Peridotite Petrology
Mantle peridotites from the Alpine-Apennine ophiolites are highly heterogeneous and show highly variable structural and compositional characteristics. They have been grouped into:
1) fertile spinel lherzolites;
2) depleted spinel harzburgites;
3) enriched plagioclase peridotites.
Fertile spinel lherzolites
The fertile lherzolites are clinopyroxene-rich, Ti-pargasite-bearing lherzolites that are usually veined by spinel(-garnet)-pyroxenite bands (Fig. 4) (e.g. Montanini et al., 2006; Piccardo et al., 2007b; Piccardo, 2008). They show porphyroclastic textures and spinel-facies assemblages and, in places, rounded spinel+orthopyroxene clusters suggesting spinel-facies recrystallization of precursor mantle garnet (Fig. 5A and B) (e.g. Hoogerduijn Strating et al., 1993). Mineral assemblages and equilibration temperatures (mean 1000°C) indicate that these peridotites equilibrated at lithospheric mantle conditions to an average sub-continental geotherm.
These peridotites are considered upper mantle rocks that were uplifted from garnet peridotite-facies conditions (P > 2.5 GPa) and equilibrated at spinel peridotite-facies conditions (2.5 GPa <= P <= 1.0 GPa) in the sub-continental lithosphere where they were resident prior to inception of Triassic-Jurassic continental extension and rifting in the Ligure-Piemontese domain. On the basis of their structural and petrologic characteristics, that indicate provenance from, and composite evolution in the lithospheric mantle, these pyroxenite-bearing spinel lherzolites have been referred to as lithospheric spinel lherzolites (e.g. Piccardo et al., 2007a, 2007b; Piccardo and Vissers, 2007).
The lithospheric lherzolite masses are frequently cut by km-scale extensional shear zones showing tectonite-mylonite fabrics (Fig. 4D). The shear zones usually show progressive syntectonic recrystallizations, starting from the oldest spinel-facies assemblages, which vary from spinel- to plagioclase- to amphibole-(chlorite)-peridotite facies parageneses, followed by shallow serpentinization (e.g. Vissers et al., 1991; Hoogerduijn Strating et al., 1993). The presence of these shear zones and their progressive syntectonic recrystallizations from deep to shallow lithospheric levels indicate that extension and thinning of the Europe-Adria continental lithosphere was, most probably, driven by a network of extensional shear zones (e.g. Piccardo et al., 2009). Accordingly, the pristine pyroxenite-bearing lherzolites from the sub-continental lithospheric mantle were progressively exhumed during continental extension and pre-oceanic rifting (e.g. Piccardo and Vissers, 2007).
Figure 4. Main features and field relationships of the sub-continental lithospheric spinel lherzolites and the reactive spinel harzburgites.
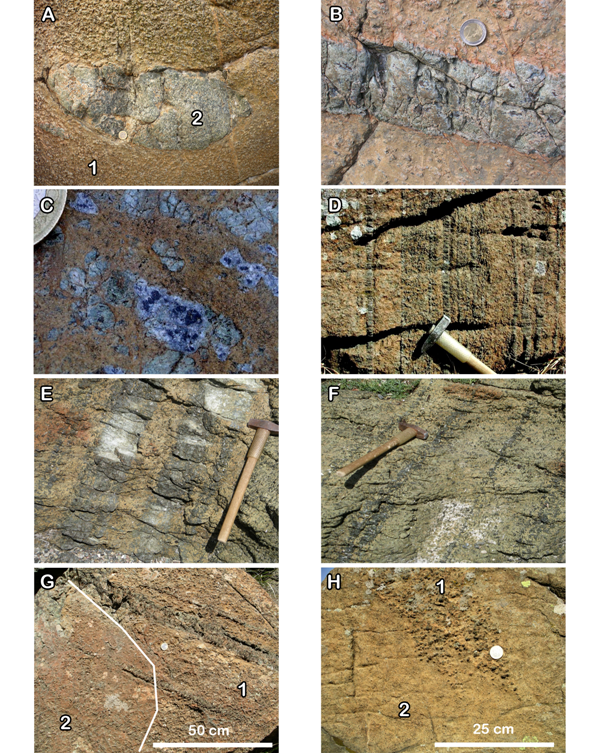
A) Pod of spinel pyroxenite (2) enclosed in lithospheric lherzolite (North Lanzo); B) Band of spinel pyroxenite enclosed in lithospheric lherzolite (North Lanzo); C) Cluster of orthopyroxene+spinel il lithospheric lherzolite: the cluster represents the breakdown of a precursor mantle garnet which indicate transition from garnet- to spinel-facies condition (Erro-Tobbio); D) Pyroxenite-rich tectonite-mylonite shear zone in lithospheric lherzolite which indicates that the sub-continental mantle underwent extension and exhumation (Erro-Tobbio); E) Lithospheric peridotite enriched in parallel pyroxenite bands (Monte Maggiore); F)Outcrop similar to E) where the diffuse percolation of a silica-undersaturated melt dissolved progressively the clinopyroxene of pyroxenites by melt-rock interaction (pyroxene dissolution/olivine crystallization), leaving out fanthoms of pyroxenites and strongly olivine-enriched reactive peridotites (Monte Maggiore);G) Primary contact between pyroxenite-bearing lithospheric lherzolite (1) and strongly pyroxene-depleted reactive spinel harzburgites (2). Harzburgite formed at the expense of lithospheric lherzolite by the reactive percolation of a undersaturated melt which dissolve pyroxenes (and pyroxenites) and crystallize olivine (Erro-Tobbio); H)Different types of reactive spinel harzburgites: 1) coarse granular spinel harzburgite; 2) fine-grained orthopyroxene-bearing dunite (South Lanzo).
Depleted spinel peridotites
Km-scale masses of clinopyroxene-poor lherzolites and harzburgites are abundant in the distal ophiolitic peridotite massifs, and are characterized by coarse granular isotropic textures and spinel-facies assemblages (Fig. 4). They usually lack of pyroxenite banding and show peculiar micro-textures which indicate dissolution of the mantle pyroxene porphyroclasts and formation of new unstrained olivine. Characteristic micro-textures consist of grains, patches and coronas of new olivine which replaces the pyroxene porphyroclasts and crystallizes interstitially between the mantle porphyroclasts (Fig. 5). These micro-structural features are interpreted as records of melt/peridotite interaction processes during the diffuse and reactive porous flow percolation of an asthenospheric melt through the lithospheric mantle (e.g. Piccardo et al., 2007a). Since these micro-textures indicate pyroxene-consuming/olivine-forming reactions, it is widely accepted that the percolating melt was silica(-pyroxenes)-undersaturated (e.g. Piccardo et al., 2007a; Piccardo and Vissers, 2007; Piccardo and Guarnieri, 2010b).
Progressive pyroxene dissolution and olivine precipitation transformed the pristine mantle lherzolites into strongly pyroxenes-depleted and olivine-enriched peridotites. Mineral assemblages suggest that these earlier stages of melt percolation and interaction occurred under spinel-peridotite facies conditions. The resulting coarse granular spinel harzburgites, which are significantly depleted in pyroxenes, enriched in olivine and are characterized by reaction micro-textures, have been referred to as reactive spinel harzburgites (Piccardo, 2003, 2008; Müntener and Piccardo, 2003; Piccardo et al., 2007a).
Enriched plagioclase peridotites
Km-scale bodies of plagioclase-bearing/rich peridotites are widespread within the distal ophiolitic peridotite massifs (Fig. 6). As a whole, these plagioclase-bearing rocks consist of an older, deformed and exsolved, spinel-facies assemblage and new mm-size, isolate plagioclase crystals and interstitial granular aggregates of plagioclase-rich gabbroic material. The most common micro-structural features are (Fig. 7): i) the presence of widespread undeformed plagioclase crystals which are interstitial or crosscutting deformed and exsolved mantle minerals; ii) the replacement of kinked mantle olivine by undeformed orthopyroxene patches; iii) the occurrence of mm-size plagioclase-rich gabbroic veins and pods, and symplectitic pyroxenes + plagioclase interstitial patches; and iv) rims of plagioclase surrounding the spinel crystals. These micro-structures are frequently coupled with clinopyroxene-dissolving/orthopyroxene+plagioclase-forming reactions (i.e. aggregates and coronas of orthopyroxene+plagioclase surrounding corroded clinopyroxene porphyroclasts) (Fig. 7) and interstitial crystallization of gabbro-noritic microgranular aggregates. Since these peridotites are characterised by olivine-consuming/orthopyroxene-forming micro-structures (Fig. 7), it is widely accepted that the percolating melt was silica(-orthopyroxene)-saturated (e.g. Piccardo et al., 2007a; Piccardo and Vissers, 2007; Piccardo and Guarnieri, 2010b). Presence and abundance of plagioclase suggest that these melt infiltration and interstitial crystallization events occurred under plagioclase peridotite-facies conditions. The resulting peridotites, which are variably depleted in olivine and enriched in plagioclase and gabbroic material, have been referred to as impregnated plagioclase peridotites (Müntener and Piccardo, 2003; Piccardo et al., 2004; 2007a; Piccardo and Vissers, 2007).
Figure 5. Representative micro-textures of lithospheric spinel lherzolites and reactive spinel harzburgites.
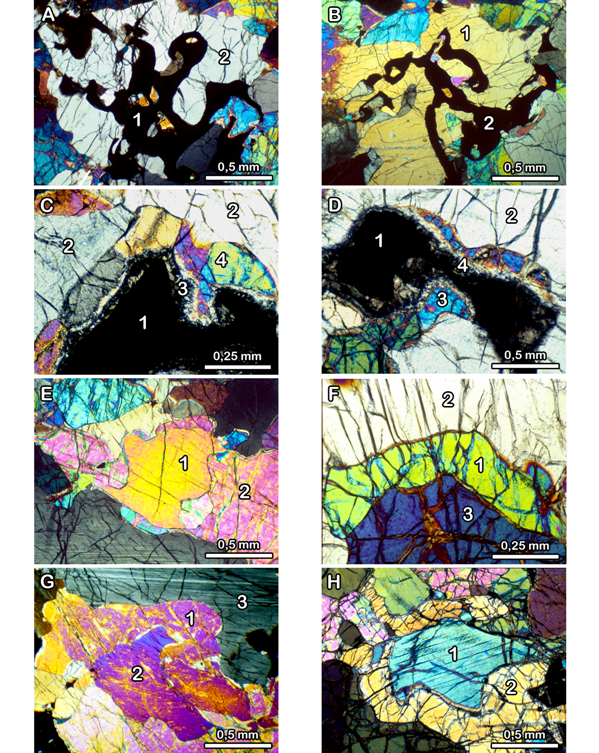
A) B) Rounded spinel (1) +orthopyroxene (2) clusters, which derive from the breakdown to and equilibration at spinel-facies conditions of a precursor mantle garnet in mantle peridotite. This micro-texture indicates the transition from pristine garnet-facies conditions to final equilibrium recrystallization under spinel-facies conditions (P < 2.5 Gpa), that can be interpreted as exhumation from asthenospheric or deep lithospheric levelts in the mantle and accretion to the lithosphere at spinel-facies conditions. C) D) Coronas of olivine (4) + plagioclase (3) (altered) between pyroxenes (2) and spinel (1). This reaction forming plagioclase + olivine marks the transition from spinel-facies to plagioclase-facies conditions in mantle peridotite. This micro-textures marks the transition from spinel-facies to plagioclase-facies conditions which indicates further exhumation of the peridotite to shallow lithospheric levels (P < 1.0 Gpa). E) Reaction micro-texture where a relict clinopyroxene porphyroclast (1) is partially replaced by new olivine (2). This micro-texture indicate melt-pyroxene reaction (pyroxene dissolution and olivine crystallization) operated by the reactive porous flow percolation of a silica-undersaturated melt. F) Rim of new magmatic olivine (1) between mantle orthopyroxene (2) and olivine (3). This micro-texture indicates olivine crystallization during porous flow percolation of and olivine-saturated melt. G) Reaction micro-texture where a relict clinopyroxene porphyroclast (2) is partially replaced by new olivine (1). Note that the new olivine replaces also the exsolved orthopyroxene porphyroclast (3), indicating dissolution of both pyroxenes. H) Reaction micro-texture where a relict clinopyroxene porphyroclast (1) is partially replaced by new olivine (2).
Figure 6. Main features and field relationships of impregnated plagioclase peridotites and replacive dunites.
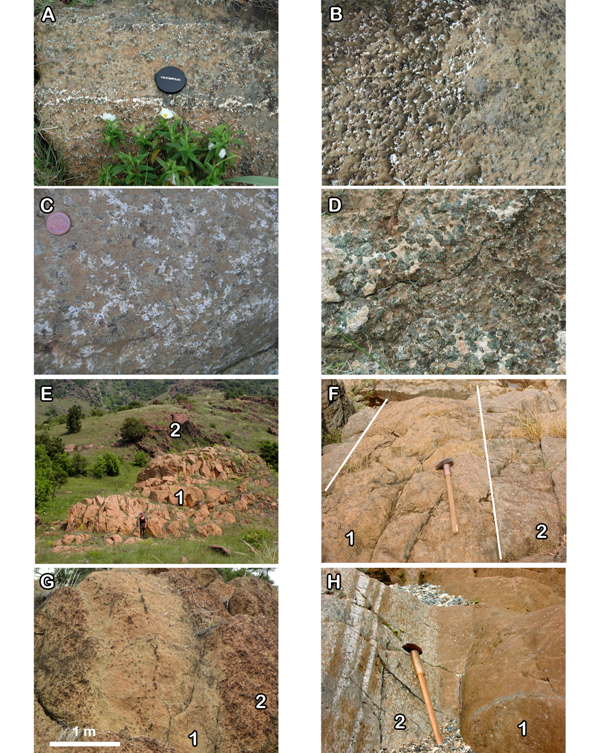
A) Strongly plagioclase -impregnated peridotite: note the thin plagioclase-rich gabbroic vein (Monte Maggiore); B) Contact between a strongly plagioclase -enriched peridotite and a reactive spinel harzburgite (Monte Maggiore); C) Strongly plagioclase-enriched peridotite (Erro-Tobbio); D) Pod of gabbro-norite highly enriched in euhedral pyroxenes (Monte Maggiore); E) Replacive spinel dunite body (1) within impregnated plagioclase peridotite (2)(South Lanzo); F) Metric replacive spinel harzburgite channel (1) cutting spinel peridotite (2)(Erro-Tobbio); G) Metric replacive spinel harzburgite channel (1) cutting spinel peridotite: note the clinopyroxene vein crystallized in the center of the channel from the melt rising within the channel (South Lanzo); H) Part of a decametric spinel dunite channel (1) running inside a strongly deformed tectonite-mylonite shear zone (2), following this shear zone foliation (Erro-Tobbio). These high permeability, high porosity olivine-rich channels are believed to drive the asthenospheric MORB magmas to shallow oceanic crustal levels.
Decametric-hectometric intrusive pods, decimetric dykes and centimetric dykelets with pyroxene-rich gabbro-norite composition are widespread within the impregnated plagioclase peridotites of the distal ophiolite massifs (Fig. 6). They are composed of clinopyroxene, orthopyroxene and plagioclase, and sporadically olivine. Pyroxenes are always abundant and usually show euhedral shape against anhedral and interstitial plagioclase. Orthopyroxene is an abundant cumulus phase. Abundance and early crystallization of orthopyroxene suggests that parental magmas of the gabbro-norite suite were relatively high-SiO2 basaltic liquids (Piccardo and Guarnieri, 2010c).
Figure 7. Representative micro-textures of the impregnated plagioclase peridotites.
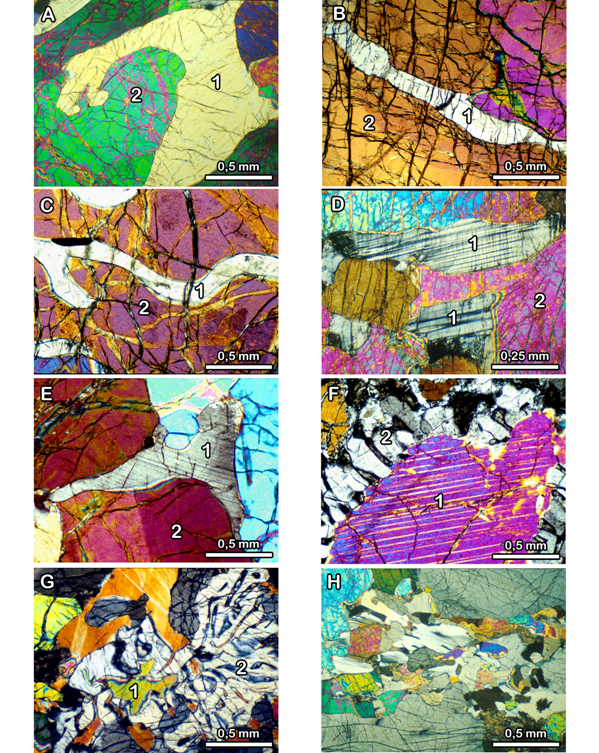
A) Orthopyroxene (1) replacement on mantle olivine (2). This micro-texture indicates reaction of the mantle olivine with a silica-saturated melt, forming orthopyroxene (olivine dissolution and orthopyroxene precipitation); B) C) Veins of orthopyroxenes cutting mantle olivine (olivine dissolution and orthopyroxene precipitation), indicating migration and reaction of a silica-saturated melt. D) E) Undeformed plagioclase crystals (1) cutting through mantle porphyroclasts (2), indicating interstitial crystallization from a percolating melt. F) G) Exsolved clinopyroxene porphyroclasts (1) partially replaced by an orthopyroxene + plagioclase (altered) symplectitic aggregate (2), indicating reaction, at plagioclase-facies conditions, with an orthopyroxene-saturated melt. H) Micro-gabbroic aggregate crystallized between mantle porphyroclasts, indicating peridotite refertilization by interstitial crystallization of a basaltic liquid.
Replacive dunite channels
All the above peridotitic rocks were locally crosscut by channels of spinel harzburgites and dunites (Fig. 6). These strongly pyroxene-depleted/free peridotites have been interpreted as replacive channels formed by the complete reactive dissolution of mantle pyroxenes by focused percolation of silica-undersaturated melts along pre-existing compositional and structural discontinuities (e.g. Piccardo et al., 2007a; Piccardo and Guarnieri, 2010a, and references therein). These rocks have been referred to as replacive spinel peridotites. In some peridotite massifs (e.g. South Lanzo, Erro-Tobbio, External Ligurides) these replacive harzburgite and dunite channels run concordantly inside tectonite-mylonite shear zones, indicating that the focused migration of melts was driven by these structural discontinuities (Fig. 6). The replacive channels sporadically host interstitial clinopyroxene grains, euhedral clinopyroxene megacrystals and gabbroic veins and dykelets, which are interpreted as products of initial crystallization of the melts percolating within the channels (Piccardo et al., 2007a).
Some discriminant compositional features
The compositional characteristics of most Alpine-Apennine ophiolitic peridotite bodies have been compiled from the literature and discussed together to the processes responsible for their formation and evolution (sources of data reported in Piccardo and Guarnieri, 2010a, and references therein).
Figure 8. Some bulk rock major element characteristics of the Alpine-Apennine ophiolitic peridotites.
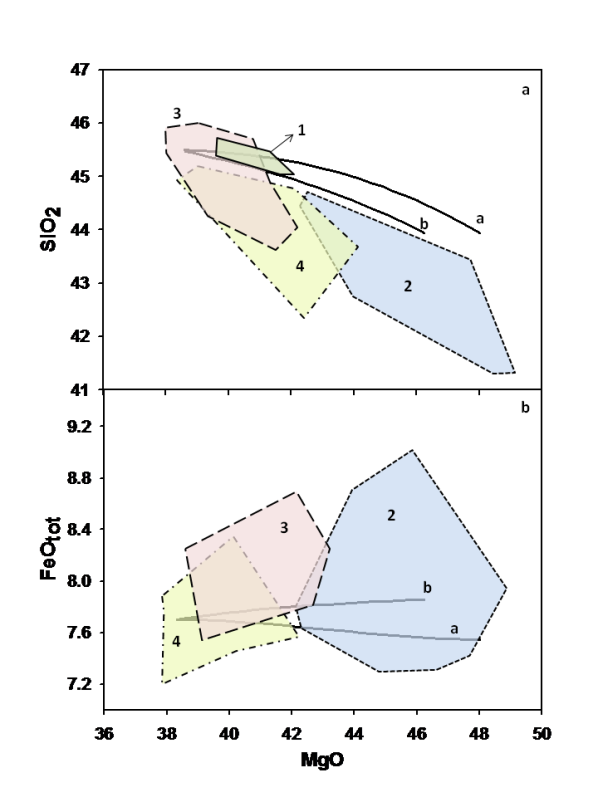
The data from the different sources have been grouped according to the palaeogeographic settings (i.e. marginal or distal peridotites) and petrologic features. 1) = Sub-continental lithospheric spinel lherzolites; 2) = Distal depleted spinel peridotites (reactive spinel peridotites); 3) = Marginal plagioclase peridotites (plagioclase peridotites impregnated by aggregated MORB melts); 4) = Distal plagioclase peridotites (plagioclase peridotites impregnated by MORB single melt fractions).
a) b) The various types of spinel peridotites are differently depleted in SiO2 and enriched in FeO with respect to refractory residua after any kind of partial melting, as calculated by Niu (1997) (trends a-b). In fact, the spinel distal peridotites (2) fall at significantly lower SiO2 contents and at significantly higher FeO contents with respect to the corresponding MgO values on the melting trends. In the MgO vs SiO2 diagram (a) the lithospheric sub-continental spinel lherzolites (1) broadly align along the melting trends at low degrees of melting, evidencing their rather fertile composition. The impregnated plagioclase peridotites from the distal settings (4) plot below the melting trends, at lower MgO values and increasing SiO2 contents than the distal spinel peridotites.
The samples from the different massifs have been grouped according to their different palaeogeographic settings (i.e. distal or marginal) and their structural-compositional characteristics. In the following, we present and discuss some discriminant compositional characteristics that are significant for giving indications on the petrogenetic processes recorded by the rocks (see a more complete discussion in Piccardo and Guarnieri, 2010a).
Bulk rock compositions of the Alpine-Apennine reactive spinel peridotites (Fig. 8) are differently depleted in SiO2 and enriched in FeO with respect to refractory residua after any kind of partial melting, as calculated by Niu (1997); this indicates that a simple partial melting and melt extraction process can not be responsible of their compositional features. This compositional evidence, together with the presence of reactive micro-textures, supports the reactive origin of these depleted spinel peridotites (e.g. Piccardo et al., 2007a; Piccardo and Vissers, 2007).
Bulk rock compositions of the Alpine-Apennine impregnated plagioclase peridotites (Fig. 8) plot at lower MgO contents than the reactive spinel peridotites, from which they derive, and at mostly higher SiO2 contents, sometimes higher than the estimated contents of primary mantle composition. This suggests that silica-rich mineral phases (plagioclase, pyroxenes) should have been added to the pristine peridotites, supporting the indication that the plagioclase peridotites underwent a melt refertilization event (e.g. Piccardo et al., 2004).
Spinel Cr# value spans over a wide range (6-50) and spinel TiO2 content ranges from very low (0.04 wt%) to significantly high (up to about 1.0 wt%) (Fig. 9a). It is widely accepted (Dick, 1989; Dick and Bullen, 1984; Hellebrand et al., 2002) that high Ti contents of spinel (i.e. TiO2 > 0.1 wt%) in oceanic peridotites is a record of melt-peridotite interaction and it indicates equilibration with MORB melts. Accordingly, relatively high TiO2 contents in spinel from the Alpine-Apennine distal reactive and impregnated peridotites confirm that they underwent reactive percolation of basaltic melts.
Figure 9. Some mineral chemistry characteristics of the Alpine-Apennine ophiolitic peridotites.
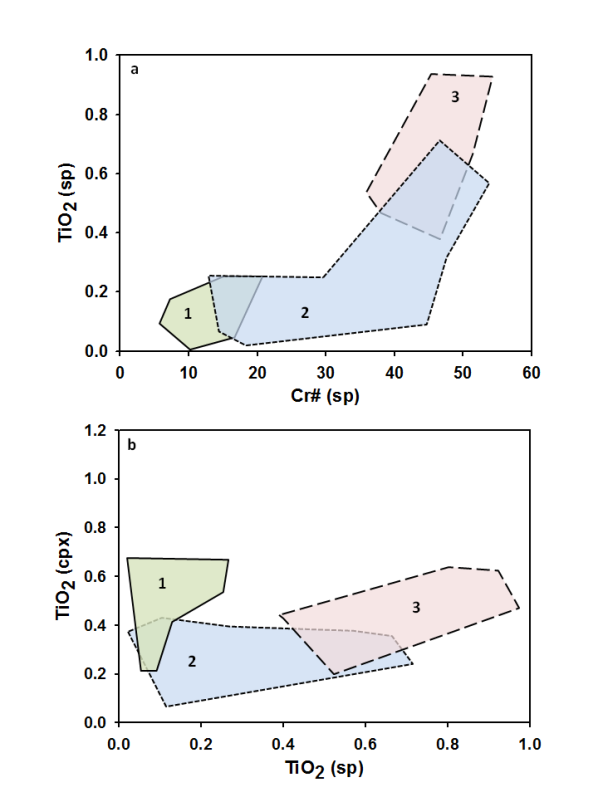
Peridotite groups as in Fig. 8.
a) Spinel Cr# spans over a wide range (6-50), the lowest values are shown by the fertile lithospheric sub-continental spinel lherzolites (1), the highest values are shown by the distal plagioclase peridotites (3). TiO2 content in Sp ranges from very low (0.04wt%) up to about 1.0 wt%. Significantly high TiO2 contents in spinel are shown by spinel and plagioclase distal peridotites (2-3) (Lower Platta, South Lanzo, Voltri Massif, Internal Ligurides, Mt. Maggiore).
b) TiO2 in Cpx ranges over a wide interval (0.12-0.98wt%). TiO2 in clinopyroxene and TiO2 in spinel show a broad positive correlation going from low-Ti, distal spinel peridotites (2) to high-Ti, distal plagioclase peridotites (3).
A broad positive correlation exists between TiO2 in clinopyroxene and TiO2 in spinel going from spinel to plagioclase peridotites (Fig. 9b). Parallel increase of Ti in clinopyroxene and spinel cannot represent the effect of closed-system metamorphic redistribution of Ti following spinel- to plagioclase-facies recrystallization, but must be more properly interpreted as due to an open-system process related to the progressive equilibration of both minerals with percolating Ti-bearing basaltic melts. Accordingly, the above compositional features support that the compositions of both Alpine-Apennine spinel and plagioclase peridotites were significantly influenced by melt-peridotite interaction processes.
Moreover, the different distal spinel peridotite masses are characterized by clinopyroxenes showing closely similar trace element compositions i.e., strongly depleted incompatible trace elements and C1-normalized REE patterns strongly fractionated in the LREE and almost flat in the MREE-HREE region (at about 10xC1) (Fig. 10a) (see discussion in Piccardo and Guarnieri, 2010a, and references therein). The spinel peridotites of the ophiolite massifs usually show clinopyroxene modal contents varying in a wide range (from 2 up to 10% modal content) in the same massif. Notably, the clinopyroxene REE patterns are closely similar, at the scale of the peridotite massif, disregarding the strong variation in the clinopyroxene modal composition of the different samples. Clinopyroxenes do not show progressive incompatible trace element depletion/fractionation concordantly with progressive decrease of the clinopyroxene content, as expected in the case they were formed by progressive partial melting.
The micro-structural evidence of melt-peridotite interaction during melt reactive percolation favours the interpretation that clinopyroxenes, although present in highly variable modal contents in the different samples, attained the trace element equilibration with a remarkably similar percolating melt during open system migration at high melt-rock ratios (Piccardo et al., 2007a). The close similarity of the clinopyroxene trace element composition in the different spinel harzburgite masses suggest, moreover, that the different harzburgites masses were equilibrated with percolating melts having closely similar geochemical affinities.
Figure 10. C1-normalized REE patterns of representative clinopyroxenes in distal reactive spinel peridotites and calculated equilibrium liquids.
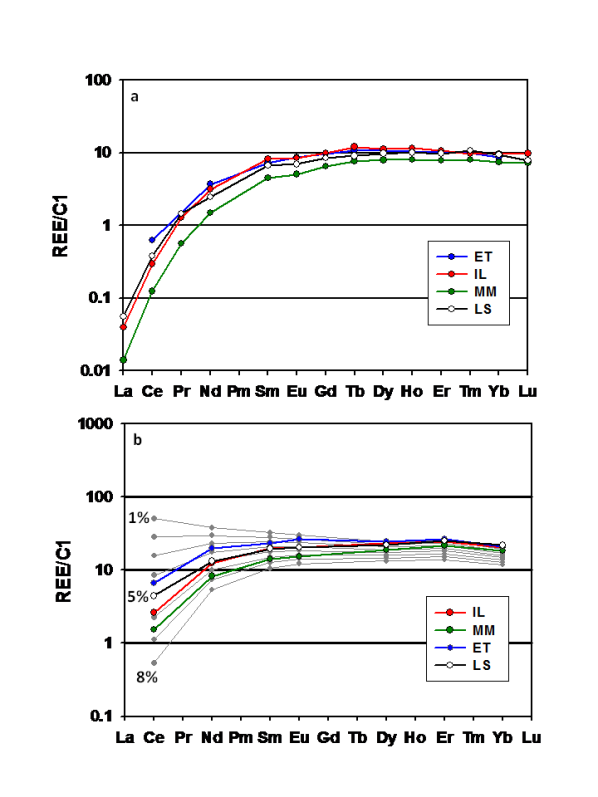
a) C1-normalized REE patterns of representative clinopyroxenes in distal reactive spinel peridotites from South Lanzo (LS), Erro-Tobbio (ET), Internal Ligurides (IL) and Mt. Maggiore (MM). The REE patterns are strongly fractionated in the LREE and almost flat in the MREE-HREE. The representative clinopyroxenes from the different peridotite massifs show closely similar REE contents and C1-normalized REE patterns.
b) The C1-normalized REE patterns of liquids calculated in equilibrium with the representative clinopyroxene core compositions (using Cpx/liquid Kds of Hart and Dunn 1993), from the reactive spinel peridotites of the different peridotite massifs. The REE patterns of liquids calculated by the fractional melting model from Johnson et al. (1990) [mantle source from Johnson et al. (1990), Cpx/liquid Kds of Hart and Dunn (1993)] are also reported for comparison. The calculated equilibrium liquids are quite similar to those of single melt increments modelled by 4-7% fractional melting of spinel facies DM asthenospheric mantle source.
Magmatic affinity of percolating melts
Information on the compositional characteristics of the percolating melts have been obtained by the clinopyroxene trace element compositions, assuming that the mantle clinopyroxenes of the percolated peridotites and the percolating liquids attained the trace element equilibration during melt-peridotite interaction under the conditions of high melt-rock ratios and open system percolation.
Concerning the reactive spinel harzburgites from the different distal massifs the REE compositions and patterns have been calculated for the liquids in equilibrium with representative clinopyroxene core compositions using the clinopyroxene/liquid KDs of Ionov et al. (2002) (Fig. 10). Concerning the impregnated plagioclase peridotites from the distal massifs the REE compositions and patterns have been calculated for the liquids in equilibrium with representative clinopyroxene core compositions using the clinopyroxene/liquid KDs of Vannucci et al. (1998), useful for saturated basaltic systems (Fig.11). The computed melt compositions have been compared with the REE composition of single melt increments produced by a fractional melting process [fractional melting model of Johnson et al., (1990), starting from the DMM (Depleted MORB Mantle) source of Johnson et al., (1990)].
The computed melts are almost coincident to MORB-type single melt increments after 4-7% of fractional melting of a spinel facies DM asthenospheric mantle source (Figs. 10b and 11b). It can be deduced that both the reactive spinel peridotites and the impregnated plagioclase peridotites were percolated at spinel- and plagioclase-facies conditions, respectively, by closely similar MORB-type single fractional melt increments showing closely similar geochemical affinities. It must be recognized that the early asthenospheric melts that infiltrated the lithospheric mantle at spinel- and plagioclase-facies conditions during continental extension and rifting were depleted single fractional melt increments that did not mixed with other melt increments and migrated isolated though the extending lithosphere (e.g. Piccardo and Guarnieri, 2010a, and references therein).
Figure 11. C1-normalized REE patterns of representative clinopyroxenes in distal impregnated plagioclase peridotites and calculated equilibrium liquids.
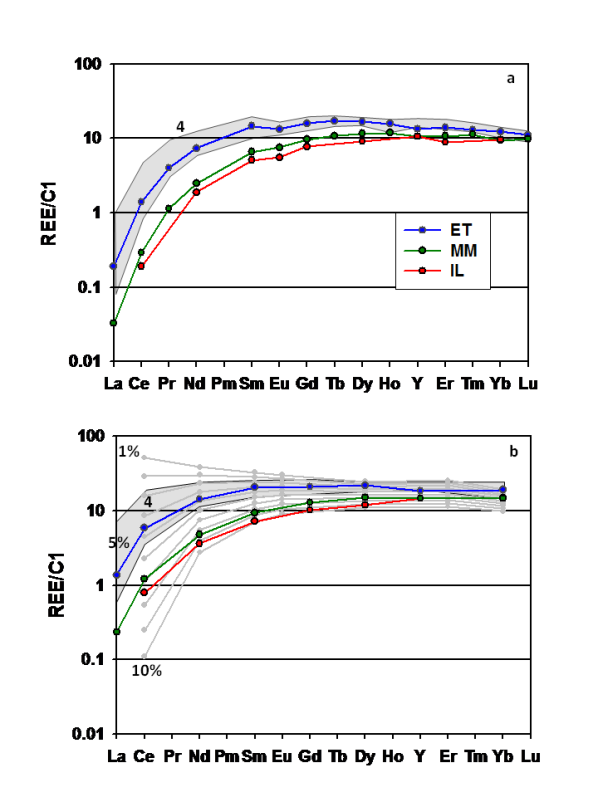
a) C1-normalized REE patterns of representative clinopyroxenes in distal impregnated plagioclase peridotites from Erro-Tobbio (ET), Internal Ligurides (IL) and Mt. Maggiore (MM) and South Lanzo (field 4). The distal plagioclase peridotites have clinopyroxene showing significant LREE fractionated C1-normalized REE patterns.
b) The C1-normalized REE patterns of liquids calculated in equilibrium with the representative clinopyroxene core compositions [using Cpx/liquid Kds of Vannucci et al. (1998), useful for saturated basaltic systems] from the impregnated plagioclase peridotites of the different peridotite massifs. The REE patterns of liquids calculated by the fractional melting model from Johnson (1990) [mantle source from Johnson (1990), Cpx/liquid Kds of Hart and Dunn (1993)] are also reported for comparison. The calculated equilibrium liquids are quite similar to those of single melt increments modelled by 4-7% fractional melting of spinel facies DM asthenospheric mantle source.
The Monte Maggiore study case
The compositional features of the Monte Maggiore peridotites furnish a scenario that well represents the evolution of the percolating melts (Piccardo and Guarnieri, 2010c).
Clinopyroxenes from the reactive spinel harzburgites are highly magnesian (Mg# 93-94), rich in Cr (Cr2O3 1.00-1.26 wt%) and strongly depleted in Na (Na2O 0.11-0.55 wt%), Ti (TiO2 0.29-0.34 wt%), Zr (1.38-2.78 ppm), Sr (0.80-2.01 ppm) and LREE (data from Rampone et al., 2008; Piccardo and Guarnieri, 2010b). Clinopyroxenes from the impregnated plagioclase peridotites are highly magnesian (Mg# 91), rich in Cr (Cr2O3 1.01-1.30 wt%) and strongly depleted in Na (Na2O 0.11-0.33 wt%), Ti (TiO2 0.27-0.47 wt%), Zr (2.83-5.60 ppm), Sr (1.00-5.17 ppm) and LREE (data from Rampone et al., 1997, 2008; Piccardo and Guarnieri, 2010c). Plagioclases are highly anorthitic (An 88-94) and strongly depleted in Sr (6.3-28.6 ppm) with respect to plagioclase in equilibrium with aggregated MORBs. Clinopyroxenes from the gabbro-norite suite are highly magnesian (Mg# 90.5-93.7), rich in Cr2O3 (1.18-1.42 wt%) and strongly depleted in TiO2 (0.28-0.43 wt%) and Na2O (0.17-0.30 wt%). They have very low LREE (LaNO = 0.04-0.28), Sr (0.8-3.5 ppm) and Zr (2.2-7.4 ppm) contents. Their C1-normalized REE patterns are rather flat in the HREE-MREE region (at about 8-15xC1) and are strongly LREE fractionated (LaNO/SmNO = 0.007-0.025). Plagioclase has very low LREE (e.g. CeN = 0.013-0.03) and Sr (15.6-30.4 ppm): C1-normalized REE patterns show a negative LREE fractionation (LaNO/SmNO = 0.07-0.96).
The compositional characteristics of minerals from reactive spinel harzburgites, impregnated plagioclase peridotites and gabbro-norite intrusives indicate that they equilibrated with strongly depleted melt fractions with MORB affinity. The computed melts in equilibrium with clinopyroxenes of peridotites and gabbro-norites show identical geochemical affinities and correspond to MORB-type depleted single melt increments formed by 5-7% of fractional melting of a spinel-facies DM asthenospheric mantle source (Piccardo and Guarnieri, 2010c).
Accordingly, at Monte Maggiore the melt migration and early crystallization stages were characterized by asthenospheric melts having almost identical LREE and incompatible trace element compositions, consistent with depleted single melt fractions. These melt increments infiltrated the lithospheric mantle at spinel-facies conditions at high melt/rock ratios and open system conditions and the peridotite minerals attained trace element equilibration with the percolating melts. Structural evidence indicate that during reactive percolation pyroxenes were progressively dissolved and olivine crystallized, causing progressive silica(-orthopyroxene)-saturation of the melts. It can be argued that the percolating melts maintain their primary strongly depleted geochemical signature, as recorded in the equilibrium minerals, during the reactive percolation at spinel-facies conditions but they were progressively modified from olivine-saturated to orthopyroxene-saturated compositions by pyroxene assimilation and olivine precipitation. The resulting strongly depleted, silica saturated basaltic liquids impregnated the mantle lithosphere at plagioclase-facies conditions and were later collected in dykelets and pods to form the gabbro-norite intrusive suite.
The replacive spinel harzburgite-dunite channels show sporadically interstitial and megacrystic clinopyroxenes and gabbroic veinlets that have been considered the early crystallization products of the melts migrating within the focused channels (e.g. Piccardo et al., 2007a). In some massifs (Erro-Tobbio, Mt. Maggiore), these magmatic clinopyroxenes show strongly fractionated LREE patterns and depleted incompatible trace element contents, suggesting crystallization from depleted melts fractions (Piccardo and Vissers, 2007; Piccardo and Guarnieri, 2010c) (Fig. 12).
In other massifs (e.g. South Lanzo) the magmatic clinopyroxenes from spinel dunite channels shows moderately fractionated LREE patterns that are more compatible with clinopyroxenes in equilibrium with aggregated N-MORB melts (Fig. 13) (Piccardo et al., 2007a). This evidence suggests a change in the melt dynamics after the early percolation of single melt fractions. The migration of aggregated MORBs within the replacive dunite channels indicate that the single melt fractions in the asthenosphere were more completely aggregated and mixed to form aggregated MORBs and that the dunite channels were exploited for delivery of the aggregated MORBs to shallow lithospheric levels. It can be argued that the aggregated MORB magmas passed through the mantle lithosphere migrating within these high porosity dunite channels, reached shallow lithospheric levels where they were intruded forming ephemeral gabbroic intrusions and were extruded at the sea-floor forming pillowed basaltic flows.
Figure 12. C1-normalized REE patterns of representative clinopyroxenes in distal replacive dunite channels of Erro-Tobbio (ET) and Mt. Maggiore (field 1) and calculated equilibrium liquids.
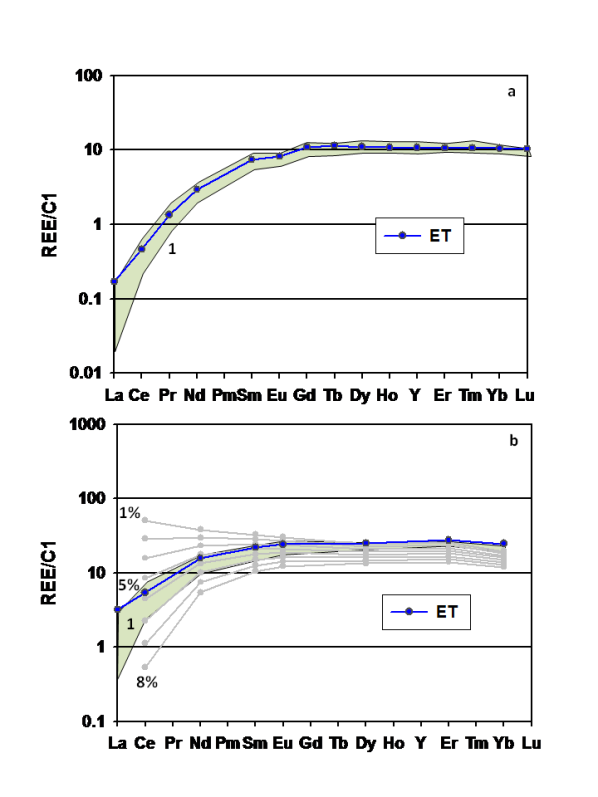
a) The distal replacive channels have magmatic interstitial clinopyroxenes showing strongly LREE fractionated C1-normalized REE patterns.
b) The liquids calculated in equilibrium with the representative clinopyroxene core compositions [using Cpx/liquid Kds of Hart and Dunn (1993)] are quite similar to those modelled for liquids formed by 4-7% fractional melting of spinel facies DM asthenospheric mantle source.
Figure 13. C1-normalized REE patterns of representative clinopyroxenes in distal replacive dunite channels of South Lanzo (field 2) and calculated equilibrium liquids.
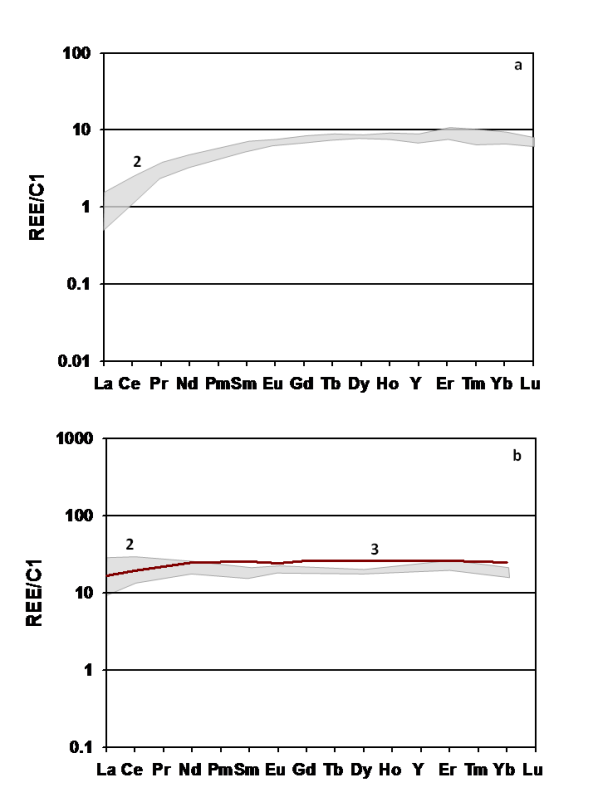
a) The distal replacive channels of South Lanzo have magmatic clinopyroxenes showing slightly LREE fractionated C1-normalized REE patterns (field 2).
b) The REE patterns of liquids calculated in equilibrium with the representative clinopyroxene compositions [using Cpx/liquid Kds of Hart and Dunn (1993)] (field 2) are quite similar to those of aggregated N-MORB melts. The REE pattern of N-MORB from Hofmann (1988) is also reported for comparison (3).