PGE in ophiolite complexes of Greece
Characteristic features of ophiolite complexes
The ophiolitic complexes in Greece, of Middle to Late Jurassic age of obduction, form two major belts on each side of the Pelagonian zone: an east Pelagonian belt in the Vardarian domain and a Supra-Pelagonian ophiolitic belt to the west (Fig. 1). The emplacement of the ophiolite complexes in Greece from the Pindos–Mirdita Ocean, or from the Vardar Ocean or possibly from both of these oceanic basins is still ulclear. The different hypotheses, with respect to the origin of the ophiolites, present geodynamic evolution models accounting for most of the available data: a mid-Triassic episode of rifting; an early Jurassic episode of sea-floor spreading forming the Maliac Ocean; a Middle to Late Jurassic convergent period with subduction and obduction episodes, and finally, a late episode of Tertiary compressional deformation responsible for the thrusting of the Jurassic ophiolitic nappes over the external zones [Mercier 1966; Ferriere 1982; Smith et al. 1975; Rassios et al. 1994; Rassios and Smith 2000; Rassios and Moores 2006; Saccani et al. 2008 Rassios and Dilek 2009; Sassani et al.1990, 2003; Saccani et al. 2011; Robertson 2012]. Some authors provide stratigraphic and petrographic arguments supporting a double origin, (western and eastern) [Vergely 1976, 1984; Baumgartner 1985; Mountrakis 1987; Beccaluva et al. 2005]. On the basis of sedimentological data from the margin series and on structural analyses of ophiolitic masses there is strong evidence for the existence of two deep basins on both sides of the Pelagonian continental ridge during Triassic–Jurassic times, corresponding to the Vardar area to the east and the Pindos domain to the west [Ferriere et al. 2004].
Ultramafic lavas and high-Mg basalts
A salient feature of the Greek ophiolites is the spatial association of ultramafic lavas and boninites, which have been described in various localities of Greece, including the Othrys ophiolite complex [Smith et al. 1975; Cameron et al. 1979; Cameron and Nisbet 1982; Paraskevopoulos and Economou 1986; Economou-Eliopoulos and Paraskevopoulos 1989; Kostopoulos 1989; Capedri et al. 1997; Pe-Piper 1998; Pe-Piper et al. 2004; Barth et al. 2008; Barth and Gluhak 2009; Saccani et al. 2011; Koutsovitis et al. 2012]. At the Agrilia formation, highly magnesian lavas of komatiitic affinity occur in outcrops covering an area of at least 2 km 2. The rocks consist mainly of serpentinized or pseudomorphed olivine and skeletal clinopyroxenes with a random orientation in a matrix of fine-grained clinopyroxenes and devitrified glass (Fig. 2) [Paraskevopoulos and Economou 1986, 1997]. Although the studied lavas from the Agrilia Formation resemble boninites because their high Mg, Cr and Ni contents, they differ as regards their mineralogical composition and mineral chemistry. These lavas are dominated by olivine phenocrysts, are orthopyroxene free, and chromite is preferentially associated with groundmass.These ultramafic lavas are similar to Archean komatiites, in terms of their chemical composition [Arndt and Nisbet 1982]. Such rocks are very rare in the Phanerozoic; they are known at the Gorgona Island, Colombia [Gansser et al. 1979; Echeverria 1980] and the Agrilia formation. The rocks from Gorgona Island are considered to be the nearest Phanerozoic analogues of komatiitic basalts [Cameron and Nisbet, 1982].
Typical pillow lavas [Hynes 1972; Dijktra et al. 2001] dominate the western part of the Othrys opthiolite complex. Outcrop at the Pournari area occurs with the form of dyke, dominated by clipyroxenes, which sometimes are skeletal, at the contact with large phenocryst of orthopyroxene, clinopyroxene and olivine (Fig. 2). Euhendral to suhendral chromite is an accessory mineral, while clinopyroxenes with an acicular morphology, show a very impressive curved branching comb [Paraskevopoulos and Economou-Eliopoulos 1997].
Figure 2. Photomicrographs (2a, d) and back-scattered electron (BSE) images (2b,c) from ultramafic lavas from the Agrilia Formation (2a,b) and high-Mg basalts from the Pournari area (2c,d), Othrys ophiolite complex.
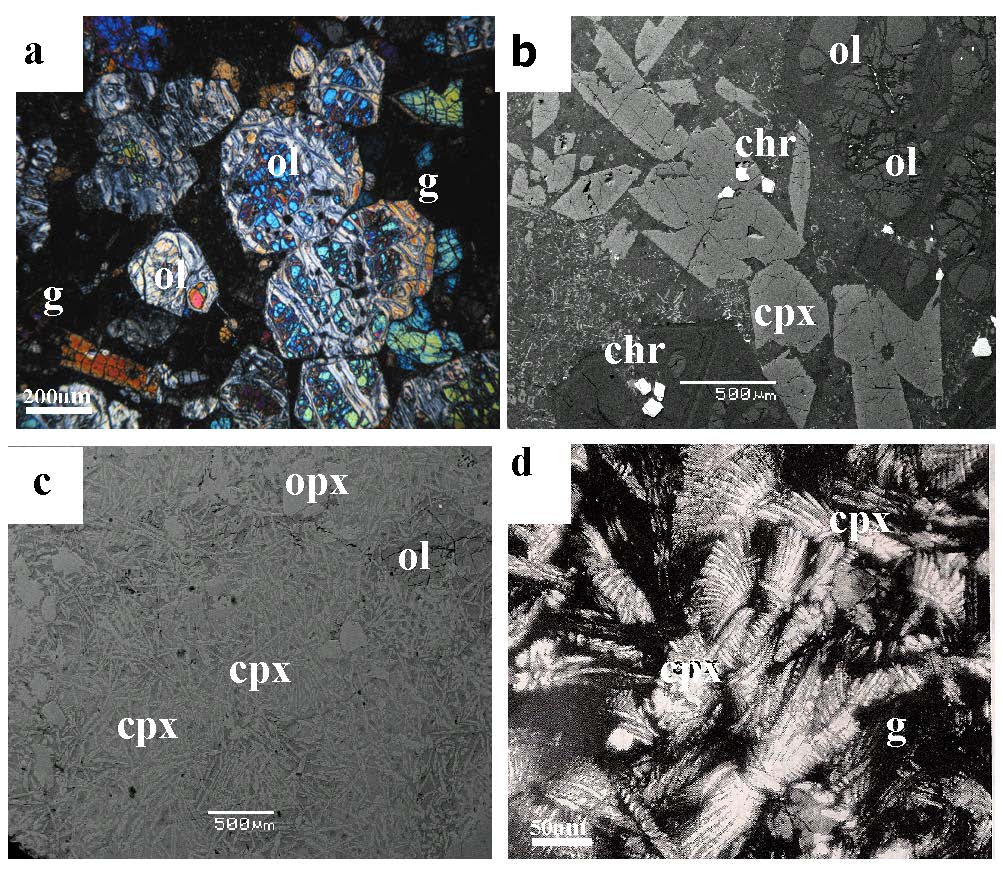
Partially serpentinized olivine phenocrysts (ol), clinopyroxene (cpx) and chromite in a devitrified glass (g) (2a viewed under cross Nichols); most chromite grains occur in the matrix, close to olivine or clinopyroxene phenocrysts (2b). The high-Mg basalts are dominated by acicular clinopyroxenes (2c) which often show the development of curved branching cockscomb forms (2d viewed under parallel nichols).
The liquid composition for the Agrilia lavas has been approached on the basis of the chemical composition of phenocrysts [Economou-Eliopoulos and Paraskevopoulos 1989] combined with experimental data of Fe-Mg partition between olivine and liquid [Bickle 1982]. Thus, using the distribution coefficient KD = [(MgO)/(FeO)]liquid [(FeO)/(MgO)]olivine, where KD = 0.314, for olivines (Fo9o-91) from the Agrilia ultramafic lavas, they concluded that they would be in equilibrium with liquids liquids containing 17 wt.% MgO, assuming no ferric iron in the liquid, and approximately 12.5 wt% MgO [Economou-Eliopoulos and Paraskevopoulos 1989]. As far as the Gorgona Islans, assuming that Fe2+/ΣFe = 0.90 for the Gorgona lavas the composition of the parental magma was calculated to contain 19% MgO and suggested that this would ctystallize olivine with Fo91.5, maximum observed (Herzberg et al. 2007].
It has been suggested that some lavas differentiate into an upper spinifex and a lower cumulate layer, as is exemplified by by changes in their mineralogical and chemical composition, such as an increase in modal olivine and chromite in the cumulate layers, and an increase in MgO, Ni and Cr [Arndt et al. 1977]. In addition, special attention was given to the platinum-group elements, which may provide evidence to assess the fractionation processes in komatiite lavas.
Geotectonic setting of ophiolites
Major ophiolite complexes
Major ophiolites, such as Pindos, Vourinos, Othris, Koziakas, Evia, Skyros, have been interpreted as parts of the same oceanic slab and differences of their geological and petrological data were attributed to intra-slab heterogeneity. MOR- and SSZ-type ophiolites (fore-arc and back-arc ridges) have been obducted, tectonically fragmented and separated by overlapping sediments of the Meso Hellenic trough. The occurrence of both styles of melting regimes within close spatial and temporal association in the same ophiolite has been explained by intra-oceanic thrusting and forced subduction initiation at (or near) a mid-ocean ridge [Rassios and Moores 2006; Barth et al. 2008].
The Vourinos complex represents an ophiolite, with supra-subduction characteristics and boninitic affinities. The Pindos complex contains a spectrum of lavas from MOR basalts through island arc tholeiites (IAT) to boninite series volcanics (BSV), and the Othris peridotites range from fertile plagioclase lherzolites (mid-ocean ridge setting) to depleted harzburgites (supra-subduction zone tectonic setting), as residua from variable degrees of partial melting [Smith et al. 1975; Pearce et al. 1984; Rassios and Moores 2006]. The harzburgites from the Othrys and the Vourinos ophiolite are highly depleted, as it is excemplified by the extremely low Al2O3 contents in pyroxenes [Economou-Eliopoulos 1996].
Ultramafic lavas and high-Mg basalts
Smith et al. [1975] concluded that in the Othrys complex there were two separate series: an earlier suite formed in a continental margin setting the Agrilia Formation, which was probably related to the opening of the ocean basin, and a later suite (the Mirna group) in which gabbroic cumulates and ultramafics were identified representing spreading in that basin. In general, high SiO2, H2O and unusually high abundances of some incompatible elements have been ascribed to complex mixing preceding boninite genesis [Sun and Nesbitt 1978; Cameron et al. 1979]. The enrichment in main incompatible elements (Sr, Rb, Ba, La), the Ti/V and Ti/Sc ratios, the pinel composition of the Agrilia lavas and the lower MgO content of the parent magma (~ 17 wt.% MgO) compared to that for boninites from Cape Vogel (20 wt.% MgO; Walker and Cameron, 1983), suggest a contribution to the magma composition by a subduction component. The Agrilia lavas have generated by a similar process like that of boninites [Crawford et al. 1981]. However, but probably somewhat further away from the trench (fore-arc setting), where the influence of the subducting slab is smaller and the partial melting degree lower compared to those of boninites [Economou-Eliopoulos and Paraskevopoulos 1989].
Methods of investigation
Polished sections were examined by reflected light microscopy and scanning electron microscope. Quantitative analyses were carried out at the University of Athens, Department of Geology, using a JEOL JSM 5600 scanning electron microscope, equipped with automated OXFORD ISIS 300 energy dispersive analysis system. Analytical conditions were 20 kV accelerating voltage, 0.5 nA beam current, 2 µm beam diameter and 50 second count times. The following X-ray lines were used: OsMα, PtMα, IrMβ, AuMα, AgLα, AsLα, FeKα, NiKα, CoKa, CuKα, CrKα, AlKα, TiKα, CaKα, SiKα, MnKα, MgKα, ClKα. Standards used were pure metals for the elements Os, Ir, Ru, Rh, Pt, Pd, Cu, Ni, Co and Cr, indium arsenide for As and pyrite for S and Fe.
Platinum-group elements (PGE) were determined by ICP/MS analysis after preconcentration using the nickel fire assay technique from large (30 g) samples, at Activation Laboratories, Ltd, Canada. This method allows for complete dissolution of chromitite. Detection limits are 5 ppb for Ru and Pt, 2 ppb for Os, Ir, Pd and 1 ppb for Rh and Au.
Characteristics of chromite deposits and occurrences
Compositional variation of chromite
The economic deposits in the Vourinos complex are either located in the uppermost part of the tectonized harzburgite within isolated dunite bodies or enclosed in narrow dunite envelopes. The chromite ore has commonly been affected by high-temperature deformation, superimposed on primary magmatic textures. Chromite ores of the South Vourinos area, including Xerolivado, one of the world's largest Alpine-type chromite deposits (about 6 million tons of ore) are remarkably homogeneous in major elements [Fig. 3; Economou et al. 1986]. Although the compositions of rest from North Vourinos differ significantly from those of South Vourinos, in particular the Kissavos area, at central part of the complex (Fig. 3) the compositional variation in all chromitites throughout the Vourinos complex is consistent with its suprasubduction zone environment [Konstantopoulou and Economou-Eliopoulos 1991; Grivas et al. 1993]. In addition, Dare et al. [2009] provide evidence using the plots of chromitites from the Vourinos ophiolite, on the Δlog fO2 versus Cr/(Cr+Al) diagram and Ti/Fe3+/(Fe3++Al+Cr) vs. Ga/(Fe3++Al+Cr) diagram, and concluded that they fall within the SSZ field.
The mantle sequence of the Pindos ophiolite complex resembles to that of Vourinos in the presence of extensive and highly depleted harzburgite. However, in contrast to Vourinos there are only a limited number of small chromite occurrences, and thus there is a low potential for exploitation in the Pindos complex [Rassios and Moores 2006]. Due to mantle and emplacement tectonics, a strong plastic and brittle deformation was superimposed on primary magmatic textures and had an important influence on the present form and distribution of chromitites [Rassios 1990]. Chromite composition of massive chromite show a wide variation of the Cr/(Cr+AI) ratio ranging between 0.47 and 0.83, while the Mg/(Mg + Fe2+) ratio ranges from 0.42 and 0.73 [Fig. 3; Economou-Eliopoulos and Vacondios 2005]. Recently, Pelletier et al. [2008] suggested that the low light-element contents of primary minerals and whole-rock samples argued against a supra-subduction zone (SSZ) origin of the Dramala mantle section, and against the previous hypothesis of hydrous melting of the Pindos mantle above a subduction zone. They concluded that the Dramala harzburgites represent a mid-ocean ridge (MOR)-type mantle, and not an SSZ-type mantle, juxtaposed with MOR-type and SSZ-type oceanic crust, either in a back-arc or in an intra-oceanic subduction zone setting.
The Othrys complex includes two tectonically separated chromite deposits, namely Eretria and Domokos, hosting about 3million tons of ore. Massive chromite ores occur as spherical, lenticular or irregular bodies of varying size. The host rock is moderately depleted harzburgite and no systematic relation exists between the distribution or size of the ore bodies to the size of host masses [Economou et al. 1986; Economou-Eliopoulos et al. 1997). A characteristic feature is a remarkably homogeneous composition type (Fig. 3). The majority of chromite ores are high-Al (of refractory type), although ores with a relatively high-Cr content were also found in a small proportion. They differ from those in the Vourinos complex, which are dominated by high-Cr (metallulgical type) deposits. However, at the central part of the Vourinos complex (Kissavos area) the composition of small chromite occurrences ranges from high-Al to high-Cr, both types being in a comparable proportion (Fig. 3).
Ophiolite masses, mostly serpentinized dunite and harzburgite, in the western margin of the Axios zone, including the areas of Edessa, Vermio, Veria, E. Thessaly (Fig. 1) contain mainly massive chromite bodies of small size. The chromite deposits of W. Chalkidiki peninsula and Serbomacedonian massif occur in altered dunite and serpentinized peridotites [Economou 1986].
Figure 3. Plot of Cr/(Cr+Al) vs Mg/(Mg+Fe2+), showing the compositional variation of chromite from the main ophiolite comples of Greece. Data from Economou-Eliopoulos [1996].
The petrological, mineralogical and geochemical characteristis of the Veria ophiolite are typical of ophiolites associated with a SSZ geotectonic environment [Economou et al. 1986]. The main lithologic type making up the Veria ophiolite is a highly melt-depleted mantle harzburgite. Mantle shear zones, several hundreds of meters in width, dominate the mantle sequence of the Veria ophiolite, which are characterized by the presence of fish-shaped serpentinite bodies. Small lens-shaped bodies of massive chromitite are common along zones of shearing. More than 100 isolated chromite occurrences, elongated lenses, following the NW-SE trend of the host serpentinized dunite. They are small (5 to 15 m in length, 0.3 to 1 m in thickness), mostly massive and dense disseminated bodies. The majority of the massive chromitites occur as irregular bodies of varying size. Several small chromitite bodies are confined to mylonite-shear zones within the Veria ophiolites. They have been affected by intense tectonic activity, which has created faulting, folding, over thrusting, foliation and lineation of minerals [Koukouzas and Kouvelos 1973]. Chromite is dominantly of high-Cr composition.
The compositions of chromitites from the entire Veria area fall within both high-Cr and -Al types according to Koukouzas and Kouvelos [1973]. Detailed investigation of chromitites from the area of Galaktos (Veria) showed a dominance of the high-Cr variety in both PGE-poor and PGE-enriched chromitites [Tsoupas and Economou-Eliopoulos 2008]. The alteration of chromite to ferrian chromite at grain peripheries and along cracks is characterized by a progressive enrichment in the Fetot and Mn contents, and a correspondingly strong depletion in Mg and Al compared to chromite cores (Table 1).
Figure Table 1. Representative microprobe analyses of chromite from massive chromitites of the Veria (Galaktos) area
All silicate minerals occurring in interstices between chromite grains have been entirely altered to Fe-poor serpentine (2 to 4 wt.% FeO), and Cr-free chlorite (5 to 11 wt.% FeO). Chromitites from mylonitic shear zones of the Galaktos area (Fig. 1) are characterized by abundant Cr-andradite and uvarovite [Ca3(Fe,Cr)2(SiO4)3], and lesser amounts of Fe-poor Cr-chlorite and serpentine flakes as interstitial secondary minerals. The most salient feature of certain garnet-bearing chromitites is the presence of abundant PGM assemblages (see below), whose occurrence seems to be independent of the chemical composition of the host chromite mineralization. Chromite hosting PGM and chromite lacking PGM both fall within the same compositional range, although the former tends to show a strong brittle (cataclastic) and mylonitic deformation [Tsoupas and Economou-Eliopoulos 2008].
Inclusions in chromite ores
Primary inclusions in fresh chromite consisting of Ni-Fe-Cu sulfides (pentlandite, pyrrhotite, chalcopyrite, bornite), silicates (olivine, pyroxenes) and the PGM have been documated at the Vourinos chromitites [Garuti and Zaccarini 1997]. Also, a close association of the PGM sulfides with enstatite, Na-rich pargasite, clinopyroxene, phlogopite, and Cu-Ni sulfides have been described at the Othrys chromite ores [Garuti et al. 1999].
The cbromite locally shows pull-apart textures and brecciation, and is often transformed into ferrian chromite along the rims and cracks. Secondary opaque-mineral assemblages consisting of milerite, heazlewoodite, Ni-Fe alloys and native copper, occur in the ferrian chromite alteration zones and/or in the silicate matrix, between chromite grains [Tarkian et al. 1992; Garuti and Zaccarini 1997; Tsoupas and Economou-Eliopoulos 2008]. Inclusions of pentlandite, pyrrhotite, chalcopyrite, less abundant PGE and rarely native iron have been described in chromite ores from the Skyros Island. The silicate matrix is entirely serpentinized and carries numerous grains of heazlewoodite and millerite. Tarkian et al. [1992] indicated that all silicate minerals including inclusions in chromite, magnetite and the matrix of both, have entirely been altered to chlorite and serpentine, and that chlorine, up to 0.34 wt% Cl were detected in the later hydrosilicates. Magnetite ore associated with chromitite has shown a different sulphide assemblage consisting of pentlandite, pyrrhotite, pyrite, Cu-Fe- and Cu sulphides, graphite, tetraauricupride (AuCu) and sperrylite [Tarkian et al. 1992].
Although the majority of the chromite ores is characterized by paucity in fluid inclusions, abundant fluid inclusions were found in chromite hosted by a coarse-grained pyroxenite dike at the Pindos complex. Chromite [average Cr/(Cr+Al) ratio = 0.73] occurs in highly variable proportion in an orthopyroxene matrix or as inclusions in orthopyroxenes, exhibites primary and secondary fluid inclusions. The primary inclusions are of following types: Three-phase solid–liquid–gas, or gas fluid inclusions (4A), which reach up to 50 μm in size. The solid minerals inside the inclusions are idiomorphic in shape and large size. Three-phase liquid–liquid–vapour or gas fluid inclusions (Fig. 4B), with elongated shapes; the size of bubbles is very small and range from 20 to 30 μm. The secondary fluid inclusions are two-phase liquid–gas and mono-phase fluid inclusions. The presence of fluid inclusions in chromite aggregates hosted in orthopyroxenite dikes, in combination with the trace element contents in chromite concentrates and the mineralogical composition of the dikes may indicate that an aqueous phase separated from the magma [Al-Boghdady and Economou-Eliopoulos 2005].
Figure 4. Primary fluid inclusions in chromite.
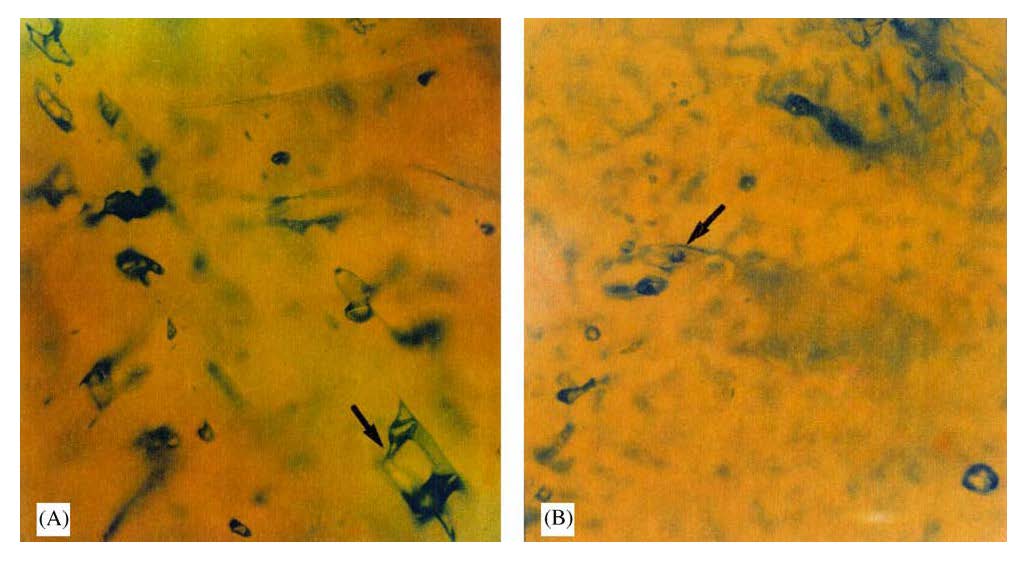
Three-phase solid–liquid–gas fluid inclusions (A), three-phase liquid–liquid–gas fluid inclusions (B) [after Bogdady and Economou-Eliopoulos 2005].
Platinum-group element contents and PGE mineralization
PGE in ultramafic lavas and high-Mg basalts
The PGE concentrations in the ultramafic lavas from the Agrilia Formation and high-Mg basalts are low. Average platinum-group element (PGE) concentrations (all in ppb) are: Os 4, Ir 0.7, Ru 7, Pt 5, Pd 18, Rh < 0.5 and Au 15 [Economou-Eliopoulos and Paraskevopoulos 1989]. The low content of PGE, the higher Pd content compared to that of Pt and the lack of detectable Rh appear to be characteristic of the Agrilia ultramafic lavas [Economou-Eliopoulos and Paraskevopoulos 1989]. In a comparison of noble metal concentrations and PGE patterns made on the studied lavas from Agrilia Formation with those of highly magnesian rocks, there appear similarities to those found in komatiites from Munro Township, Ontario [Crocket and MacRae, 1986].
The crystallization history of Munro Township indicates that the main chemical trends are generated by fractional crystallization of olivine. Pd content in the Agrilia lavas falls within the range of values found in Munro Township komatiites, but Pt is very low. Pd and Pt are preferentially partitioned into the melt, so that these elements are concentrated in spinifex-textured upper flow units in the komatiites [Keays 1982; Crocket and MacRae 1986]. Ir content of the Agrilia lavas is very low (average 0.68±0.08 ppb). Keays [1995] found that olivine in dunitic komatiites at Kambalda, Western Australia, contain the bulk of the Ir and suggest that during differentiation of a mafic melt Ir coprecipitates as Ir-Os alloy with the first silicate (usually olivine) or oxide phases to settle from the magma. The role of oxides (chromite) in the Agrilia ultramafic lavas appears to be minimal; there is not any positive correlation of Ir with Cr, indicating an important control of chromite fractionation on Ir concentration (Table II). Given the compatible nature of Ir [Crocket and MacRae 1986], the low Ir content in the Agrilia lavas may reflect either Ir removal from the magma, during crystal fractionation in deep crustal magma conduits, prior to lava formation or, if we assume that the Agrilia melts moved rapidly from the source to the surface before significant crystallization, then it may reflect a relatively low degree of partial melting. PGE patterns for primitive rock types (komatiites) are relatively flat, whereas more evolved rock types exhibit fractioned PGE patterns. Further, an increasing Pd/Ir ratio is the characteristic trend to be expected in the normal crystallization of mafic magma [Barnes et al. 1985]. The values ( ~ 12) of the Pd/Ir ratios for the Agrilia lavas are close to those for typical komatiites (5-10), and their PGE patterns which are almost flat, also resemble komatiites. The slightly higher Pd/Ir ratios of Agrilia lavas compared to komatiites may be related to magma fractionation, and/or a relatively low degree of partial melting, or enrichment in Pd by an alteration process. However, a higher degree of fractionation of the magma would be expected to produce enrichment in Pt and probably Rh as well [Barnes et al. 1985]. In addition, there is abundant evidence to suggest modification of Au and Pd during varying types of metamorphism [Keays 1982; Barnes et al. 1985]. Thus, hydrothermal alteration and/or serpentinization, which have affected those rocks and reactions of hydrating fluids with olivine (and pyroxene) to form alteration products may have inhibited the complexing and transport of elements as Pd and Au. Therefore, although an enrichment of Pd could be due to such an alteration, the relatively low Pd/Ir ratios and PGE patterns being flat, with exception of a negative Pt anomaly, may indicate that the studied rocks from Agrilia represent relatively primitive magmas.
Platinum-group minerals (PGM) in the Agrilia lavas were not identified. In general in komatiites have been described PGM of only small size (18 - 7 μm), which are predominantly sperrylite (PtAs2), sudburyite (PdSb) and merenskyite (PdTe2) [Nesbitt et al. 1979; Keays 1995, Herzberg 1995; Barnes and Maier 1999; Maier et al. 2002]. The paucity of PGM in komatiites seem to be consistent with the experimental data by Brenan and Andrews [2001] suggesting that PGM crystallization from basaltic magma may be feasible, but it is possible that the extrusion temperature of komatiites exceeds the maximum thermal stability of the PGM. For example, laurite has been shown to be stable up to the temperature of 1275 oC in mafic magmas [Brenan and Andrews 2001].
PGE in chromite deposits and occurrences
Since chromite is the main PGE collector in ophiolite complexes the PGE distribution in chromitites associated with ophiolite complexes of Greece have become an interest topic for many authors. Platinum-group element (PGE) content in large chromite deposits is generally low (a few hundreds of ppb). However, a PGE-enrichment has been recorted in: (a) in all PGE, (b) only in Os, Ir and Ru or (c) in Pt and/or Pd, relatively small chromite occurrences, of both high-Cr and high-Al type, in the uppermost parts of the mantle and/or in the lowest crust sequence of Greek ophiolites. Examples of PGE-enrichment in Greece include the ophiolites of Pindos, (up to 7 ppm total PGE), [Economou-Eliopoulos and Vaconndios 1995, Tarkian et al. 1996, Economou-Eliopoulos et al. 1999; Prichard et al. 2008; Grammatikopoulos et al. 2009], the Skyros island in the Aegean (up to 3 ppm), the Veria (up to 25 ppm) [Table 2; Tsoupas and Economou-Eliopoulos 2005, 2008] and Vourinos (up to 3 ppm) [Konstantopoulou and Economou-Eliopoulos 1991].
More specifically, the PGE total for most of the chromitite samples from the Vourinos complex are less than 200 ppb. However, in a small portion (about 15% of the analysed samples) from the northern and central parts of the complex there unusually high concentrations for one or more PGE (Table 3). The PGE distribution is heterogeneous, the total ranging from 53 to 3,030 ppb. The majority of the analyzed samples from North Vourinos show Ru >> (Os+Ir) in contrast to the southern part of the complex, where Ru < (Os + Ir). In addition, Pd/Ir and (Pt + Pd)/(Os + Ir + Ru) ratios are much higher in the northern than in the southern part [Konstantopoulou and Economou-Eliopoulos 1991].
The majority of chromite samples show a relatively high Ir, Os and Ru contents, while Pt and Pd are low resulting in chondrite (C2)-normalized PGE patterns with a negative slope (Fig. 5) and a low Pd/Ir ratio. In contrast, some chromitite samples from the Pindos complex, which are Rh, Pt and Pd enriched whilst Ir, Os and Ru contents are low, show smooth sloped to positive slope PGE-patterns (Fig. 5). Moreover, chromitites with a similar major-element composition may exhibit a variation in the PGE-patterns and the values of the Pd/Ir ratios.
The PGE content in chromite ores from the Othrys ophiolite complex is low, while the chondrite normalized PGE-patterns are characterized by a negative slope, which are similar to those for large chromite deposits associated with ophiolite complexes [Economou-Eliopoulos et al. 1997].
Figure 5. Chondrite-normalized PGE-patterns.
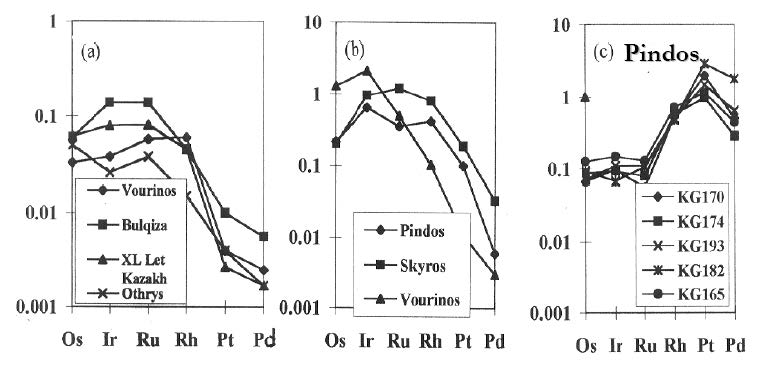
Chondrite-normalized PGE-patterns (sample/C1 chondrite) for chromite ores hosted in ophiolite complexes from the Vourinos and other large chromite deposits (a), IPGE-enriched ores (b) and PPGE-enriched ores. Data from Tables 2 and 4; Tarkian et al. [1996]; Economou-Eliopoulos et al. [1997].
Recently, high concentrations of PGE (mostly compatible IPGE), have been determined in chromitites hosted in the Veria ophiolites, northern Greece. Whole ore PGE concentrations in massive chromitites range from < 100 ppb to high PGE concentrations, reaching 25 ppm ΣPGE (Os = 7,400, Ir = 6,020, Ru = 9,700, Rh = 310, Pt = 760, Pd = 750, all in ppb; Table 4). The high PGE content, and the presence of extremely abundant PGM grains concentrated along a shear zone of strongly brecciated chromite ore associated with garnet was initially described by Tsoupas and Economou-Eliopoulos [2005, 2008].
A negative slope characterizes the chondrite-normalized PGE patterns, for both PGE-depleted and PGE–rich chromitites (Fig. 6). In a comparison of the latter with other PGE-enriched ophiolite-related chromitites, it is clear that the enrichment of refractory PGE in the Veria chomitites in is unique.
Figure 6. Chondrite-normalized PGE-patterns (sample/C1 chondrite) for the Veria chromite ores and PGE-enriched chromitites related to other ophiolite complexes.
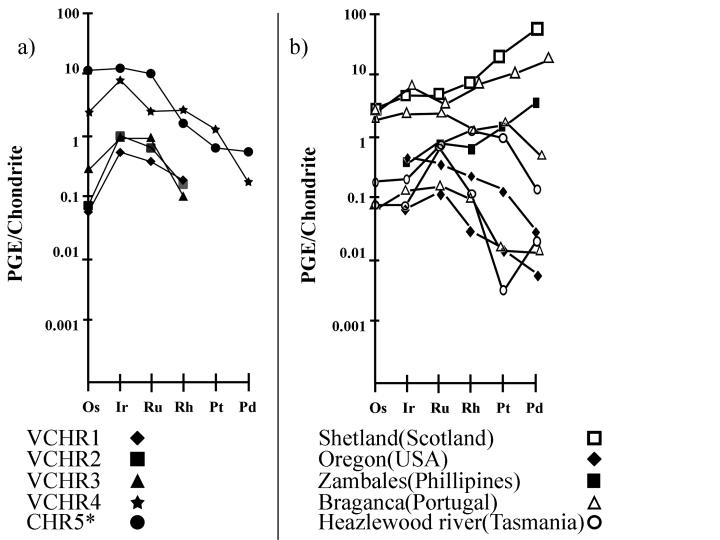
Data from Table 4; Stockman et al. [1984] for Heazlewood River, (Tasmania); Bacuta et al. [1990] for Zambales (Phillipines); Peck and Keays [1990] for Oregon (USA); Tarkian and Prichard [1987] for Shetland (Scotland); Bridges et al. [1993] for Braganca (Portugal). Normalization values (C1) after Naldrett and Duke [1980].].
Platinum-group minerals in Greek ophiolites
Several authors have recorded the presence of platinum-group minerals (PGM) in the chromite deposits of Greece. Laurite, (Ru,Os,Ir)S2, constitutes more than 75% of the platinum group mineral (PGM) population, accounting for the Ru-Os-Ir dominated composition of the chromitite host [Augé 1986; Tarkian et al. 1992, 1996, Garuti and Zaccarini 1997, Garuti et al. 1999, Tsoupas and Economou-Eliopoulos 2005, 2008; Kapsiotis et al. 2006; Prichard et al. 2008; Grammatikopoulos et al. 2009].
The existences of primary PGM (laurite, irarsite, Os-Ir alloys) as inclusions in fresh chrromite [Auge 1985, 1988; Auge and Johan 1988], as well as PGM assemblages suggesting origin at low temperature, by desulfurization of pre-existing PGM sulfides [Garuti and Zaccarini 1997; Kaptiotis et al. 2006; Grammatikopoulos et al. 2009] have been well documented.
Platinum-group minerals (PGM) have been identified in chromitite from the Pindos ophiolite complex. The PGM consist of Os-rich laurite, enclosed in chromite, sperrylite, eclusively in the serpentinized matrix of chromitite, and an unidentified phase, Pt(Ni,Fe)3 [Tarkian et al. 1996]. Also, at the Milia area, have been identified Os, Ir, Ru (IPGE) alloys and sulfarsenides, while the Korydallos area is dominated by Pt- and Pd-bearing PGM including Pt–Pd alloys, PGM arsenides and sulfarsenides, sperrylite and members of hollingworthite – irarsite – platarsite solid-solution series [Prichard et al. 2008]. In addition, Ru-rich alloy grains in chromitites from the Milia area, with relatively high Rh and Pt abundances, have been described by Grammatikopoulos et al. [2009].
PGM identified as inclusions in chromitites of the Othrys ophiolite complex were Os-rich laurite and erlichmanite [Garuti et al. 1999]. They can be classified into two subgroups: the more Os-, Ir- and Ru-rich or IPGE (Ir-goup) and Pt, Pd-rich or PPGE (Pt-group) assemblages. The more Pt- and Pd-rich assemblages (Pd–Pt, Pd–Pt-alloys, Pt-arsenides, most likely sperrylite) occur interstitial to chromite grains. The Othrys is similar to that of chromitites located in the mantle unit of other ophiolite complexes of the Balkan peninsula (Vourinos, Skyros Island, Rhodope) in terms of the predominance of Ru-Os-Ir phases, although it is distinguished because of the Os-rich composition of laurite and the presence of erlichmanite. In addition, the significance variation on the Os/Ru ratios, hich has been attributed to the fractionation between these two elements [Garuti et al. 1999].
At the area of Veria, laurite inclusions within unaltered chromite grains, and large PGM grains, ranging from 0.8 mm to 1.3 mm in size (Fig. 7) were located in PGE-enriched chromitite. Also, extremely abundant solitary fragmented PGE-minerals (more than 100 grains) within sheared massive chromite samples were identified (Fig. 8). At the contact between strongly fragmented chromite and coarse-grained chromite PGM grains are dispersed in a trail up 3 mm in length [Tsoupas and Economou-Eliopoulos 2008]. The PGM fragments may be angular, rarely corroded grains or fine-grained aggregates occurring in a matrix of cataclastic chromite cemented by Cr garnet.
Figure 7. Back-scattered electron (BSE) images of extremely large PGM grain, composed by Or-Ir-Ru-oxides and remnants of laurite-irarsite assemblages.
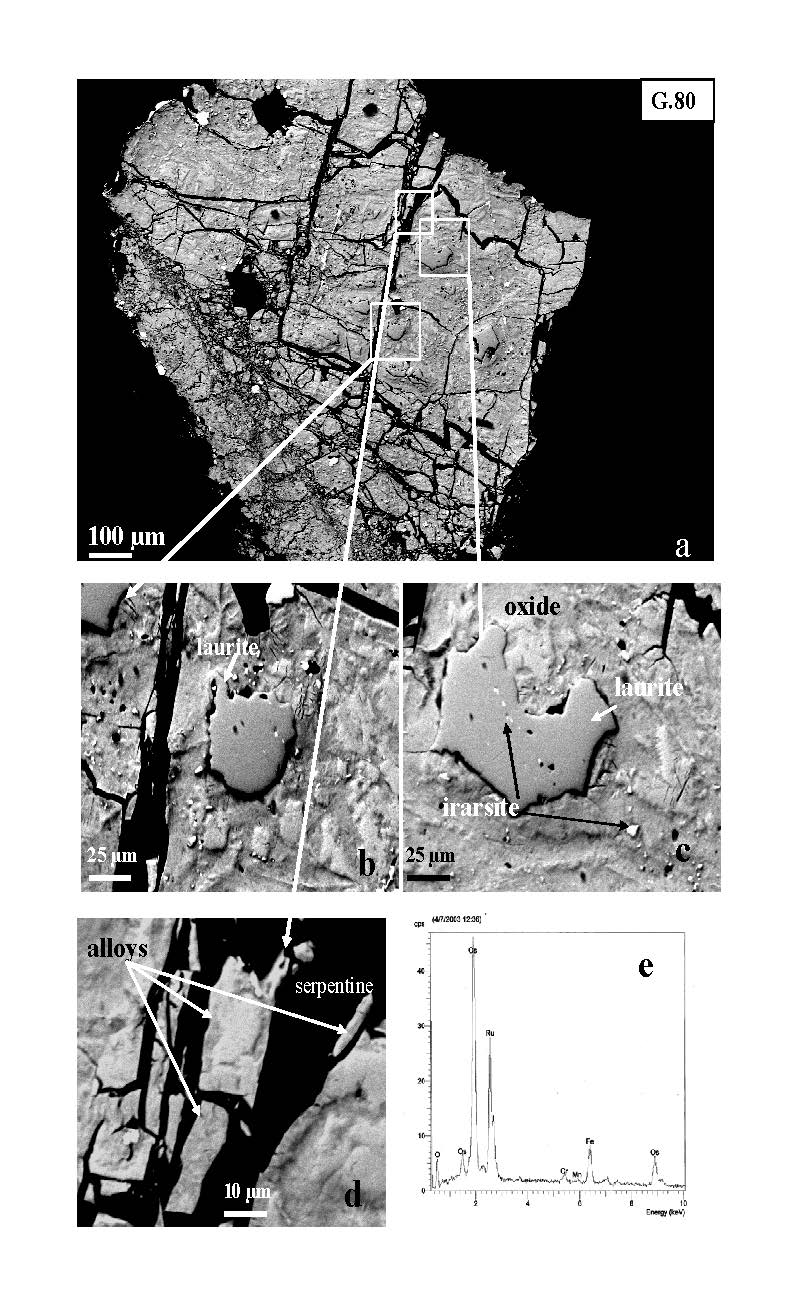
It was found at the contact between strongly fragmented chromite in a matrix of garnet (and less amount of serpentine), and coarse-grained chromite. That large PGM grain has probably conserved most of its initial form, and avoided the dislocation along the movement direction, although strongly fragmented. (e) The X-ray spectrum shows the presence of oxygen [from Tsoupas and Economou-Eliopoulos 2008].
Figure 8. Back-scattered electron (BSE) images of extremely abundant dismembered-fragmented IPGM grains zone.
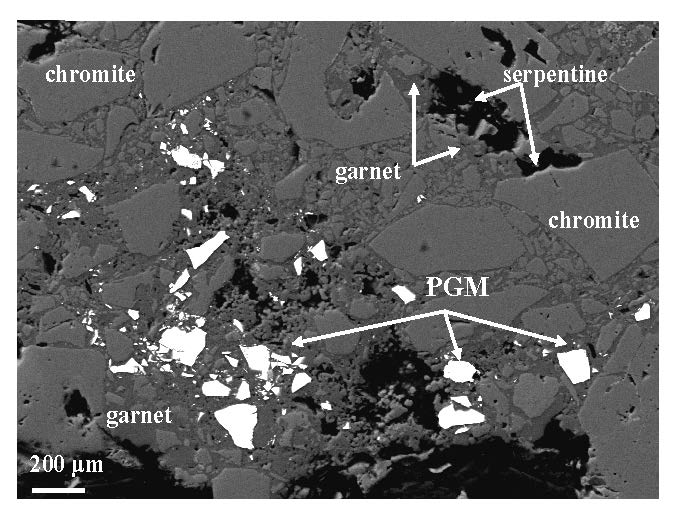
Back-scattered electron (BSE) images of extremely abundant dismembered-fragmented IPGM grains zone, associated with strongly fragmented chromite as well, in a matrix of garnet and lesser amounts of serpentine/chlorite. They are found along a highly shearing zone from the Galaktos area.
Laurite (RuS2) was identified as small inclusions within unaltered chromite grains in the Galaktos chromitites, but it mostly occurs as relicts containing very small irregular grains of irarsite within a matrix of Ru-Os-Ir alloy/oxide (Fig. 7), exhibiting a wide compositional variation (Table 5a). Irarsite, displaying varying form and composition, is also a major PGM. It is associated with laurite in the large PGM grain and as intergrowths with platarsite-hollingworthite and garnet [Tsoupas and Economou-Eliopoulos 2008]. Pt-Pd-Rh-minerals occur commonly as relatively fine-grained assemblages (ranging from <2 to 50 μm) with irarsite and other minerals, and show a wide compositional variation (Table 5b). Pt-alloys show a variation ranging from tetraferroplatinum, with low amounts of other elements, to Pt-Ir-Fe-Ni alloys. Both Pt-alloys and Pt-sulfarsenides are predominant Pt-minerals, whereas Pd-analogues are both very fine-grained and rare. Rhodium-arsenides, close to the composition of hollingworthite, are the commonest Rh-minerals, although sulfarsenides are also present (Table 5b). These compounds form fine grains associated with Pt-Pd-Ni-Fe-arsenides and –alloys (platarsite, vasilite) within a silicate matrix dominated by garnet; they also form intergrowths with garnet. Rh-arsenides are characterized by a strong enrichment in Ni, and can be represented by the general composition RhNiAs (Table 5b). Awaruite (Ni3Fe), containing small amounts of Co and Cr, heazlewoodite (Ni3S2), pentlandite and maucherite commonly occur within fractures and the serpentine-chlorite matrix.
Figure Table 5a. Representative microprobe analyses of IPGM from PGE-rich chromitites of the Veria (Galaktos) area
Figure Table 5b. Representative microprobe analyses of Ph-minerals from PGE-rich chromitites of the Galaktos area
Thus, mineral-chemistry data indicate that the sulfur content decreases gradually outwards the periphery or cracks of IPGM grains in chromite ores, of Greece and hence the replacement of laurite by Os–Ir–Ru alloys has been well documented [Garuti and Zaccarini 1997; Tsoupas and Economou-Eliopoulos 2005, 2008; Kaptiotis et al. 2006, 2009; Prichard et al. 2008; Grammatikopoulos et al. 2009]. The enrichment in Ir, Pt, Pd, Fe, Ni, Co and Mn, and/or metallic phases, such as metallic Ru, has been interpreted as a replacement association, characterized by in situ desulfurisation products of the PGM sulfides, at relatively low (350–500oC) temperature [Stockman and Hlava 1984; Tarkian and Prichard 1988; McElduff and Stumpfl 1990; Garuti and Zaccarini 1997; Melcher et al. 1997; Ahmed et al. 2003; Tsoupas and Economou-Eliopoulos 2008].
Phase equilibrium constraints on synthetic PGE-minerals
The stability limits and mineral – sulfide melt partitioning behavior of the platinum-group minerals laurite (Ru,Os,Ir)S 2 and Ru–Os–Ir alloy, as functions of temperature and sulfur fugacity have been well enstablished. More specifically, experimental data have demonstrated that under appropriate conditions of T, P, fO2 and fS2, the precipitation of laurite from basaltic liquids is feasible, laurite can be formed in equilibrium with Os–Ir alloys and it is stable up to the temperature 1275oC (< 1300 oC) in mafic magmas [Brenan and Andrews 2001; Andrews and Brenan 2002]. Also, they found that laurite and alloy were Ru–Os–Ir-rich and Pt–Pd-poor, with grains of alloy containing more Os and Ir than laurite, and both phases becoming (Os + Ir)-rich with increasing f(S 2). Bochrath et al. [2004] showed that laurite may be the first sulfide mineral to crystallize directly out of a sulfide-undersaturated silicate melt, under conditions more oxidized than Δf O2=FMQ−1.5, which applies to most terrestrial melts. A melt that is more reduced than this value would exsolve a sulfide liquid at the f S2 in equilibrium with RuS 2, and hence would fractionate all noble metals into sulfide melt, destabilizing discrete PGE sulfides. Initially, laurite is nearly stoichiometric (RuS 2) with very low contents of Os and Ir. As the temperature decreases and f S2 increases Os is progressively incorporated into the laurite lattice, due to the partition coefficient of Os between laurite and silicate melt, and it significantly increases with decreasing temperature [Andrews and Brenan 2002].
Implications for PGE and chromite mineralization
Despite, the compositional variation of laurite and IPGE-alloys, hosting in chromite ores associated with ophiolites, they are similar in terms of (a) their precipitation directly out of a sulfide-undersaturated basaltic melt at temperatures higher than 1000oC (lower than 1275oC), (b) their small (< 25 μm) grain size and (c) small whole PGE content in the chromite ore, and their formation is well constrained [Ohnenstetter et al. 1999; Garuti et al. 1999; Matveev and Ballhaus 2002; Cabri 2002; Bockrath et al. 2004]. Liquidus, falling within the two-phase field defined by high-f(S2) experiments [Andrews and Brenan 2002] suggest high-f(S2) conditions during their crystallization or that the laurite formed at a lower temperature (1010-1030oC). A close association of the PGM sulfides with enstatite, Na-rich pargasite, clinopyroxene, phlogopite, and Cu-Ni sulfides provide evidence for crystallization at high temperature, under relatively high sulfur fugacity. The Othrys is similar to that of chromitites located in the mantle unit of other ophiolite complexes of the Balkan Peninsula (Vourinos, Skyros Island, Rhodope) in terms of the predominance of Ru-Os-Ir phases, although it is distinguished because of the Os-rich composition of laurite and the presence of erlichmanite. The lower temperature in this case seems to be consistent with the association of hydrous minerals reflecting an increased HO content in the crystallized magma [Matveev and Ballhaus 2002].
The members of the irarsite-hollingworthite solid-solution series and other Os-,Ir, Ru- and Rh-bearing PGM in chromitites from some ophiolite complexes, and their association with altered chromitites may indicate either in situ alteration or/and re-mobilization and re-deposition of PGE during at least two stages [Tarkian and Prichard 1987]. The presence of Ru-Os-Ir-Fe-oxides, with significant and variable Fe, Cr, Co, Ni and Mn contents, associated with serpentine, chlorite, Cr-garnet, ferrian-chromite and magnetite in chromite ores, suggest that they may have been derived from in situ alteration of primary PGM, by desulfurization and subsequently oxidation of preexisting sulfides of Os–Ru–Ir [Garuti and Zaccarini 1997]. Apart from the well documantated replacement of PGM by desulfurisation products,at relatively low temperature [Stockman and Hlava 1984; Tarkian and Prichard 1987; McElduff and Stumpfl 1990; Garuti and Zaccarini 1997; Melcher et al. 1997; Tsoupas and Economou-Eliopoulos 2005, 2008; Kaptiotis et al. 2006; Prichard et al. 2008; Grammatikopoulos et al. 2009], primary PGE mineralization may provide valuable evidence for the PGE and chromite mineralization.
The geotectonic environments at which noble metals are mobile or become concentrated are considered to be critical for understanding the PGE mineralization and developing strategies for prospecting [Naldrett 1980, Campbell et al. 2010]. The association of laurite with hydrous fluids in immiscible hydrous liquids, the simultaneous precipitation of PGE alloys, laurite, olivine, and chromite consistent with a supra subduction zone (SSZ) geotectonic environment related to chromite deposits hosted in ophiolite complexes [Matveev and Ballhaus 2002]. The experimental data are consistent with the formation of chromite deposits in ophiolite complexes, only in the presence of water-rich magmas saturated in olivine and chromite, in a supra subduction zone (SSZ) environment [Bacuta et al. 1990; Zhou and Robinson 1997; Ohnenstetter et al. 1999; Economou-Eliopoulos et al. 1999; Gervilla et al. 2005]. Although, due to the high solubilities of the PGE in basaltic magma, direct nucleation and crystallization of PGM from silicate melt would be precluded, the majority of the authors agree that laurite and alloys occurring as inclusions within natural chromite crystals may have formed by direct crystallization from a silicate magma under appropriate conditions of T, P, fO2 and fS2 [Tredoux et al. 1995; Matveev and Ballhaus 2002; Bockrath et al. 2004]. Matveev and Ballhaus [2002] on the basis of experimental data, found that the liquidus temperature of chromite decreased with increasing H2O content (4 wt%). With lower water content (1–2 wt% H2O) higher temperatures (1430–1480 oC) at 1.5 GPa have been reported. Also, upon olivine and chromite precipitation, chromite would be collected in the hydrous liquid droplets, accompanied by PGE-rich metallic alloys, while olivine was collected in the basaltic melt [Matveev and Ballhaus 2002]. The PGE distribution in chromite ores, associated with layered intrusion and ophiolites complexes, differs as far as their PGE-type: chromites in the former, such as the UG2 horizon of the Bushveld complex, showing a PPGE-enrichment relative to IPGE, are characterized by a high fractionation of PGE [Naldrett 2004]. In contrast, PPGE contents in chromitites hosted in ophiolite complexes of Greece and elsewhere PPGE are commonly much lower than IPGE, in particular in large chromite deposits [Economou-Eliopoulos 1996]. Although a relationship between sulfur and PGE content is unclear, due probably to the desulfurisation of primary PGE-minerals, the presence of sulfur in the parent magma seems to be a major controlling factor for the PGE-concentration and deposition, in particular in PGE-enriched chromitites. Extremenly high PPGE-enrichment in certain chromite occurrences of Greece (Tables 2, 3 and 4), and elsewhere, like Shetland, UK [Prichard et al. 1986; Tarkian and Prichard 1987, Prichard et al. 1994], Bulqiza complex, in Albania [Ohnenstetter et al. 1999], Troodos in Cyprus [Prichard and Lord 1990], New Caledonia [Augé et al. 1998], the Zambales ophiolite complex in the Philippines [Bacuta et al. 1990] and in Thetford mines in Quebec [Corrivaux and Laflamme 1990], are located mostly at the uppermost parts of the mantle and/or the lowest parts of the crust sequence (towards the petrological moho). The occurring enrichment in Pt and Pd may be the result of the sulfur saturation and PPGE deposition in relatively small chromite occurrences, in contrast to large chromite deposits in ophiolite complexes, where parent magmas are PGE diluted [Prichard et al. 2008]. The mineralogical associations of Pt with Ni, and Pd with Cu in the Pindos samples, suggest that the Pt and Pd were collected by the small fraction of base-metal-rich immiscible sulfide liquid and that the Pt collected in the Fe–Ni-rich phase and the Pd with Cu-rich one. The presence of only a small amount of immiscible sulfide liquid led to very high concentrations of Pt and Pd within the sulfide liquid and to the presence of abundant Pt- and Pd-bearing PGM associated with the rare base-metal sulfides [Prichard et al. 2008]. Therefore, the PPGE enrichment can be considered as a sensitive fingerprint for the orientation in the mantle sequence of the ophiolite complexes and hence the explotation for chomite deposits [Economou-Eliopoulos 1996].
The enrichment in IPGE at Milia is a common feature shown also by the Skyros and Veria chromitite, although the levels of the IPGE exhibit a wide variation [Tsoupas and Economou-Eliopoulos 2005]. Chromitites at Milia, with average Cr/(Cr+Al) ratio 0.83 may derived from magma produced by a high degree of mantle melting [Prichard et al. 2008]. However, the Cr/(Cr+Al) ratio at the Skyros chromite ores is relatively low (average 0.52) and at Veria it exhibits a wide range (0.5- to 0.85) in a small same, even in the same thin section (Table 1). The wide variation, in both IPGE and PPGE of high-Cr and high-Al ores, through small scale regions (like Pindos, Skyros and Veria) and the highly heterogeneous Os isotopic composition at Cuba [Gervila et al. 2005], may suggest different episodes of partial melting, subduction-related crustal recycling and metasomatism [Ahmed and Arai 2003; Griffin et al. 2003; Walker et al. 2005; Pearson et al. 2007]. Although there is a general acceptance of both the primary magmatic nature of the IPGE-enriched minerals hosted in chromitite and of remobilization of PGE at relatively low temperature during the postmagmatic stage, the relative timing of postmagmatic events and the alteration of the PGM is still uncertain. The IPGE enrichment at Veria, reaching 25 ppm total PGE and resulting in an abundance of PGM grains, may be related to postmagmatic processes covering a long period of deformation, beginning with plastic deformation in the asthenospheric mantle and culminating with brittle deformation in the crust [Tsoupas and Economou-Eliopoulos 2005, 2008]. Among major factors controlling such a wide variabily, may be difference as fas far as: (a) the magmatic sources of the parent magma, (MOR, SSZ or arc regions) during the evolution of the geotectonic environment (b) melt–rock reactions during upwelling basalting magmas and progressive fractionation of parent magmas [Leblanc 1995; Economou-Eliopoulos 1996; Melcher et al. 1997; Zhou and Robinson 1997; Ahmed and Arai 2003]. The presence of both high-Cr and high-Al chromitites, which may be PGE-poor and PGE enriched in the Pindos complex [Economou-Eliopoulos and Vacondios 2005] combined with the stable Ga/Fe3++Al+Cr) ratio (~1000) for those ores suggest that magmatic source and fractionation are major factors of their compositional variation rather than melt –rock reactions [Dare et al. 2009].
With respect the origin of coarse-grained platinum-group minerals, reaching values up to a few mm, which have been located in many placer deposits associated with ultramafic-mafic complexes, such as Alaskan and ophiolitic type intrusions is a subject of debate, due to the paucity of coarse-grained PGM in parental mafic-ultramafic complexes. Some authors have suggested that coarse-grained PGM have formed at low-temperature, during the weathering and sedimentation [Augustithis 1965; Bowles et al. 2000]. Others suggested that coarse-grained PGM may have crystallized in late magmatic pegmatitic environments in the mafic/ultramafic complexes [Cabri and Harris 1975; Johan et al. 1990; Johan 2000; Malitch et al. 2003; Hattori et al. 2004; Tolstykh et al. 2005]. In particular, based on the paragenesis of IPGE with silicates it has been suggested that large IPGM-phases, with a grain-size ranging from 400 to 1000 μm, have been formed at temperature from 700 to 850 oC [Johan et al. 1991, 2000; Melcher et al. 1997; Tolstykh et al. 2005; Ahmed 2007]. In the case of the extremely large IPGM (1300 μm) at Veria, located exclusively along shear zones of chromitites (Figure 6), it has been suggested that IPGM and accompanying chromite may have re-crystallized during plastic deformation episodes at relatively lower temperatures (800 to 900oC).
Evaluation of PGEs as an economic factor in ophiolite complexes
The geotectonic environments at which noble metals become concentrated are considered to be critical for understanding the PGE mineralization and developing strategies for the chromite and PGEs exploration. The PGE distribution in chromite ores associated with layered intrusion and ophiolites complexes differs as far as the PPGE-enrichment relative to IPGE in the former, suggesting a high fractionation of PGE [Naldrett 2004], in contrast to large chromitites hosted in ophiolite complexes of Greece and elsewhere showing a low ΣPGE content and negligible IPGEs [Economou-Eliopoulos 1996]. The presence of an extremely high PPGE-enrichment in the small chromite occurrences, located towards the petrological moho, such as in Pindos and elsewhere [Prichard et al. 1986; Tarkian and Prichard 1987; Prichard and Lord 1990; Prichard et al. 1994; Tarkian et al. 1996; Ohnenstetter et al. 1999], suggest the presence of only a small amount of immiscible sulfide liquid resulting to very high concentrations of Pt and Pd [Prichard et al. 2008], and can be considered as a sensitive fingerprint for the orientation in the mantle sequence of the ophiolite complexes and hence the exploration for chomite deposits [Economou-Eliopoulos 1996].
The enrichment in IPGE such as the Pindos (Milia), Skyros and Veria in small chromite occurrences has been attributed to different episodes of partial melting, subduction-related crustal recycling and metasomatism [Ahmed and Arai 2003; Griffin et al. 2003; Walker et al. 2005; Pearson et al. 2007]. In particular, the IPGE enrichment at Veria (up to 25 ppm) may be related to postmagmatic processes covering a long period of deformation, at relatively lower temperatures (800 to 900oC), beginning with plastic deformation in the asthenospheric mantle and culminating with brittle deformation in the crust along the permeable shear zones, under appropriate pressure, temperature, redox conditions [Tsoupas and Economou-Eliopoulos 2008]. Thus, the most targeting for PGE locations general in ophiolite compexes may be are (a) for the chromitite-IPGM associations exclusively small chromite occurrences along shearing zones of ophiolite complexes, postdating their initial/magmatic PGE deposition, and (b) for PPGE the uppermost parts of the mantle and the lowest crust sequence.
Transport of PGE in hydrothermal systems
The mobilization of PGE by processes involving an aqueous fluid has been well documented. The current state of knowledge of solubility of PGE has been reviewed and applied toward a better understanding of the PGE mineralization in hydrothermal systems [Gammons et al. 1992; Wood 2002; Wilde et al. 2002]. The stable oxidation stages of Pt and Pd in aqueous solution under the most oxidizing conditions are Pt(II) and Pd(II). The Pt and Pd are quite soluble under a range of hydrothermal conditions (as chloride or hydroxyl species), in hot oxidized brines (as chlorides), and in hot, reduced, sulfur-rich waters (as bisulfide omplexes). On the basis of experimental data, it has been concluded that the highest Pt and Pd concentration is attained under both oxidized and acidic conditions and that chloride is the most abundant ligand in hydrothermal solutions, forming strong complexes with Pt and Pd, and it is a potentially important ligand for aqueous transport of these metals [Gammons et al. 1992, Wood 2002]. Also, elements like As, Bi, Sb, Se and Te form very insoluble compounds with PGE.
In hydrothermally altered harzburgite of the Semail ophiolite of Oman, elevated Pt (up to 450 ppb) is associated with silicified and carbonate altered serpentinite (listwaenite), which occurs along Tertiary extensional faults [Wilde et al.2003]. Also, significant concentrations of PGE (few ppm Pd and Pt) were found in magmatic breccia at the contact between the Bangur intrusion and its ultramafic host, Baula-Nuasahi Complex, India. Based on mineralogical and geochemical data, including oxygen, hydrogen and sulfur isotopes, it has been suggested that the precious metals in the hydrothermal fluids were derived from the magma rather than an external source [Augé and Lerouge 2004]. Platinum and Pd contents in sea-floor massive sulfides and sulfides of Fe-Ni-Cu-Co type in ultramafic parts of ophiolite complexes are very limited [Economou and Naldrett, 1985; Foose et al., 1985). However, elevated contents (up to 1 wt.% Pt) in marcasite and chalcopyrite from East Pacific Rise, 1000 ppb Pd in sulfide deposits on the mid-Atlantic, and 160 to 1000 ppb Pt in disseminated pyrite and chalcopyrite from brecciated pipeform diabase, underlying the massive ore of the Pindos ophiolite complex (Greece) have been recorded [Economou-Eliopoulos 2005 and references therein].
Characteristics features of the sulfide ores in the Pindos ophiolite complex
A salient feature of the Pindos massive ore from Kondro is the significant contents of Au (up to 3.6 ppm), Ag (up to 56 ppm), high Se contents (up to 1900 ppm), Co (up to 2200 ppm), Mo (up to 310 ppm), Hg (up to 280 ppm) and As up to 150 ppm (Table 6). The ore is mainly comprised of pyrite and chalcopyrite. Bornite sphalerite, marcasite, covellite and siegenite are found in lesser amounts. Textural relationships indicate that early pyrite, commonly occurring as fractured, large crystals, is extensively replaced by chalcopyrite, and then by bornite in a matrix of quartz. Cu-bearing sphalerite, with up to 3.6 wt.% Fe and 3.2 wt.% Cu, cements minor chalcopyrite and pyrite. Pyrite is extensively replaced by intergrowths between chalcopyrite and Fe-poor sphalerite with an average Cu content of 0.5 wt.%. The latter association, found in cross-cutting veins, hosts selenides (chiefly clausthalite, PbSe), the telluride mineral melonite (NiTe2), gold, galena and barite. Concentrations of ‘invisible Au’ in coarse-grained pyrite ranges from 0.7±0.13 to 1.01±0.27 ppm, whereas concentrations in fine-grained pyrite lie between 7.67±1.6 and 5.3±2.7 ppm, accompanied by significant As content [Economou-Eliopoulos et al. 2008]. The Pd content in breccia samples (10 to 27 ppb) is low as compared to that of Pt (160 to 1000 ppb). A significant enrichment in Pt in kaolinite-rich brecciated diabase underlying the Kondro massive ore, and the limited range of δ34S values for pyrite concentrates from the diabase breccia (+1.0 to +1.5‰), are characteristic features [Sideris et al. 1984].
Genetic significance of the platinum and palladium contents
Elevated Pt contents in the mineralized diabase breccia of Pindos compared to massive sulfide ore seems to confirm the higher solubility, and hence further transport, of Au relative to Pt, causing Au enrichment in the massive ore [Pan and Wood 1994]. Thus, assuming that Au and Pt/Pd were derived from the same source area, and that the transporting complexes of these metals are the same [Wood 2002] the concentration of Au in massive sulfide ore and Pt in the underlying diabase breccia may reflect higher solubility of Au that remains more soluble than Pt, and the extent to which these metals are saturated in a given hydrothermal solution. The increasing trend of average Zn, Au, Ag, As, Se, Sb, Mo and Hg contents from the Pindos is similar to that at Mirdita (Albania). They are considered to be [Economou-Eliopoulos et al. 2008] the closest analogues to polymetallic sulfides associated with felsic volcanic rocks at the modern seafloor, and that their formation has taken place during the initial stages of subduction (fore-arc setting).