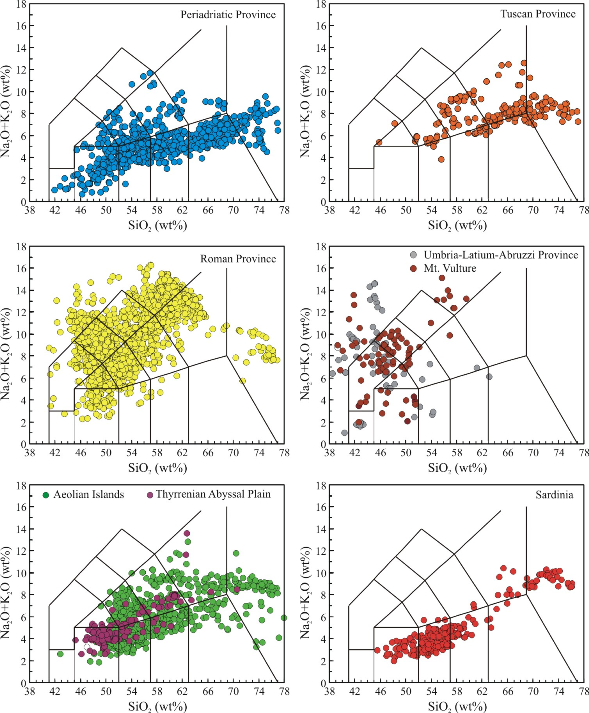
Top (a) = subduction-related (or “orogenic”) rocks. References in Lustrino et al. (2011).
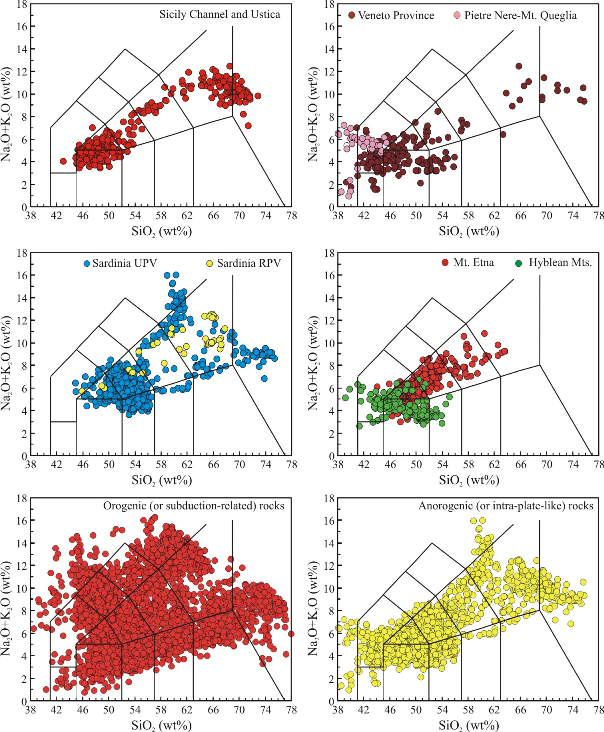
Bottom (b) = intra-plate-like (or “anorogenic) rocks. References in Lustrino and Wilson (2007) plus updates downloadable from the GEOROC web site (http://www2.mpch-mainz.mpg.de/~geo/Databases/GEOROC/Expert_Datasets.htm). The last two plots of fig. 7b show the Italian Cenozoic igneous rocks divided into “orogenic” and “anorogenic” groups. The full list of analyses (8754 whole rocks) and references can be requested to the second author.
Figure 11. Magmatic activity of the Italian area at different time spans, from 65 Ma to Present (a-h).
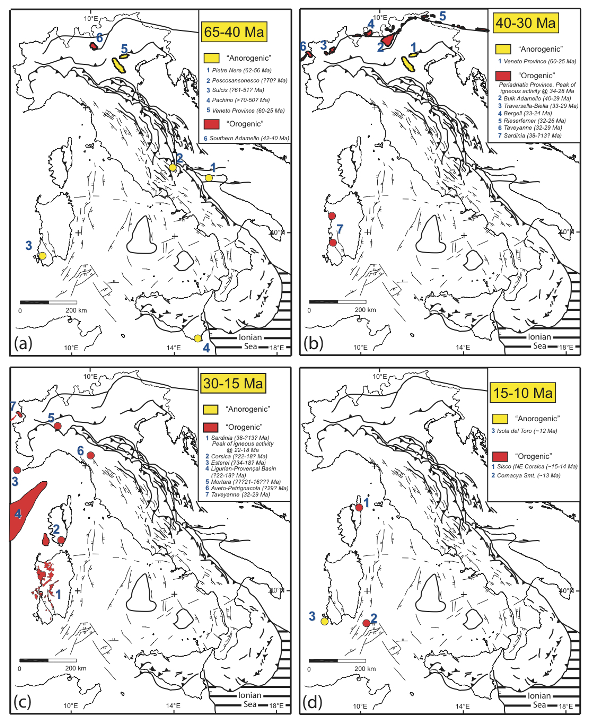
Yellow symbols: igneous rocks with chemical composition resembling magmas emplaced along active subduction zones (subduction-related or “orogenic”). Red symbols: igneous rocks with chemical composition resembling magmas emplaced in within-plate oceanic settings (intra-plate-like or “anorogenic”).
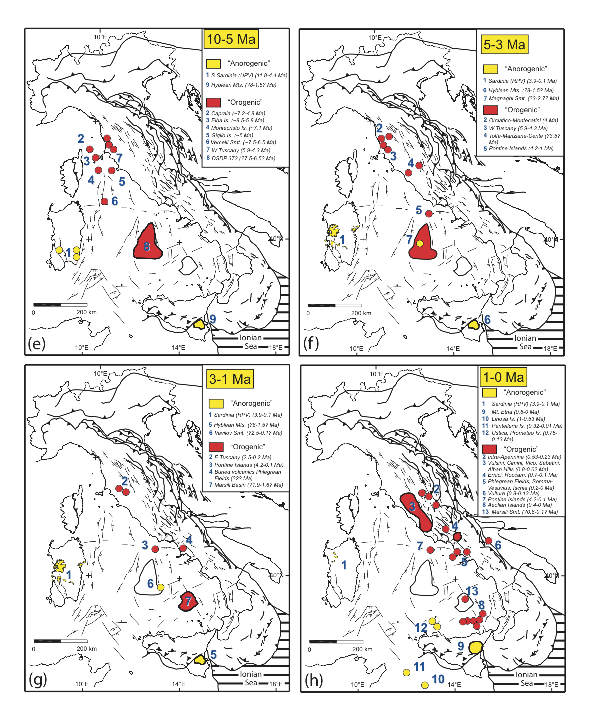
For a better definition of “orogenic” and “anorogenic” terms used in geochemistry applied to igneous petrology the reader is referred to Wilson and Bianchini (1999), Lustrino and Wilson (2007) and Lustrino et al. (2011).
The most ancient Cenozoic igneous activity observable close to the Alpine Chain started during Middle Paleocene and continued intermittently until Late Oligocene (~65-25 Ma) in the Veneto region, eastern Po Plain. The igneous rocks of the Veneto region cover an area of ~2000 km2 and are concentrated in four main volcanic districts (Lessini Mts., Marostica, Berici and Euganean Hills) with eruption of predominantly basic alkaline sodic lavas (mostly alkali basalts, hawaiites, benmoreites, trachytes) plus rarer Si-richer types (quartz trachytes and rhyolites; Fig. 10; references in Lustrino and Wilson, 2007).
Along the Alpine Chain, igneous activity developed mostly along the Insubric Line from ~42 to ~24 Ma (Fig. 11a-b-c), partially coeval with the igneous activity in the Veneto region (Rosenberg, 2004, and references therein). The bulk of the magmatism (>95% of the present-day outcrops) is emplaced in the time span 33-29 Ma and is represented by a syn-tectonic TTG (Tonalite-Trondhjemite-Granodiorite) association (Fig. 10). Minor gabbroic and dioritic stocks and even rarer calcalkaline and more exotic (i.e., lamproitic and kamafugitic) volcanic rocks are found mostly as dikes, especially in NW Alps (e.g., Venturelli et al., 1984; Conticelli et al., 2009, and references therein). With the exception of the plutonic rocks of southern Adamello, all the plutonic rocks emplaced along the Alpine Chain postdate or are coeval with the Eocene metamorphic peak (e.g., Rosenberg, 2004; Rosenbaum and Lister, 2005, and references therein).
Apennines and Sicily
The Apennines describe an arc from northwest Italy, down throughout the Italian peninsula, continuing to the southwest into Sicily and merging into the mountain belts (Maghrebides) of northwest Africa (Tunisia, Algeria and Morocco). The Apennine belt is mostly characterized by thin-skinned tectonics (Fig. 7; e.g., Bally et al., 1986; Scrocca et al., 2005, and references therein). The tectonic piling is radial, varying with continuity from N-NE (Northern Apennines) to NE (Central Apennines), E (Southern Apennines), E-SE (southernmost Apennines, Calabria) and S (Sicily; Fig. 3). The depth of the basal décollement plane at the front of the Apennines accretionary wedge varies significantly moving along strike (3 km in the Ionian Sea and 10 km in the Northern Apennines; Bigi et al., 2003; Lenci et al., 2004).
With the exception of the Calabro-Peloritani arc, the Apennines are mainly made up of Jurassic-Tertiary limestones (deposited both in shallow and deep-sea environments) and dolostones of African (Adriatic) origin (e.g., Patacca et al., 1989; Dercourt et al., 1992; Santantonio, 1994; Catalano et al., 2001; Ciarapica and Passeri, 2005; Santantonio and Carminati, 2010), Late Tertiary foredeep silicoclastic deposits (Ricci Lucchi, 1986, 1990; Mutti et al., 1999; Milli et al., 2007), Early Miocene carbonate successions (e.g., Carannante et al., 1988; Pescatore et al., 1999; Brandano et al., 2010) and subordinate ophiolitic melange (e.g., Montanini et al., 2008; Molli, 2008; Tortorici et al., 2008). The ocean, from which the ophiolitic bodies originated, separated the western margin of Adria from the southern European paleo-continental margin.
The geometry of the chain is shown by several seismic reflection and refraction studies acquired through the belt (Pieri, 1983; Scarascia et al., 1994; Cernobori et al., 1996; Barchi et al., 1998; Improta et al., 2000) and its foreland (e.g., Pieri and Groppi, 1981; Bally et al., 1986; Sella et al., 1988; Scrocca et al., 2003). As shown in Fig. 5, the Adriatic lithosphere is characterized by average continental lithosphere thickness (~70 km) increasing towards the belt (~110 km; Calcagnile and Panza, 1981). The Ionian lithosphere is ~90 km thick (Panza et al., 1992) and is rather interpreted as true oceanic (e.g., Finetti et al., 1996; Catalano et al., 2001) or transitional-to-continental (e.g., Calcagnile et al., 1982; Cernobori et al., 1996). A clearly defined Benioff-Wadati zone is present in Calabria-Peloritani-SE Tyrrhenian Sea, with hypocentre depths increasing towards NW (Finetti, 1982; De Voogd et al., 1992; Catalano et al., 2001; Chiarabba et al., 2008).
Although the Apennines were deformed mainly during Neogene-Quaternary (e.g., Boccaletti et al., 1990), remnants (thrust slices) of previously (Cretaceous-Paleogene) deformed rocks occur in the internal (western) portions of the belt (e.g., Abbate and Sagri, 1970; Principi and Treves, 1984). Associated with the east-directed tectonic piling, an “eastward” younging of foredeep sediments involved in the fold-and-thrust belt is recorded (e.g., Ricci Lucchi, 1986). Contemporaneous to compressional tectonics at the front, an “eastward” wave of extensional tectonics disrupted the inner portion of the belt, driving to the development of the Tyrrhenian Basin, of inland basins of western Italy and intra-mountain basins (e.g., Sartori, 1989; Bartole et al., 1995; Barchi et al., 1998; Cavinato et al., 2002).
Although the Apennines are generally made of unmetamorphosed cover rocks, metamorphic rocks are found in the northern (Tuscany) and southernmost (Calabria, as discussed later) sectors of the chain (e.g., Vignaroli et al., 2009 and references therein). Bimodal PT paths (HP metamorphism superimposed by a HT imprint) have been reconstructed for the rocks of the Tuscan Apennines and islands (e.g., Giglio and Gorgona Islands and Argentario Promontory; Apuane Alps; Rossetti et al., 1999, 2001a). The age of the HP stage has been constrained to ~31-27 Ma (Brunet et al., 2000) whereas the HT event occurred from 15 to 8 Ma (Kligfield et al., 1986; Molli et al., 2000).
Figure 12. Lateral migration of topography in the Apennines.
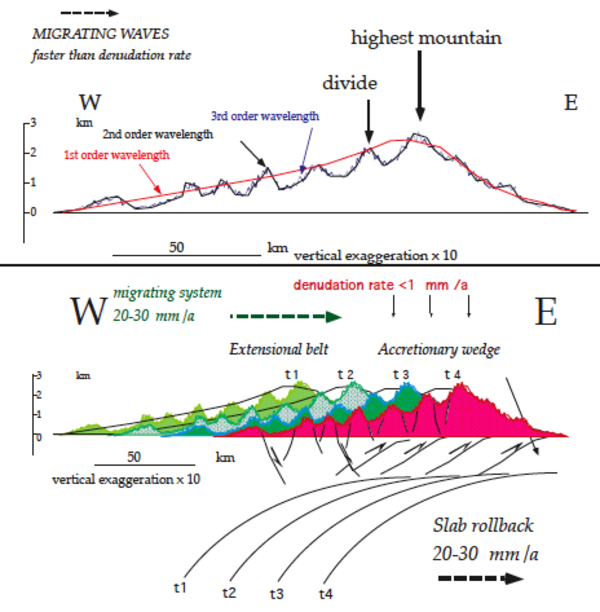
Above, idealised topographic section of the Apennines. The offset between divide and highest mountains appears to be associated to the faster effect of the tectonic ‘eastward’ migration with respect to the denudation rate. Deviations from this profile rely on strong lithological differences determining variable denudation rates, or slowing of the slab retreat rate of the Apennines subduction zone. At least three orders of topography can be distinguished: the first (> 100 km) due to geodynamic scale processes (subduction, depth of the decollement plane determining the volume of the accreted rocks in the prism, asthenospheric wedge under the belt); the second (5-15 km) determined by horsts and grabens spacing, or pre-existing folds; the third (0.5-2 km) related to smaller scale tectonics, lithologies and denudation rate. Below, interpreted relation between slab rollback and ‘eastward’ migration of the topography, in conjunction with the migration of the accretionary prism and the extensional belt. This simple model varies when slab rollback decreases, lithologies of the foredeep prevail in the emerged extensional belt decreasing frontal topography, and variations in depth of the frontal decollement plane (after Salustri Galli et al., 2002).
Frequently, the highest mountain peaks do not coincide with the water divide (Salustri Galli et al., 2002), but they are rather located toward the foreland to the East (Fig. 9). Since erosion is slower (0.7 mm/yr) than tectonics (1-10 mm/yr), the eastward propagation of the tectonic wave cannot be entirely compensated (Fig. 12). This generated a shift between the highest mountains and the water divide that remained more internal. In the Alps the lateral growth is not polarized as in the Apennines, and the water divide more frequently coincides with the highest peaks (Fig. 9). The salients and recesses in the Apennines accretionary prism front generally correspond to steeper or shallower foreland regional monocline respectively. These along-strike undulations appear controlled by the inherited grain of the Mesozoic Tethyan rift-related horsts and grabens, and still control the location of the water divide and the highest peaks (Fig. 13). The forward propagation of thrusts manly followed an in-sequence trend; however, an internal out-of-sequence thrusting can be inferred at the base of the foothills (Fig. 14).
The oldest Cenozoic igneous rocks cropping out along the Italian peninsula are found in southern Italy at Punta delle Pietre Nere, Gargano, Apulia region (Fig. 11a). This is a Paleocene (~62-56 Ma) small outcrop (~800 m2) of intrusive mafic alkaline rocks (mela-syenite and mela-gabbro) with lamprophyric (i.e., K-rich) affinity (Vollmer, 1976; de Fino et al., 1981; Bigazzi et al., 1996; Conticelli et al., 2009; Fig. 11a). A very small dyke swarm, about 15 m wide, containing 1-6 m thick dykes has been found in central Italy (Monte Queglia, Abruzzo region; Fig. 11a). These dykes, whose age has been constrained to Eocene time (<54 Ma), show overall mineralogical and chemical compositions resembling the Punta delle Pietre Nere rocks (Barbieri and Ferrini, 1984; Durazzo et al., 1984; Vichi et al., 1995; Fig. 10a). From Late Paleocene to Late Miocene no igneous activity is recorded along peninsular Italy. The only exception are Oligocene (~29 Ma) conglomerates, up to 400 m thick, rich in volcanic fraction (with boulders up to 1-2 m3) found in the Aveto-Petrignacola formation (Northern Apennines; Mattioli et al., 2002a; Fig. 11c) and a ~30 Ma-old buried volcano, covered by thousands of meters of sediments in the Po Valley (Mortara volcano; Mattioli et al., 2002b; Fig. 11c). The chemical and mineralogical composition of the Aveto-Petrignacola and Mortara rocks is quite similar, with abundance of andesitic, dacitic and rhyolitic composition with calcalkaline affinity (Fig. 10b). These rocks share mineralogical, petrographic and geochemical similarities also with the coeval (~32 Ma-old) volcaniclastic sandstones of the Taveyanne formation (NW Alps; Ruffini et al., 1997; Fig. 11c).
Figure 13. Relation between the regional monocline, the accretionary prism front, the divide and the highest topography.
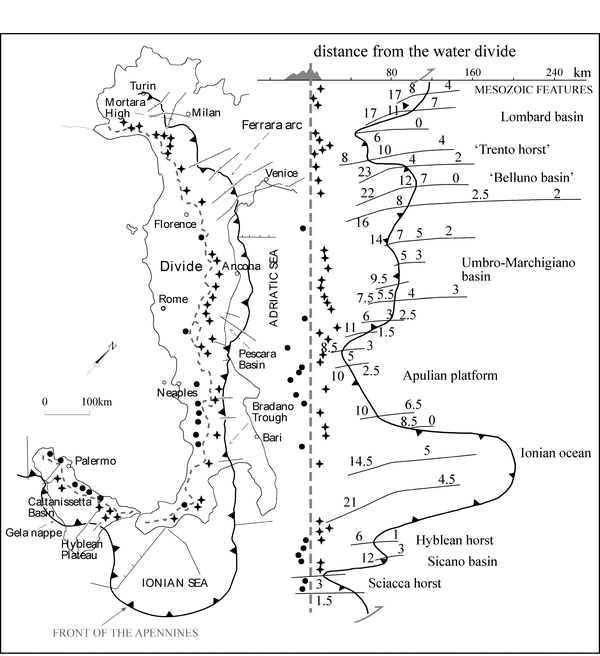
Relation between the regional monocline around the Apennines (grey lines; the numbers indicate the monocline dip in degrees), the accretionary prism front (barbed line), the divide (dashed line; modified after Mariotti and Doglioni, 2000) and the highest topography (dots and crosses) in a map view (left), and normalized to the divide (right), marked with dots. In the central-northern Apennines the highest mountains are more displaced toward the foreland than along the southern Apennines where the thick continental lithosphere of the Apulian Platform encroached the trench and slowed the subduction zone. Salients and recesses of the accretionary prism follow the inherited Mesozoic grain (i.e., horsts and grabens). The water divide and the highest peaks miscorrelation appears to be controller as well by the Mesozoic architecture. The absence of a large offset in Calabria could be attributed to salt in the decollement at the base of the accretionary prism in the Ionian, to the high denudation rate of the crystalline rocks inland, and the presence of relevant transfer zones such as the Crati and Catanzaro grabens (after Salustri Galli et al., 2002).
Other sparse outcrops of Oligocene-Miocene igneous rocks are represented by pelitic to arenitic levels rich in volcanic fragments: the Bisciaro Formation (“Livello Raffaello”, possibly Aquitanian, central Italy; Montanari et al., 1991), the Upper Oligocene-Aquitanian marls of the Tertiary Ligurian-Piemontese Basin (e.g., “Marne di Rigoroso”, Monferrato; Ruffini and Cadoppi, 1994; “Marne di Antognola”; Morandi et al., 1991), the Late Oligocene volcaniclastic levels of the Ranzano Basin (Northern Apennines; Cibin et al., 1998), the Late Oligocene-Early Miocene Calabro-Lucano Flysch (Southern Apennines; Critelli and Monaco, 1993), the Late Oligocene volcaniclastic turbidites of the Tufiti di Tusa Formation (Northern Sicily; Carbone et al., 2005).
The igneous activity resumed from latest Miocene until present days. From ~8.5 to ~0.2 Ma a discrete igneous activity generated series of volcanoes and plutonic stocks mostly in the Tuscany region (North-Western Apennines) and offshore northern Tyrrhenian Sea (Poli, 2003; Peccerillo, 2005; Peccerillo and Lustrino, 2005; Avanzinelli et al., 2009; Fig. 11e). These rocks are grouped under the name of Tuscan Magmatic Province, and are represented both by crust- and mantle-derived melts, i.e., with pure crustal anatectic rhyolitic melts as well as liquids of pure mantle and hybrid (crust + mantle) origin (Fig. 10b). Plagioclase- and leucite-free lamproitic rocks are found in association with shoshonites, and high-K calc-alkaline rocks. Despite the name of the province, the southern outcrops of the Tuscan magmatic province crop out in northern Latium region (Tolfa-Cerite districts; Peccerillo, 2005; Fig. 11f).
Italy is particularly famous among Earth scientists for its Plio-Quaternary igneous activity developed along the Tyrrhenian coast with exotic chemical and mineralogical composition (Peccerillo, 1985, 2005; Beccaluva et al., 1991; Conticelli et al., 2002; Peccerillo and Lustrino, 2005; Avanzinelli et al., 2009; Lustrino et al., 2011). These became the subject of detailed mineralogical, petrographic and geochemical investigations more than one century ago. Washington (1906) recognised and defined for the first time the Roman Magmatic Province on the basis of the common presence of abundant leucite and the strong degree of SiO2-undersaturation. Since then, the volcanoes of this region have been fully investigated, and a wealth of geochemical, mineralogical, petrographic, volcanological and geophysical data is now available. The igneous activity in the Roman Magmatic Province started during early Pliocene (~4.2 Ma) and is still active in the southernmost sectors (Mt. Vesuvius, Ischia Island and Phlegrean Fields; Peccerillo, 2005, and references therein; Fig. 11g-h). From North to South, the main volcanic edifices are Vulsini Mts., Vico Volcano, Sabatini Mts., Alban Hills, Ernici Mts., Mt. Roccamonfina, Pontine Islands, Procida, Vivara and Ischia Islands, Somma-Vesuvius and the Phlegrean Fields (Fig. 11g-h). The oldest products (~4.2-1 Ma) crop out in the Pontine Island district as acid volcanic rocks (mostly trachytes and rhyolites; Fig. 10b). The SiO2-oversaturated composition of these rocks strongly contrast with the SiO2-saturated to strongly SiO2-undersaturated compositions of the Roman Magmatic Province products. On the basis of this difference, the Pontine Islands rocks are grouped in the Tuscan anatectic province by several authors (Peccerillo, 2005; Avanzinelli et al., 2009, and references therein) but are grouped in Figure 10b together with the Roman Magmatic Province. With the exception of the Pontine rocks and the thick volcanic successions buried beneath the Campania plain (where more than 1800 m thick andesites have been drilled by the national oil company), the main rock types belong to potassic SiO2-saturated (e.g., shoshonitic) and strongly potassic SiO2-undersaturated series (e.g., leucitites, tephrites to phonolites; Peccerillo, 2005; Conticelli et al., 2009; Avanzinelli et al., 2009; Lustrino et al., 2011, and references therein).
A completely different igneous activity (in terms of chemical composition of the volcanic rocks) developed in the central sectors of Apennines in a restricted time range. The rocks that belong to this activity are grouped in the Umbria-Latium-Abruzzo ultra-alkaline (or Intra-Apennine) Province (~0.6.0.4 Ma; Stoppa and Lavecchia, 1992; Castorina et al., 2000; Stoppa et al., 2003; Fig. 11h). The peculiar feature of this province is the presence of numerous monogenetic volcanoes, located along main extensional faults, with strongly SiO2-undersaturated composition, exotic mineralogical paragenesis and very limited areal extension. The main rock types are strongly SiO2-undersaturated melts like melilitolites, melilitites, Ca-carbonatites and ultrapotassic rocks (kamafugite group, with coppaelite and venanzite type localities near Cupaello and San Venanzo villages; Fig. 10b).
In terms of geographic position (southern Apennines, close to the Apennine thrust front), mineralogy (e.g., presence of exotic minerals like haüyne) and chemical whole-rock composition, the Mt. Vulture (or Lucanian Province) can be considered isolated from the main Roman Magmatic Province (Fig. 11h). The igneous activity developed during Pleistocene (~0.8-0.1 Ma; i.a., Melluso et al., 1996; Beccaluva et al., 2002; Peccerillo, 2005; De Astis et al., 2006) with emission of pyroclastic rocks with minor lava flows and domes. The main rock types of Mt. Vulture are basanites and trachy-phonolites with minor foidites, tephrites, phono-tephrites and melilitites (Fig. 10b), less potassic than the Roman district rocks and with haüyne joining leucite as the main feldspatoid. A carbonatitic (alvikite) small lava flow has been recently discovered in this district (D’Orazio et al., 2007, 2008).
In North-East Sicily, one of the largest volcanoes of the entire Mediterranean area crops out: Mt. Etna (Fig. 11h). The first igneous activity of this volcano has been dated ~0.6 Ma (Gillot et al., 1994) and since then it is still active. The first products are tholeiitic to transitional lavas, later evolved towards more sodic alkaline magma compositions (Tanguy et al., 1997; Schiano et al., 2001; Armienti et al., 2004; Corsaro and Pompilio, 2004; Lustrino and Wilson, 2007; Viccaro and Cristofolini, 2008; Fig. 10a). The most recent products have a mildly alkaline affinity and relatively evolved compositions (hawaiites, mugearites, benmoreites, trachytes; Fig. 10a).
Few tens of km south of Mt. Etna, the Hyblean Plateau volcanic province crops out (Fig. 11a-e-f-g). The most recent igneous activity (Miocene-Pliocene) includes abundant tholeiitic basalts, plus minor alkaline sodic mafic rocks basanites, alkali basalts, hawaiites, nephelinites and ankaratrites (Di Grande et al., 2002; Peccerillo, 2005, and references therein; Fig. 10a).
Po Plain and Adriatic Sea
The Po plain is the foreland basin of northern Apennines and Southern Alps (e.g., Doglioni, 1993). Active thrusts, related to tectonics in the Apennines (e.g., Pieri, 1993; Scrocca et al., 2007; Carminati et al., 2010), are buried beneath the Quaternary sediments of the southern Po Plain (Fig. 14 and 15). The inactive front of the central Southern Alps (i.e., west of the Giudicarie Line) is also buried beneath Po Plain sediments in the so-called “Milano Belt” (Laubscher, 1990). The south-verging front of the Southern Alps and the north-verging front of the Northern Apennines merge south of Milano, as shown by seismic lines (Pieri and Groppi, 1981; Consiglio Nazionale delle Ricerche, 1992; Fig. 3). The Pliocene-Quaternary sedimentary pile, made up of shallow-sea to continental mainly siliciclastic rocks (e.g., Amorosi, 1996), is as thick as 8 km, implying fast subsidence rates (>1 mm/yr) during the last 5 Ma (Fig. 8 and 15). Tectonic (e.g., lithospheric flexure, faulting and folding) and sedimentological (e.g., compaction) processes control these long-term vertical velocities (Carminati et al., 2003a). Shorter-term subsidence pulses in the area are controlled by ice cycles (e.g., Carminati et al., 2003b) and by human activities (Carminati and Martinelli, 2002). Geodynamics and climatological changes are the main drivers of the sedimentation (Vittori and Ventura, 1995; Garzanti et al., 2002), which has filled the basin with alternate layers of sand and clay. Gravel is present only near the Apennines chain in alluvial fan deposits (Ori, 1993; Amorosi et. al., 1996), and in fans in the foothills between the Alps and the Po River extending from the outlets of the Alpine rivers into the Plain (Guzzetti et al., 1997).
Figure 14. Timing of thrusting at the front of the Apennines accretionary prism
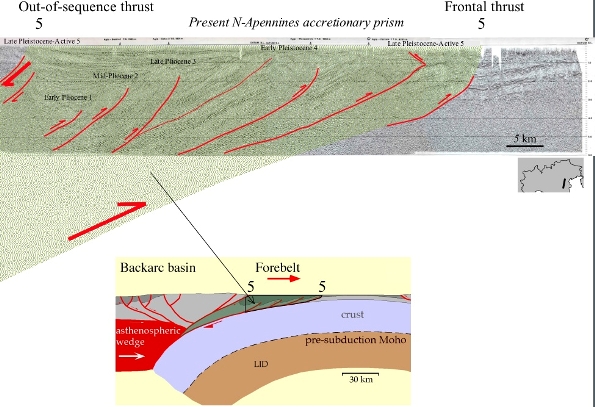
The front of the Apennines accretionary prism buried beneath the Po Basin shows an in-sequence foreland thrust propagation (1-5) since the Late Miocene-Early Pliocene up to Present. However, at the southern margin, an active out-of-sequence thrust deforms Peistocene sediments (5). The accretionary prism off-scraps only the shallower part (dominantly sedimentary cover) of the subducting lithosphere. Most the foredeep basin is located on top of the accretionary prism (piggy-back basin). In the section below the geodynamic interpretation. Seismic section after Pieri (1983).
The Adriatic Sea is the strongly segmented foreland basin of both Apennines and Dinarides-Hellenides (e.g., Royden et al., 1997; Carminati et al., 2004b; Scrocca, 2007). The Adriatic Italian offshore is characterized by fast subsidence rates (up to 1 mm/yr) in the northern part (e.g., Carminati and Di Donato, 1999), and rather by tectonic uplift (active since the Middle Pleistocene with rates of 0.3-0.5 mm/yr) in the southern Apulian part (Doglioni et al., 1994). The sedimentary fill of the basin is dominated by turbiditic siliciclastic sediments. As shown in Fig. 3, the front of the Apennines is located in the western portion of the northern Adriatic Basin and reaches the middle of the central Adriatic Basin (Scrocca et al., 2007). These fronts are buried under Pliocene-Recent sediments. The southern Adriatic basin, as well as onshore Apulia, form the foreland area of the Apennines, the Apenninic front being positioned well inland along the Bradanic Trough (e.g., Doglioni et al., 1994; Fig. 3).
Sardinia
The geological structure of Sardinia is the result of several tectono-magmatic processes that begun with the Late Paleozoic compressional tectonics that led to the consumption of large oceanic masses (Paleo-Tethys) and the amalgamation of continental plates into the super-continent Pangea (tectono-magmatic phase locally ascribed to the Hercynian Orogeny; see Compagnoni et al., this volume). The back-bone of this island is made up of plutonic and metamorphic rocks related to the shortening of the Hercynian Orogeny and the following relaxation tectonics that led to a disruption of the recently amalgamated super-continent. During the Carboniferous-Permian, Sardinia (and Corsica) experienced abundant production of calcalkaline to high-K calcalkaline granitic-granodioritic magmatism (e.g., Tommasini et al., 1995; Ferré and Leake, 2001) plus minor gabbroic-tonalitic and volcanic activity (calcalkaline to sodic alkaline dykes), partially coeval with, or pre-dating continental rifting that dismembered Pangea (see Lustrino, 2000, for a review).
Up to the Eocene, Sardinia and Corsica were part of the southern margin of Europe. During Late Eocene, a first extensional phase, roughly coeval with extensional tectonics in central Europe (Rhine Graben) and SE France (Alés, Manosque-Forcalquier and Camargue Basins; Séranne, 1999) developed in SW Sardinia with the formation of the E-W-trending Cixerri Graben. A second, more important, Late Oligocene extensional phase affected the entire Sardinia-Corsica micro-plate and resulted in the development of NE-SW directed rifting system that evolved into continental drifting during Late Oligocene-Early Miocene (e.g., Alvarez et al. 1974; Vigliotti and Langenheim 1995; Speranza et al., 2002; Gattacceca et al., 2007, and references therein). The Oligocene rifting in Sardinia was synchronous to the Ligurian-Provençal basin rifting, dated from the Oligocene (~34 Ma) to the middle Aquitanian (21 Ma) according to the age of the sediments drilled in the Gulf of Lion, just beneath the break-up unconformity (Gorini et al., 1993). Burdigalian extensional tectonics is also recorded in onshore grabens (Maerten and Séranne 1995). At a poorly constrained time (~22 Ma) the Sardinia-Corsica block started to rotate counter-clockwise away from the southern Europe paleo-margin, with a rotation pole located around the gulf of Genoa (Liguria, NW Italy; Speranza et al., 2002; Gattacceca et al., 2007). The rifting and drifting tectonics associate with this rotation led to the formation of the Ligurian-Provençal Basin, possibly floored by oceanic crust (e.g., Gueguen et al., 1998; Rollet et al., 2002; Schettino and Turco, 2006). The rotation ended around the Langhian (~15-16 Ma; see Lustrino et al., 2009, for a review) when intense igneous activity occurred (Brotzu, 1997; Lustrino et al., 2004, 2009). The Oligocene-Aquitanian tectonics of Sardinia is dominated by N-S trending normal faults (present-day coordinates) bounding a graben system that cut through pre-Alpine basement Sardinia from North to South for a total length of about 220 km and an average width of 50 km (Casula et al. 2001; Faccenna et al., 2002; Cherchi et al., 2008). The Sardinian Trough (also known as Fossa Sarda) is filled by up to 2 km of Upper Oligocene syn-rift (mainly non-marine sediments followed by fluvial-deltaic sediments, marls and silts) and Burdigalian-Messinian post-rift (marine marl-silt successions) deposits (Cherchi et al., 2008, and references therein). Starting from the Pliocene a new, NW-SE trending graben developed in SW Sardinia (Campidano graben; e.g., Balia et al., 1991), partially overlapping the southern sector of the Fossa Sarda.
As it concerns the Cenozoic igneous activity of Sardinia, the oldest products are Paleogene (~61-51 Ma) lamprophyric dykes (camptonite) drilled in the SW sector of the island (Sulcis area; Maccioni and Marchi, 1994; Fig. 11a). These dykes show major and trace element content roughly similar to the Pietre Nere and Mt. Queglia alkaline rocks (see above; Fig. 11a). Apart for these volumetrically insignificant products, the bulk of the igneous activity started during Late Eocene. With the exception of a single sub-volcanic outcrop dated at ~38 Ma (Lustrino et al., 2009; Fig. 11b), the bulk of the igneous activity in Sardinia started at ~32 Ma, during the peak of the igneous activity along the Insubric Line. The peak of the activity was concentrated around 22-18 Ma, in concomitance with the fastest counter-clockwise rotation of the island (Lustrino et al., 2004, 2009; Gattacceca et al., 2007; Fig. 11b-c). During the peak of the volcanic activity in Sardinia, the magma production along the Alpine Chain and in the Veneto area had already ceased. The igneous rocks of Sardinia are volcanic (<1% of plutonic rocks), essentially in ignimbritic facies, with minor lava flows and domes. These have mostly medium- to high-K calcalcaline composition, with rare arc-tholeiitic types. Very rare peralkaline compositions are produced during the last stages of igneous activity (with the type-locality of comendites in S. Pietro Island, Sulcis district, SW Sardinia; Morra et al., 1994). Dacitic to rhyolitic ignimbrites are the most abundant rock types, followed by andesitic, basaltic andesitic and minor basalts (Morra et al., 1997; Lustrino et al., 2004, 2009, and references therein; Fig. 10b). The igneous activity in Sardinia stopped around 15-13 Ma, after the end of counter-clockwise rotation of the Sardinia-Corsica micro-block (Beccaluva et al., 1985; Speranza, 2002; Gattacceca et al., 2007; Lustrino et al., 2009).
Figure 15. Long term subsidence rates for the Po Plain.
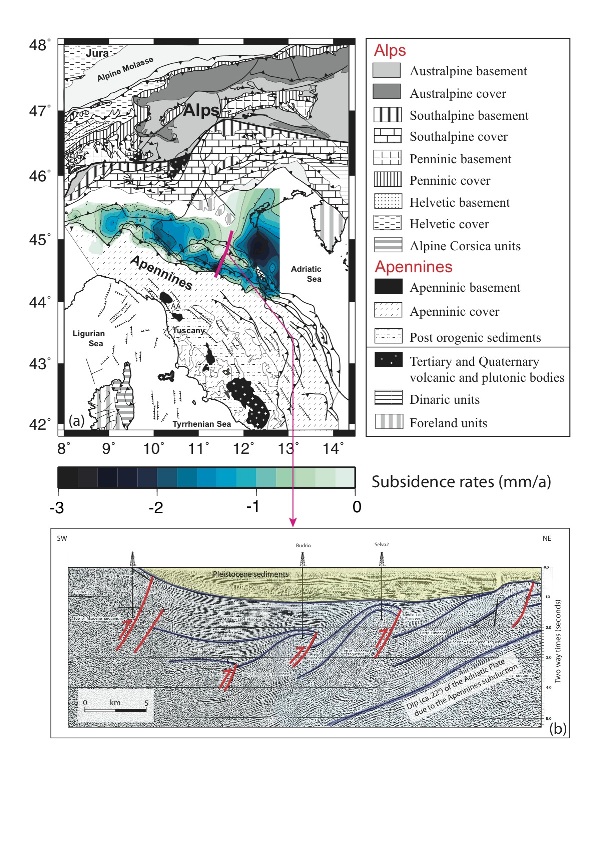
(a) Long term (averaged over the last 1.4 Ma) subsidence rates for the Po Plain (after Carminati and Di Donato, 1999). AA: Apuane Alps. (b) Seismic section through the front of the Northern Apennines (interpreted from Pieri, 1983). The trace of the section is shown in panel a.
Early Oligocene-Early Miocene (~34-16 Ma) calcalkaline and high-K calcalkaline rocks (andesites, micro-diorites, dacites and rhyolites, often emplaced as ignimbritic flows) are also found along the Provençal coast in southern France (Nice-Cap D’Ail area; e.g., Ivaldi et al., 2003; Beccaluva et al., 2005; Lustrino et al., 2), SE Corsica (e.g., Ottaviani-Spella et al., 2001) and offshore western Corsica (Rossi et al., 1998). In the late Middle Miocene (~11.8 Ma) a new phase of volcanic activity developed in Sardinia, with mineralogical, chemical and isotopic characters completely different from the Late Eocene-Middle Miocene phase (Lustrino et al., 2007b; Fig. 11d). The products of this second volcanic phase are low-volume, low degree-melting products with sodic alkaline compositions, ranging from basanitic to trachytic (Fig. 10b), found only in the southern sectors of Sardinia (Fig. 11d-e). The youngest products of this second phase of Cenozoic igneous activity of Sardinia (called RPV; Radiogenic Pb Volcanics; Lustrino et al., 2000, 2007a) are dated 4.4 Ma. After ~0.6 Ma a new volcanic activity (third phase) developed in the central-northern sectors of Sardinia (Fig. 11f-g-h) with mildly sodic alkaline and tholeiitic compositions (Fig. 10a) characterized by very peculiar trace element and isotopic features compared to the rest of the entire circum-Mediterranean Cenozoic igneous rocks (see below). The last products of this activity (called UPV group, i.e., Unradiogenic Pb Volcanics) are as young as 0.1 Ma (Gasperini et al., 2000; Lustrino et al., 2000, 2007a; Fig. 11h).
Calabria and Sicily
After the cessation of the Sardinia-Corsica block rotation (~15 Ma), the eastward extensional wave shifted to the East of this block, leading to the extension and break-up of its easternmost margin: the Calabrian-Peloritani terrane (see the movie). This terrane was separated from Sardinia probably during the Langhian and drifted towards the SE up to its present position. The Calabrian-Peloritani Arc (Fig. 3) is the link between the Southern Apennines and the Maghrebian belt, but differs from these being constituted of crystalline basement nappes, with a top-to-the-East to top-to-the South tectonic polarity. These nappes are both of continental (Hercynian granitoids and meta-granitoids) and oceanic (Neo-Tethys) origin (Amodio Morelli et al., 1976; Liberi et al., 2006; Johnston and Mazzoli, 2009; Vignaroli et al., 2009), plus sedimentary cover metamorphosed up to blue schist facies. HP-LT metamorphism in ophiolites and abyssal sediments from Calabria has been alternatively dated at 38–33 Ma (Rossetti et al., 2001b) or ~30 Ma (Iannace et al., 2007), thus possibly locating the HP event at the Eocene–Oligocene boundary (Vignaroli et al., 2009). No igneous activity is recorded in Calabria during the Cenozoic. This accretionary prism was thrust onto the Apenninic units, a continental platform formed by low-grade Paleozoic crystalline basement rocks covered by Mesozoic carbonate deposits, during the Early Miocene (Amodio Morelli, 1976, Ietto and Barilaro, 1993).
The Peloritani Mts. Belt (north-eastern Sicily) is composed mostly of crystalline units, metamorphosed mainly during the Hercynian orogenesis (De Gregorio et al., 2003), thrust onto the sediments of the African margin during the Tertiary. Late Eocene-Langhian syn-orogenic deposition, controlled by a combination of active thrusting, regional subsidence and sea-level change, was followed by sedimentation in basins controlled by normal faults from the Serravallian to the Pleistocene (Lentini et al., 1995). The Recent evolution of the Peloritani Mts. Belt is characterized by progressive uplift of the southern margins of the Tyrrhenian Basin, and local active subsidence related to down-faulting (Lentini et al., 1995).
Similarly to the Apennines, the bulk of the island of Sicily (south-west of the Taormina Line) is made up of Mesozoic-Cenozoic sediments thrust southward (although clockwise block rotations and oblique-slip thrusting also occurred) during the Neogene (Scandone et al., 1974; Oldow et al., 1990; Channell et al. 1990; Catalano et al., 2000). The Iblean Plateau, mainly comprising Mesozoic to Lower-Middle Miocene carbonatic rocks, discontinuously overlain by more recent sedimentary and volcanic rocks, is the foreland area of the Apennines-Maghrebian belt of Sicily.
Tyrrhenian Sea
During the last stages of the Late Eocene-Middle Miocene igneous activity of Sardinia (~15 Ma), northern Corsica was characterized by the intrusion of a small dyke swarm in the Sisco area (NE-most Corsica; Fig. 11d), with a peculiar lamproitic (i.e., SiO2- and MgO-rich, Al2O3- and CaO-poor ultrapotassic leucite-free) composition (e.g., Peccerillo et al., 1988; Conticelli et al., 2009). These are considered as the first magmatic activity associated with the opening of the Tyrrhenian Sea. Avanzinelli et al. (2009) grouped the Sisco lamproite with the Cornacya seamount (~12 Ma; SE Sardinia; Mascle et al., 2001; Fig. 11d) and the oldest products of Capraia Island (~7.2 Ma; Tuscan archipelago; Chelazzi et al., 2006; Gasparon et al., 2008; Fig. 11e) in the Corsica Magmatic Province on geochemical and petrological grounds.
A new phase of rifting affected easternmost Sardinia, its offshore and the Tyrrhenian Sea starting from Middle Miocene. The eastern Sardinia continental margin is characterised by half grabens filled by syn-rift sediments of Late Tortonian to Messinian age, including thick Messinian evaporites, while the post-rift Pliocene-Quaternary sequence drapes the previous stratigraphic units. A pre-rift terrigenous sedimentary sequence of Serravallian-Tortonian age occurs on the uppermost Sardinia margin (Sartori et al. 2001). The basement of these basins is characterised by crystalline-metamorphic Variscan and Alpine rocks (including Tethyan ophiolites) that recorded compressive deformation (Sartori 1986; Bigi et al. 1990; Doglioni 1992; Guerrera et al. 1993), covered by syn-orogenic clastic sediments.
The Tyrrhenian Sea is a triangular extensional basin, more than 3600 m deep in its central part, crossed by a major (probably lithospheric scale) discontinuity known as the 41st Parallel Line (Savelli and Wezel, 1979; Spadini and Wezel, 1994; Bruno et al., 2000). North of this lineament (in the Northern Tyrrhenian Basin), geophysical studies indicate the presence of a stretched continental crust ~20 km thick (Panza et al., 1992). Here only small amounts of igneous rocks (Tuscany Province) have been found (e.g., Peccerillo, 2005, and references therein). South of the 41st Parallel Line, more intense stretching of the continental crust (the crust is <10 km thick; e.g., Morelli et al., 1970) led to a diachronous oceanization in two sub-basins (Vavilov and Marsili, characterized by crust thinner than 6 km; e.g., Kastens et al. 1988; Sartori 1990). The depth of the Moho increases towards the continental margins reaching depths of 30 km along the Corsica-Sardinia margin. The lithosphere is as thin as 30 km under the central part of the basin (Panza et al., 1992), where large heat flow values (>150 mW/m2) are recorded (e.g., Della Vedova et al., 1984; Zito et al., 2003).
In the Tyrrhenian domain, similarly to Corsica and the Apuane Alps, extensional deformation is rlargely accommodated by ductile detachment faults disrupting an over-thickened (likely Alpine) belt, since the Late Oligocene-Miocene (Carmignani and Kligfield, 1990; Jolivet et al., 1990; Molli et al., 2006; Fig. 16). The first subsidence episode was recorded by Langhian sediments deposited along the eastern coast of the Corsica island (Orszag-Sperber and Pilot, 1976), coeval with the Sisco igneous activity (~15 Ma; see above). As already discussed for the eastern Sardinia margin, the major rifting phase in the Tyrrhenian basin occurred in Tortonian-middle Pliocene time (e.g., Trincardi and Zitellini, 1987) and became progressively younger toward the northeast (Bartole, 1995).
Figure 16. Lithospheric scale cross-section from Corsica to the Adriatic (modified after Carminati et al., 2004) and present-day vertical motions.
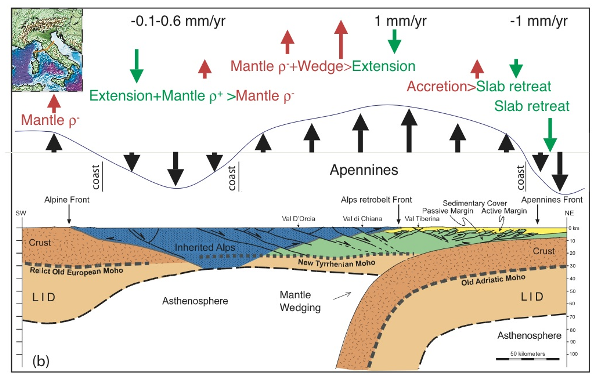
In this model, the Apennines prism (light green) developed along the retrobelt of the Alpine orogen (the blue double-wedge in the center of the section), where there was oceanic or thinned continental lithosphere able to subduct to the east of the pre-existing orogen. The alpine orogen was boudinated and stretched by the backarc rift. Above the cross section, are reported the vertical movements, their average rate (although lateral variations occur), and the interpreted origin. Note that several geodynamic mechanisms appear to coexist and control the uplift or subsidence along the different segments of the subduction-backarc system.
In the central sectors of the Tyrrhenian Basin, basins bounded by listric normal faults, cutting both Messinian-Quaternary sedimentary sequences and the underlying crust, are filled by Pliocene-Quaternary deposits and lava flows. These faults flatten close to the Moho and locally serpentinized mantle, tectonically overlain by stretched continental crust, crops out (e.g., Kastens, Mascle et al. 1987). The Tyrrhenian Sea is floored by igneous rocks in two main areas, the Vavilov and Marsili basins (e.g., Kastens, Mascle et al. 1987; Bonatti et al. 1990; Beccaluva et al., 1990; Sborshchikov and Al-Mukhamedov, 1992; Trua et al., 2002, 2004, 2007; Peccerillo, 2005; Fig. 11e-f-g-h). In the Vavilov basin ~7.5-3.5 Ma-od volcanic rocks have been dredged and cored, and two large volcanic structures have been identified: the Magnaghi seamount (~3.0-2.7 Ma) and the Vavilov seamount (~0.7-0.1 Ma; Trua et al., 2004, 2007). In the Marsili basin (~1.9-1.6 Ma; Fig. 11g) only a giant volcano has been identified, the Marsili seamount (<0.7 Ma; Fig. 11h). The composition of the Vavilov and Marsili seamounts range from T- (Transitional) to E-type (Enriched) MORB to calcalkaline basaltic and high-K andesitic, being N-MORB lithologies nearly absent (Fig. 10b). Sodic alkaline rocks (mostly hawaiites) have been dredged only along the Magnaghi seamount flanks, on the Quirra seamount (SW Tyrrhenian Sea) and rarely along the Vavilov seamount (Beccaluva et al., 1982; Robin et al., 1987; Peccerillo, 2005).
North of NE Sicily, seven major volcanic islands (Aeolian Islands: Filicudi, Alicudi, Panarea, Lipari, Vulcano, Salina and Stromboli: Fig. 11h) plus a large number of volcanic seamounts are active from ~1.3 Ma (e.g., De Astis et al., 2000; Calanchi et al., 2002; Gioncada et al., 2003; Peccerillo, 2005; Francalanci et al., 2004, 2007). Two of the seven volcanoes (Stromboli and Vulcano) are currently in activity. The Aeolian Islands are located on a 15-20 km-thick continental crust, above an active and seismogenic NW steeply dipping (70-85°) Benioff-Wadati plane (e.g., Chiarabba et al., 2008 and references therein). The igneous activity of the Aeolian Islands started with calcalcaline products (Sisifo seamount), continued with shoshonitic activity (Eolo and Enarete seamounts) and then ultrapotassic magmatism developed on the islands, together with the previous two magmatic series (Francalanci et al., 2007 and references therein; Fig. 11b). A general increase of potassium content of the magma is recorded in several islands (e.g., Vulcano and Stromboli) but this is not a rule for the entire district (e.g., Peccerillo, 2005; Francalanci et al., 2007).
Ustica is a small volcanic island located on a ~50 km-thick lithosphere along the southern margin of the Tyrrhenian Sea for a total height of ~2220 m (with less than 10% sub-aerial; Fig. 11h). It is made up of Quaternary volcanic rocks (0.75-0.13 Ma; De Vita et al., 1998) with mildly Na-alkaline affinity. The rocks range in composition from alkali basalts to rarer trachytes (Cinque et al., 1988; Bellia et al., 2000; Trua et al., 2003; Peccerillo, 2005; Fig. 10a). Interestingly, after a strong earthquake occurred in 2002 few km off-shore the NW coast of Sicily (about 40 km SE Ustica), the communications through the fibre-optic cable between Palermo and Roma were interrupted. After the recovery of the cable, it was observed that a side was completely burnt and that about 3 km of cable were locked. Favali et al (2006) interpreted this feature as the consequence of a volcanic eruption of a new active and still unknown volcano.
Sicily Channel
In the Sicily Channel the Apennines-Maghrebides belt is cross-cut by a NW-trending rift system. Three main elements mark the accretionary prism of Sicily and its off-shore continuation (Corti et al., 2006): (a) a Pliocene–Pleistocene northwest-dipping foreland monocline generating the overlying foredeep (Mariotti and Doglioni, 2000); (b) a roughly ENE-WSW to E-W-trending thin-skinned imbricate wedge, progressively emplaced from the Early-Middle Miocene to Present (e.g., Roure et al., 1990a; Catalano et al., 1996); (c) northwest-trending normal faults and related grabens or half grabens, associated with a Pliocene-Recent rifting phase (e.g., Reuther and Eisbacher, 1985; Torelli et al., 1995) that led to the development of the Sicily Channel. Toward the northwest, the Sicily Channel rift seems to be connected with the Campidano graben in southwest Sardinia (Corti et al., 2006). The rift affects also the Pelagian shelf and onshore Tunisia and possibly continues to the southeast into the Sirte basin in Libya (Corti et al., 2006). South of Sicily the rifting process produced the Pantelleria, Malta, and Linosa grabens.
The islands of Pantelleria and Linosa are the main volcanoes of the Sicily Channel district (Fig. 11h). These are located in a transtensive area of post-Miocene NW-trending horsts and grabens between Sicily and Tunisia. The igneous activity of Pantelleria (~118-4 ka) is younger than that of Linosa (1.06-0.53 Ma; Rossi et al., 1996). Both the islands are characterized by rocks with sodic alkaline compositions, with type-locality of pantellerites (peralkaline rhyolite) at Pantelleria (Esperança and Crisci, 1995; Civetta et al., 1998; Avanzinelli et al., 2004). The volcanic products of the island of Linosa are nearly indistinguishable from the Pantelleria rocks, but mafic to intermediate compositions are here more abundant than evolved lithotypes (Di Bella et al., 2008; Fig. 10a). The Sicily Channel district is also characterized by the presence of several volcanic seamounts (e.g., Graham and Nameless Banks, Tetide, Anfitrite, Galatea and Cimotoe seamounts, plus other minor volcanic centers; e.g., Beccaluva et al., 1981; Calanchi et al., 1989; Rotolo et al., 2006). Rock types are, in order of abundance, hawaiites, alkali basalts, basanites, mugearites and tholeiitic basalts (Fig. 10a).