Our knowledge of the rheology of the Earth's crust and lithosphere is a major limiting factor to our ability to model geodynamical processes and to interpret geological structures. While laboratory experiments on rock deformation provide invaluable constraints on rock rheology [e.g., Kohlstedt et al., 1995; Gleason and Tullis 1995], extrapolating experimentally determined results to time and spaces scales relevant for geodynamical models is nontrivial and introduces many uncertainties [e.g., Paterson 2001]. In addition, rheological anisotropy is ubiquitous in nature [e.g., Cobbold 1976; Lister and Williams, 1983], but is extremely difficult to be tackled experimentally. Approaches other than laboratory experiments that can yield rock rheological information are thus very useful. These include studies of 1) geological structures like faults and shear zones [e.g., Snoke and Tullis 1998] and fold geometries [e.g., Hudleston and Lan 1995], and 2) seismological and neotectonic studies of currently active fault systems [e.g., Rutter et al., 2001]. In this paper we follow the first approach. We investigate the mechanics for the development of a group of related structures - kink-bands and kink folds, which are common in upper crustal rocks and explore the use of their occurrence to constrain some aspects of the rock rheology.
Kink-bands [1] are common structures in well-layered and foliated rocks as well as anisotropic crystals. They are tabular zones or lenticular domains (kind-band domains, KBD's), generally with sharp boundaries (kink-band boundaries, KBB's), within which the penetrative planar feature (foliation in a rock or cleavage in a crystal) is rotated relative to its orientation outside of the zone (Figure 1). Within the KBD, the foliation may remain more or less planar (Figure 1a, b, c, and d, "simple kink-bands" in this paper) or may show further kinks (Figure 1e, "composite kink-bands" in this paper). Kink-bands may occur in a single set (Figure 1a, b, c, and e) or as a conjugate pair (Figure 1d). The geometry of the latter has rendered them being variably referred to as box folds, conjugate folds, or conjugate kinks [e.g., Paterson and Weiss, 1962; Hobbs, et al., p.210-211; Ramsay and Huber, 1987, p.319, 409, 420].
Figure 1. Kink-bands in rocks
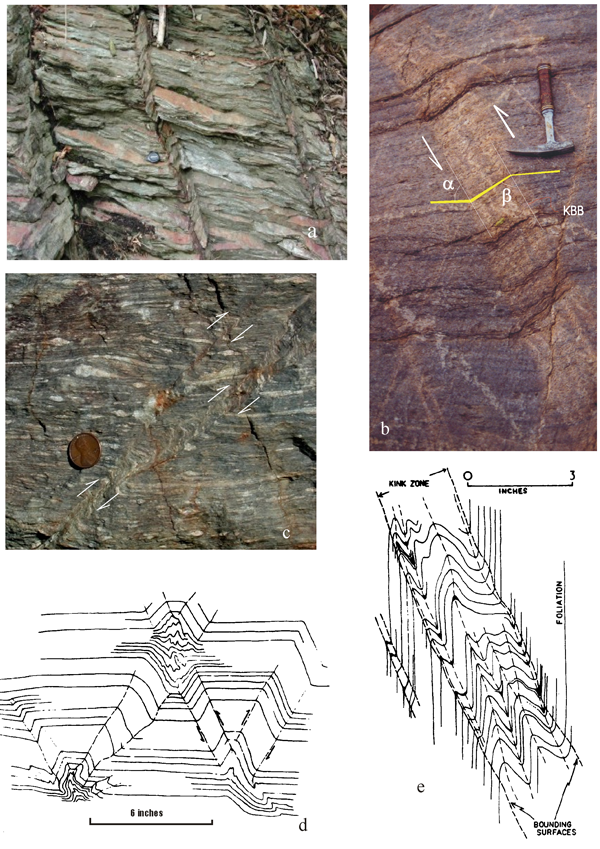
Kink-bands in rocks.
Kink-bands in metasiltstone at Harpers Ferry Maryland. Diameter of lens cap is 3cm.
Kink-bands in Huronian metasedimentary rocks, Espanola, Ontario.
Kink-bands in mylonite of the Garth Run shear zone in Madison County, Virginia. The mylonic C-foliation is cut by the kink-bands.
Conjugate kink-bands in Black Carboniferous slates on the southern side of the Beara Peninsula, Co. Cork, Ireland.
Composite kink-band at Creggan-a-tiadwar, central Murrisk, Co. Mayo, Eire. (d) and (e) are taken from Dewey (1965) with permission from Elsevier Science. See text for further discussion.
It is clear from laboratory experiments on slates and phyllites at room temperatures and various confining pressures [Paterson and Weiss 1962, 1966; Donath 1964, 1969; Anderson 1974] that, when subjected to foliation-parallel shortening, conjugate kink-bands will develop at relatively high confining pressures (e.g., > 300MPa, Anderson 1974), while fracturing occurs at lower confining pressures. The transition from kink-band development to fracturing may be also weakly strain-rate dependent according to experiments on salt-mica schist (W.D. Means per com). It is unclear how kink-bands originate and evolve. Paterson and Weiss (1962, 1966, 1968) proposed a kinematic model (Figure 2) whereby kink-bands start from a point or line source and grow in width by the KBB's migrating laterally into undeformed material. The process continues until conjugate kink-bands merge to form symmetric kinks and no undeformed regions are left in the material [see also Weiss 1980, Ramsay and Huber 1987, p.409]. Donath (1964, 1969) and Dewey (1965, 1969) maintain that KBB's are controlled by the principal stresses and are fixed to material particles after their initiation. The bounding KBB's of a kink-band separate until b = 90°
and then approach until b = a
(both angles are as defined in Figure 1b), when the kink-band "locks" and the mechanism is exhausted. Subsequent strain must be accommodated by development of new kink-bands or other mechanisms.
Figure 2. Kinematic model of Paterson and Weiss
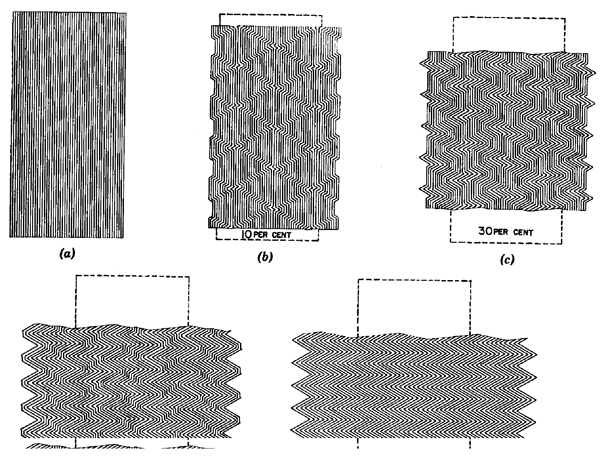
Kinematic model of Paterson and Weiss (1966) for kink-band development. Kink-band boundaries migrate until the whole rock is occupied by kinks. In this model kink-bands are earlier stage structures of kinks.
In the following we demonstrate with numerical finite difference modeling that the onset of kink-bands is a strain localization phenomenon necessarily controlled by rock rheology, stress state and deformation conditions, but the development of kink-bands after initiation is largely kinematic, with noticeable KBB migration and rotation. However, KBB migration does not consume the whole rock volume and is not a primary mechanism for the development of kink folds. Rather, kink-bands (strain localization) and kink folds (distributed strain) are produced by competing mechanisms governed by the degree of anisotropy of the rocks. Therefore we suggest 1) where kink-bands and/or kink folds are present the dominant rheological behavior at the time these structures were developed was likely plastic, and 2) the relative dominance of kink-bands and kink folds in a rock volume is an indicator of the degree of anisotropy of the rock.
[1] In this paper, we will use the term kink to describe any fold with straight limbs and angular hinge and use the term kink-band to describe a pair of kinks that include a short limb between two longer limbs. Typically the long limbs are at least an order of magnitude longer than the short limb. Formation of trains of kinks may involve similar foliation-parallel slip on alternating limbs, whereas foliation-parallel slip is probably restricted to, or is at least dominant in, the short limb in the formation of kink-bands. As we will demonstrate in this paper this genetic difference may be the fundamental difference between end member kink-bands (strain localization) and kinks (pervasive deformation). Geometrically there is a gradation from closely spaced kink-bands to asymmetrical kinks with limbs of similar length. Many natural examples of such kinks, from a genetic point of view, may be closely spaced kink-bands, which we have no way of telling. We therefore use the terms descriptively, restricting the term "kink-band" to the markedly asymmetric type, as described above, and to conjugate arrays of kink-bands which remain recognizable even when internal and external limbs approach similar length. All other morphologies are simply referred to as kinks.