Structural analysis of Martian bedrock
Exposure
Sands obscure the bedrock in the southeast half of the crater, where higher elevations suggest thick unconsolidated deposits. The greatest outcrop relief, around El Capitan and Big Bend is less than a half meter. Faceted rock surface morphology is typical of exposure by wind abrasion. There are minor gullies that might have been eroded by water but the alternative of wind erosion could not be ruled out (D. Marchant, pers. comm.) and there are no large channels entering or exiting the crater. We conclude that aeolian degradation of the crater rim was the probable cause in exposing bedrock in the northwestern quadrant of Eagle Crater (Figure 2a).
The morphology of Martian craters is dominated by local wind kinematics [Kuzmin et al. 2001, Toigo, A.D. & Richardson, M.I. 2003]. A unidirectional wind pattern heavily degrades the downwind rim of the crater while preserving most of the upwind rim morphology. Where two orthogonal wind directions dominate, an extensive expanse of crater rim is degraded. Atmospheric models predict a directional change of prevailing easterly winds in the Terra Meridiani region due to seasonal effects [Greeley & Thompson 2004]. Extensive and widespread erosion of the Eagle Crater rim is thus consistent with multiple wind directions, mostly from the southeast. Lesser secondary winds may be evidenced by the presence of transverse dunes observed outside the crater.
Bedding
There is evidence for right-way up bedding in the form of cross laminations as previously widely reported [Grotzinger 2004]. Small loose blocks may be inverted but there is no evidence of meso-scale overturning in outcrop. Rose diagrams of bedding traces are dominated by east-west trends consistent with the limits of rim outcrop (Figure 6a).
Big Bend, at the east end of the outcrop, appears to be kink- folded about an oblique axial plane (Figure 6b). The hinge region is not clearly visible and there may be a concealed fault in the corner of Big Bend. This may represent a minor perturbation of the impact stress field or it may mark a history of deformation involving more than just a single impact event. Judging by homoclinally dipping strata seen at Anatolia, well away from the crater rim, the tilting of sedimentary strata may be a regional phenomenon (Figure 7a), possibly marking the hanging wall of a thrust sheet involved in wrinkle ridge tectonics [Golombek et al 1991, 2001; Okubo & Schultz 2004].
Figure 6a. Rose Diagram of fracture trends
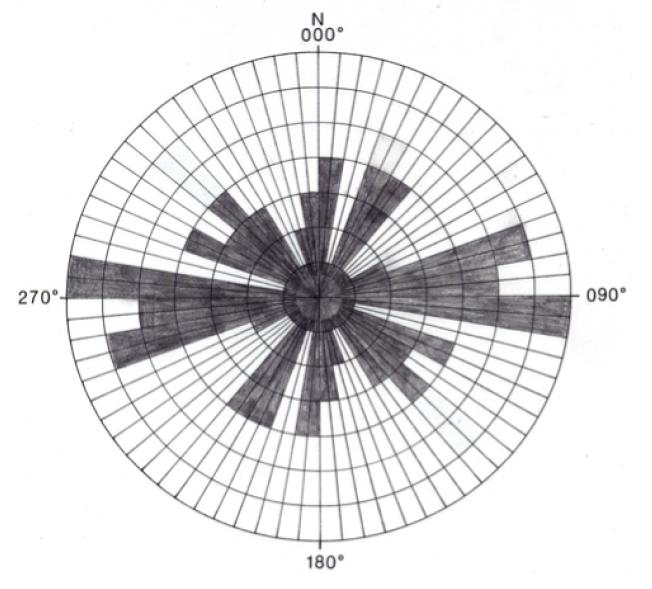
Rose Diagram of fracture trends. n=56. The main cluster is parallel to bedding. Two minor clusters are oblique to bedding.
Figure 6b. Kink folding of beds in the Big Bend
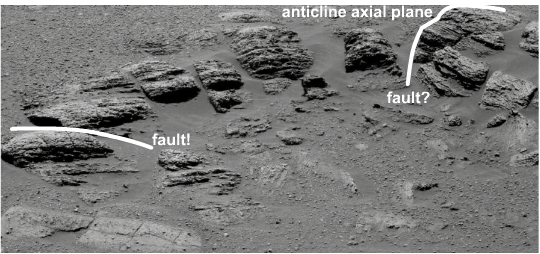
Kink folding of beds in the Big Bend. The core of the fold is poorly exposed and may be faulted. A strike slip fault or slump with pivotal motion is evident at the center left. True thickness of strata is less than 0.5 meter.
Figure 7b. Anatolia
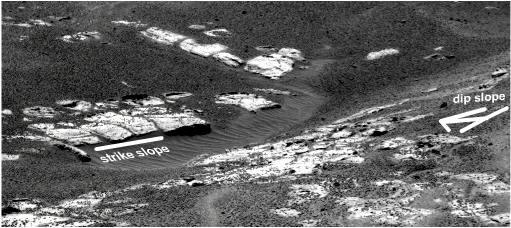
Homoclinally dipping strata at Anatolia may represent regional deformation. Bedding traces are exposed mainly as strike sections tangential to the crater rim. Outcrop morphology clearly indicates that the layers dip radially away from the crater rim in general, consistent with an origin by impact, rebound, and rim folding.
There are some parts of the western Eagle Crater rim, here called quasi-broken formation, where bedding traces are not readily discernible (Figure 8a). Apparently loose blocks dominate, but they do not form a jagged topography like that at Fram Crater (Figure 8b). Rather, rock surfaces are dominantly planar and the ground is notably even-paved, like a patio made of stonemason's "crazy pavement." (Unfortunately, we found no evidence of weeds between the paving slabs!) Many adjacent slabs can be fitted back together in jig-saw fashion by closing slightly gaping fractures. Bedding is interpreted to be sub-parallel to surface slope in some of these regions, as confirmed by close-up photography. Bedding in the quasi-broken formation is thus rotated relative to bedding in outcrop but minimally disrupted from one slab to the next. These rocks may represent extreme aeolian erosion [Williams, S. 2004] or an inner rim collapse structure, or may be the crestal exposure of the fold seen in profile at Big Bend.
Figure 8a. Quasi-broken formation
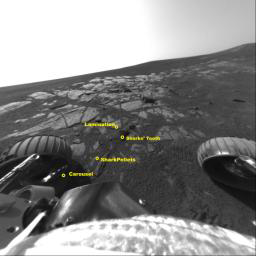
Quasi-broken formation. The ground is remarkably even-paved, like a stone patio. Neighboring slabs fit together in jigsaw fashion.
Faults and Fractures
Many individual sub-meter-scale rocks have inconsistent bedding orientations and are evidently not in place. Since these are clearly the same lithology as the outcrop and occur principally on the inner arc of the rim, they may represent impact-collapse structures. One prominent block at El Capitan has clearly pivoted inwards along a steep oblique fault.
Fracture patterns in the intact outcrop are dominated by relatively short, straight, meter-scale joints and are consistent with an impact origin [Ohman et al. 2003, Rodriguez et al. 2004]. There is no extensive development of conjugate fractures typical of horizontal tectonics on Earth. Fracture frequency in rose diagrams reflects the range of rim outcrop, with significant numbers of NNW orientations.
Fracture patterns in the quasi-broken formation are dominated by triangular slab shapes with many acute dihedral angles (Figure 8a). Small fractures often truncate against the sides of larger triangular slabs in a fractal-like arrangement. Triangular fracture patterns in the quasi-broken formations may be explained by the intersections of radial fractures sets (Figure 8c), by irregular chocolate tablet structure [Figure 4.11A, Ramsay & Huber 1983]. Chocolate tablet structure is possible if elastic rebound from the impact extended the crater rim in all directions. However, radial fracture sets seem to be the best explanation, given the thin atmosphere and propensity for impact tectonics on the Martian surface. There are also curved fracture patterns that resemble those formed by primary desiccation processes on Earth (Figure 8d, e). Desiccation cracks often have 90 degree joins as well as oblique ones and are generally curved.
Figure 8c. Radiating fracture patterns
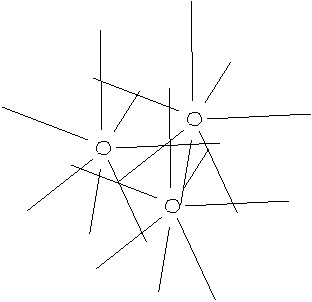
Formation of acute-angled triangular slabs by intersection of radiating fracture patterns.
Figure 8e. Desiccation cracks on Earth
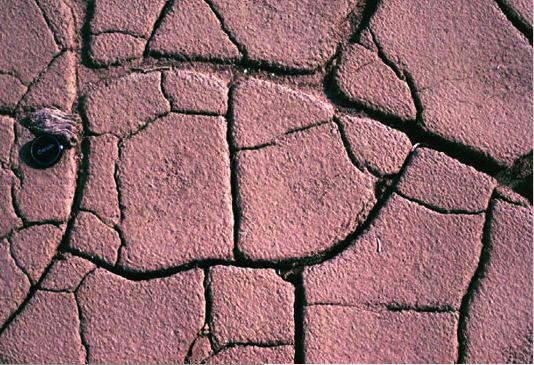
Desiccation cracks on Earth Death Valley for comparison, (photo: C. Simpson).
Fracture Cleavage
Several locations exhibit what appears to be a fracture cleavage - a closely spaced penetrative brittle fabric (Figure 9a). Fracture cleavage spacing is similar to the diameters of concentrically zoned spherules ("blueberry"), i.e. 4 to 8 mm on average, and fracture cleavage orientation, where visible, is at a high angle to bedding. Neither bedding nor fracture cleavage appear to wrap around the blueberries. However, some spherical blueberry outlines appear to be cut against cleavage planes, implying a tectonic, post diagenetic origin for the fabric. Some fracture fabrics weather proud, suggesting a possible mineral in-filling; however, photographic resolution is not sufficient to confirm this.
Crystal Vugs
Study of the orientations of Jarosite crystal casts [Squyres & Grotzinger 2004] reveals no evidence of compaction nor of penetrative finite strain post crystal growth (Figure 9b). Crystals are preferentially aligned at a high angle to bedding but this does not appear to be a strain fabric.
Striations
The surface Bounce Rock, a decimeter-scale loose rock located well outside of the crater rim, has a distinctly striated surface in photographs taken prior to specimen abrasion (Figure 9c). Care is needed in interpreting the specimen because, as its name implies, this rock was hit by the landing module's air bags. However, the striations appear to penetrate the rock and thus could not have been created simply by contact with the air bags. It is possible that the landing impact could have broken open a weak fracture but in that case, the other half of the specimen should be lying nearby on the surface, which it is not. We feel confident that the striations are of pre-mission origin - either due to meteorite impact or tectonic faulting. Striation geometry is consistent with a slickenside of fault origin, which may be mineralized or simply grooved. There are visible steps cross-cutting the striations as commonly described in fault-related slickensides. However, a slight fan-like striation geometry at the edge of the specimen may indicate that it is a shatter cone structure, i.e., a rapidly developed fracture splay formed when the rock was ejected from an impact site. If the specimen is indeed part of a shatter cone, then it should not differ significantly in composition from the rest of the rock. But if it is a mineralized slickenside, then it may have a very different chemistry to the bulk rock. This issue is significant given the importance attributed to chemical analyses at this location [Mullen 2004, Rayl 2004]. The greasy surface luster supports a meteoritic origin; meteorites commonly acquire a streaky surface sheen as they pass through the atmosphere.