Evolution of the MHB and successive geodynamic settings
This synthesis (Figs. 20 and 21) is mainly based on our work about the MHB but also considers the results obtained from other studies in the basin [e.g. Brunn, 1956; Desprairies, 1979; Ori and Roveri, 1987; Barbieri, 1992; Doutsos et al., 1994; Kontopoulos et al., 1999; Zelilidis et al., 2002; Vamvaka et al., 2006].
Pre-MHB setting
Before the first MHB transgressive deposits, late Lutetian in age, the pre-MHB domain belongs to the internal zones that have been intensively deformed after the deposition of Paleocene flysch [e.g. Celet and Ferrière, 1976]. This major tectonic episode of the Hellenides is characterized by mainly southwest-verging folding and thrusting. The structural initiation of the Pelagonian Indentor, that is necessarly prior to MHB development, can be attributed to this event.
In the inner domains of the internal zones, this Tertiary episode of deformation is also characterized by intense metamorphism. In the outer domain, there is no significant tertiary metamorphism as the Late Cretaceous limestones are not metamorphosed. The important metamorphism of the Pelagonian zone in this area is late Jurassic in age, associated with the emplacement of ophiolitic nappes during obduction [e.g. Ferrière et al. 2012].
Lutetian to Late Eocene small confined basins
The first sedimentation areas in the MHB domain are restricted to two small confined basins: The Rizoma sub-basin resting on Pelagonian basement and Krania sub-basin resting on ophiolitic units (Fig. 20A).
The Krania sub-basin presents evidences for synsedimentary tectonic activity, such as internal angular unconformities, flysch facies with many olistostromes and large olistolites, and numerous slump events indicating instabilities on slopes. The northern boundary of the Krania sub-basin is clearly an active flexure that controls the sedimentation within the area. There is no evidence for an eventual connection between the two sedimentation areas of Krania and Rizoma, although it cannot be totally excluded.
Figure 20. Detail of the MHB evolution from Pindos Subduction (late Eocene) to Collision (Oligocene – middle Miocene)

Six geologic stages are represented with, for each of them, a map of the MHB and a cross-section in the southern part of this basin. Vertical and horizontal scales are similar. See text for more explanations. Schematic maps and cross-sections illustrate the successive stages of evolution of the MHB in the framework of the Hellenic Tertiary subduction-collision of external zones under the internal ones.
Maps. Oblique black arrows: direction of sedimentary flows; + = uplift areas; - = subsiding areas. Cross-sections. Vertical black arrows: areas of major uplift and of main subsidence ; white arrows: direction of sedimentary flows.
Figure 20A. Late Lutetian-late Eocene
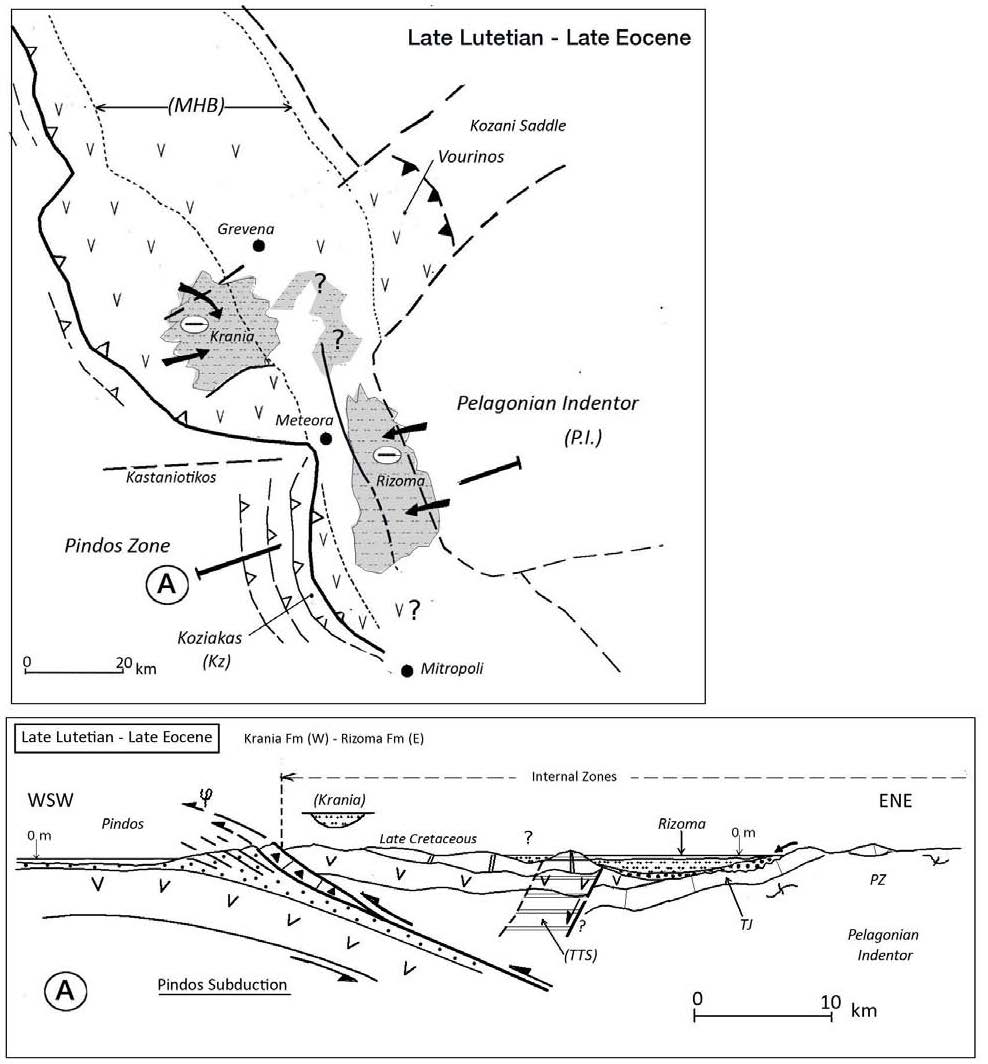
The Krania-Rizoma subsiding areas are linked to the Pindos subduction. They developed in the fore-arc domain just on the east of the Pindos accretionary prism. The location of these two basins is linked to the Pelagonian Indentor structure. Sediments are rich in elements coming from the close areas (ophiolites for the Krania basin, Paleozoic basement for the Rizoma basin).
These small basins are contemporary with the late Eocene development of the large thrust driving the internal zones over the external zones, that is to say the Pindos Zone. This is confirmed by the younger flysch deposits in the Pindos Zone that are Lutetian in age, even up to Late Eocene [Desprairies, 1979].
Therefore, the sedimentation in Krania and Rizoma sub-basins takes place during the onset of convergence and related Pindos subduction, responsible for the development of an accretionary prism below the Pelagonian upper unit that has been covered by ophiolitic nappes during Jurassic obduction [e.g. Ferrière et al., 2012]. Because it originated at the back of a rising accretionary prism, the deep sub-basin of Krania and the sub-basin of Rizoma may be considered as forearc basins on an active margin.
The pattern of subsidence, at that time, should be driven by mechanical parameters of the subducting crust (thickness, temperature, angle of the subducting slab). The boundaries of Krania and Rizoma sub-basins are controlled by heterogeneities of the upper crustal unit such as flexures at the borders of the Pelagonian Indentor (Rizoma basin on the western side and Kozani- Krania saddle on the NW side of this Indentor) (Fig. 20A).
Latest Eocene compressional episode
The Eocene-Oligocene transition is outlined by a major angular unconformity, particularly important nearby the large tectonic structures (e.g. Monachiti area, Fig. 14; Ft in Theopetra area, Fig. 16A ).
This is a period of significant compressional deformation attested by the development of eastward verging reverse faults, and of folds associated locally with slight cleavage (e.g. in Krania-Mylia and Theopetra-Avra areas). It corresponds to the end of the late Eocene tectonic phase.
We consider that this major compressional event is the consequence of the arrival in the subduction zone of the Gavrovo-Tripolitsa platform unit (Fig. 20B). This is consistent with the continuing rise of the Pindos accretionary prism, the exhumation of the main flysch tectonic windows below the ophiolitic nappes (Filippi Anticline, Af, Fig. 20B and Fig. 20C), and the emersion of the Krania and Rizoma sub-basins as evidenced by the occurrence of reddish continental conglomerates at the base of Oligocene series on top of the unconformity.
Figure 20B. Latest Eocene (till boundary with Oligocene)
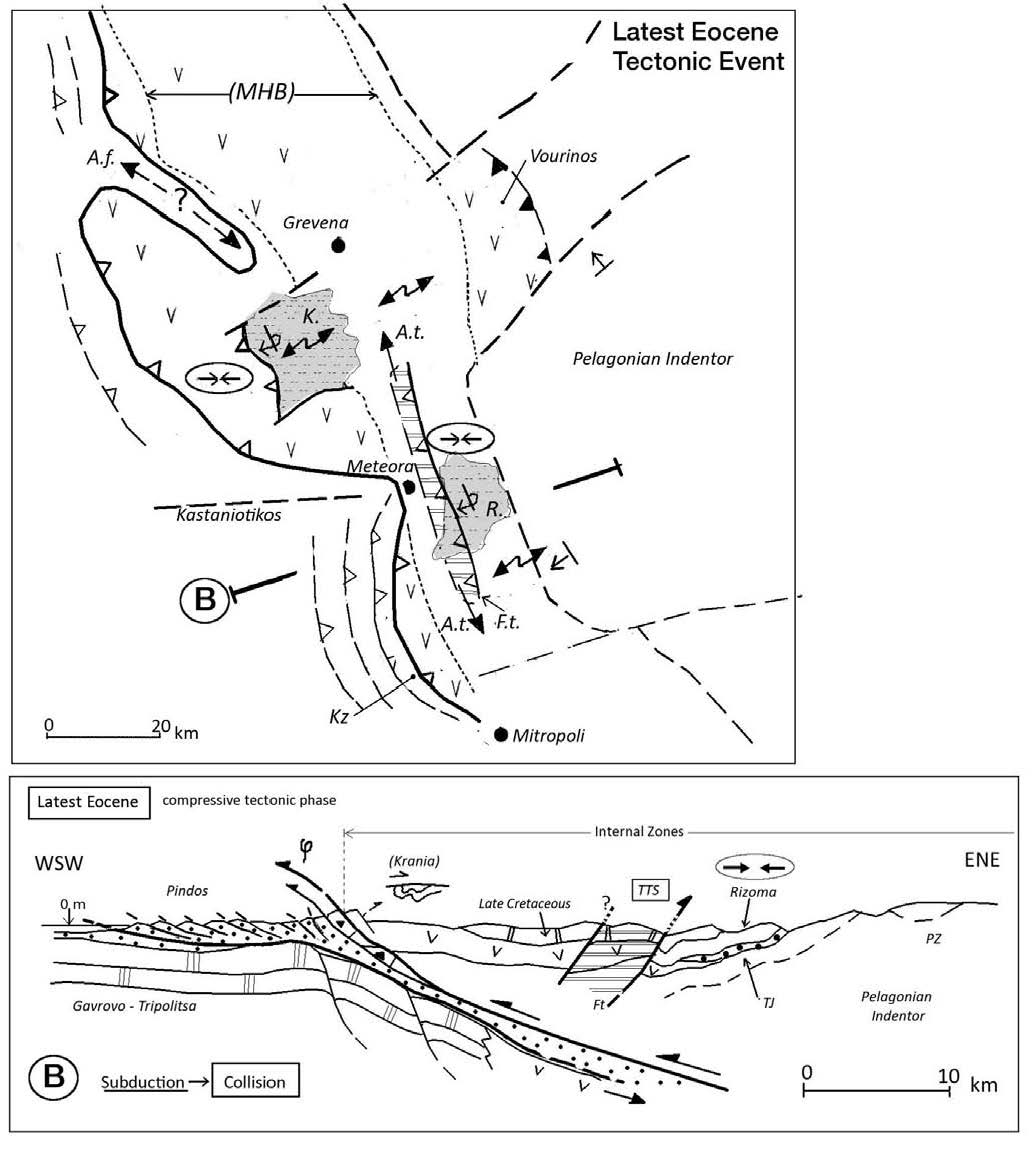
Latest Eocene (till boundary with Oligocene). Development of the main compressive tectonic structures linked, for the authors, to the arrival of the thick Gavrovo-Tripolitsa crust in the subduction zone. The western boundaries of Krania and Rizoma outcrops are active (faulted flexure and reverse faults). The northern boundary of the Krania basin is also active.
The collision is therefore marked in the overriding plate, even though the Gavrovo-Tripolitza crust may have been thinner than a normal continental crust, as suggested by its continuous subsidence during the Mesozoic and early Paleogene at the margin of the Pindos basin [Aubouin, 1959].
From this observation, it appears that the time required for the subduction of the whole Pindos basin is about 10 Ma long (45-43 Ma to 35-33 Ma). The former total width of the Pindos basin, 300 to 600 km in the northern Hellenides, is reconstructed from: i) mapping of the isopic zones; ii) the amount of tectonic shortening estimated from outcrops and balanced cross-sections [Skourlis and Doutsos, 2003], and iii) the estimated extension/spreading rate of this basin made of thin continental crust [Thiebault, 1982] or oceanic crust [Bonneau, 1982]. These studies suggested an average Pindos Basin subduction rate of about 3 to 6 cm/year.
The first elongated major basin:the western MHB
Oligocene initial development of the large MHB
The basal Oligocene sediments are generally thick conglomerate beds resting unconformably on top of deformed basement, including late Eocene sub-basins. The Oligocene Eptachorion Formation is characterized by a very strong tectonic subsidence: the basal continental conglomerates are overlaid by turbidites and then by massive marls indicating an important deepening of the basin floor, up to 600m water depth according to Barbieri [1992]. Locally, subsidence is accompagnied by synsedimentary normal faults and by onlaps of relatively deep turbidites on top of subaerial to shallow-marine conglomerates or reefal limestones (e.g. north of Alatopetra) revealing a sharp steepening of depositional profiles. During that time, the Pindos Fold and Thrust Belt keep rising, as recorded by syntectonic fans in the lower part of turbiditic deposits in the western MHB (north of Alatopetra). Such a strong subsidence in the course of the major underthrusting of a thick crust has to be explained. As the MHB evolves into a major NW- SE marine trough, the process is necessarily at the scale of the whole margin.
The presence of thick dense ophiolitic bodies west of the Pelagonian unit, forming the basement of the MHB from this area of Greece up to Albania [Robertson and Shallo, 2000], could partly control the location of the main subsiding areas. However, the NW-SE direction of the Oligo- Miocene MHB trough, parallel to the Hellenic frontal thrust and to the external zones, argues for a main control by the external underthrusted units. Crustal delamination at the base of the Pelagonian overriding unit (basal tectonic erosion) during the eastward underthrusting of Gavrovo-Tripolitza crust beneath the basin (Fig. 20C) has to be considered as the most probable model to acount for this major synsubduction subsidence during Oligocene times.
Figure 20C. Oligocene (Eptachorion Formation)
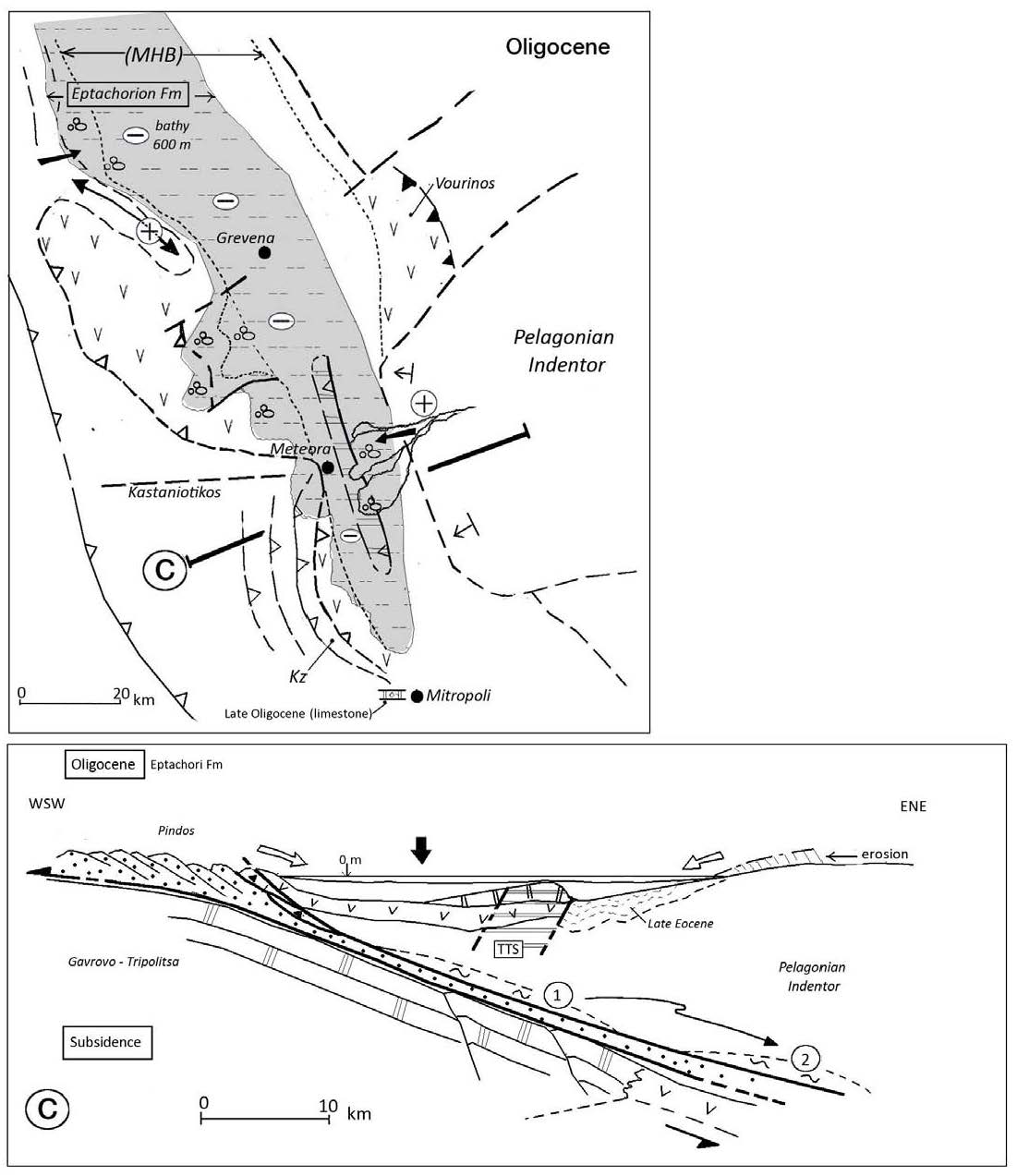
Oligocene (Eptachorion Formation). The elongated wellknown MHB is initiated at this time (Greece and Albania). The development of subsidence while a relatively thick crust (Gavrovo-Tripolitsa zone) is underthrusted has to be explain. We consider that a basal tectonic erosion (numbers 1 to 2) is the mechanism at the origin of the subsidence (see also Fig. 19).
The first major sedimentary change in the main MHB: coarse grained deposits linked to an eastern uplift (Pentalofon Fm, Latest Oligocene – Early Miocene)
The stratigraphic transition from Eptachorion marls to Pentalofon coarse sediments, notably the Lower Meteora Conglomerates, corresponds to a major event in the MHB evolution. Because of the lack of angular unconformity between these two formations, we propose that two main forcing factors have to be considered: eustatic sea-level variations and tectonically induced vertical motions. At that stage, the migration of depocenters toward the inner domains is not really effective.
The stratigraphic transition from turbidites and marls to coarse-grained deltas (e.g. Taliaros Formation or Lower Meteora Conglomerates) reflects an overall progradation trend during the late Oligocene to early Miocene times. Whereas conglomerates of Pentalofon Formation overlie Taliaros deltas in the northern MHB, they directly truncate Eptachorion marls in the south.
On one hand, the imprint of the Rupelian-Chattian eustatic sea-level fall [Haq et al., 1988] could explain this sharp Eptachorion-Pentalofon boundary. Nevertheless, this event seems less prominent on some of the most recent charts edited for the European basins [Abreu and Haddad, 1998] (Fig. 12). On the other hand, we have demonstrated that the conglomeratic fan-deltas, especially the Lower Meteora Conglomerates (Pentalofon Formation), that dominate deposition during the late Oligocene - early Miocene times are mainly due to tectonic activity [Ferrière et al., 2011]. This has been demonstrated by: i) the evolution of topsets geometry of the Meteora Gilbert-deltas that implies progradation over a steep and active basin slope; ii) the reactivation of existing tectonic structures at the time of Pentalofon Formation deposition (deposition of Meteora Gilbert-delta on the side of the Theopetra-Theotokos Structural-high, Pl. III-B); iii) the existence of syn-deposition Early Miocene normal faults on the southwestern side of the MHB (Mitropoli area, no 6 on Fig. 13B ; Pl. III-E).
This basin stage, starting with Pentalofon Formation, was mainly controlled by uplift of the eastern border of the MHB which became the main drainage area (Fig. 20D). The high sedimentation rate associated with conglomeratic deposition led to rapid overfilling of the basin on the west side of the present TTS (Fig. 20D).
Figure 20D. Late Oligocene (?)-Early Miocene
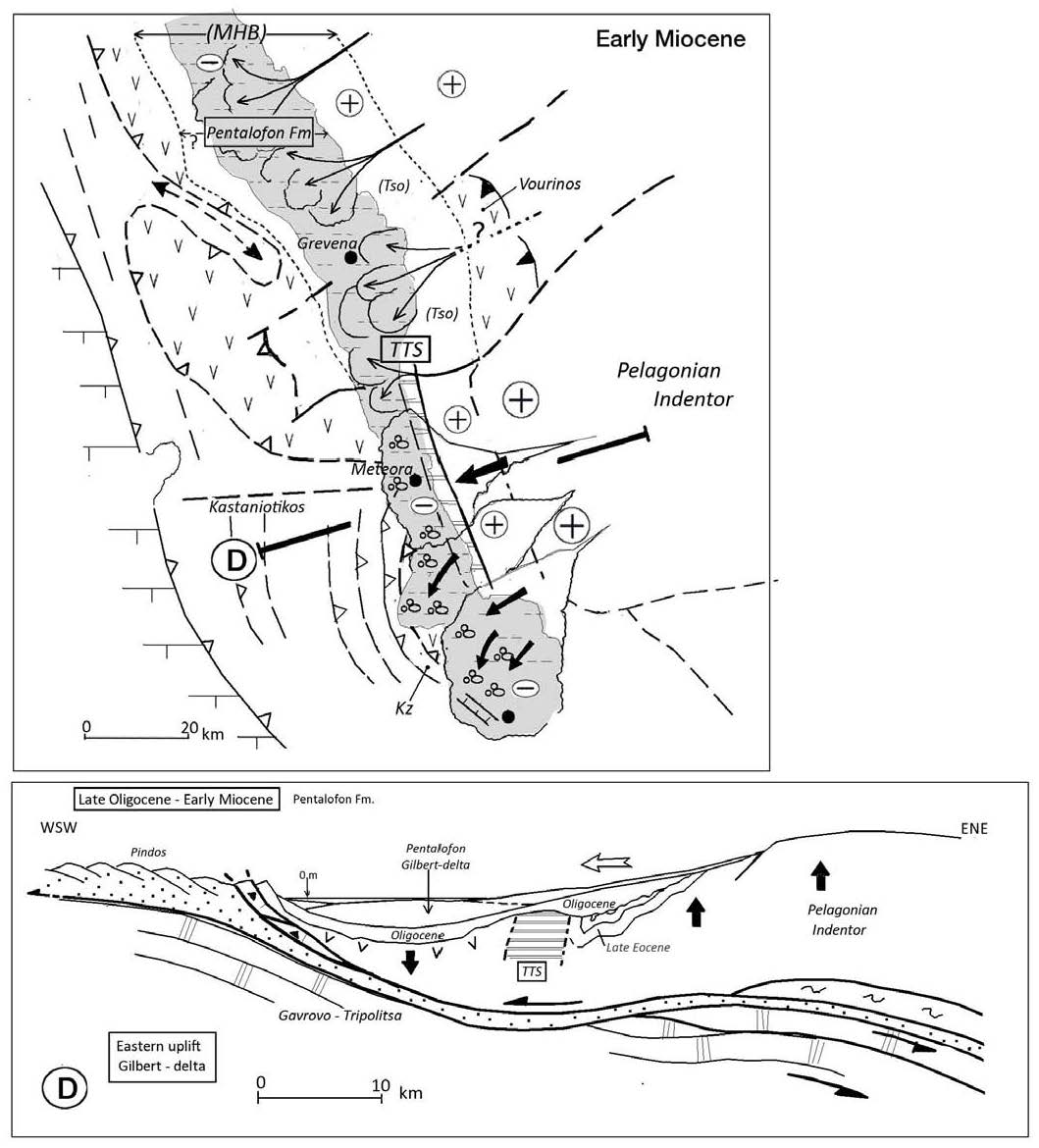
Late Oligocene (?)-early Miocene: Tsarnos Fm (only in the northern MHB) and Pentalofon Fm. A main change appears in the MHB at this time. A major uplift developed on the eastern boundary of the MHB with a subsiding area on its western side (Pentalofon sub-basin). Coarse grained terrigenous sediments are abundant. Some of them are deposited as a Gilbert deltas in the Meteora area [Ori and Roveri, 1987].
The second stage of large basin: the western MHB
The sedimentological transition from Pentalofon to Tsotyli Formation is sharp in the southern MHB but is not really marked in the northern part of the basin. However, the main characteristic of this transition at the whole basin scale is the significant eastward shift of the depocenter. This shift seems to correspond to a brief event. The two successive areas of sedimentation, Pentalofon to the West and Tsotyli to the East, are separated by the TTS in the southern MHB (Fig. 20E).
Figure 20E. Early-mid Miocene (Tsotyli and Ondria-Orlias Fms)
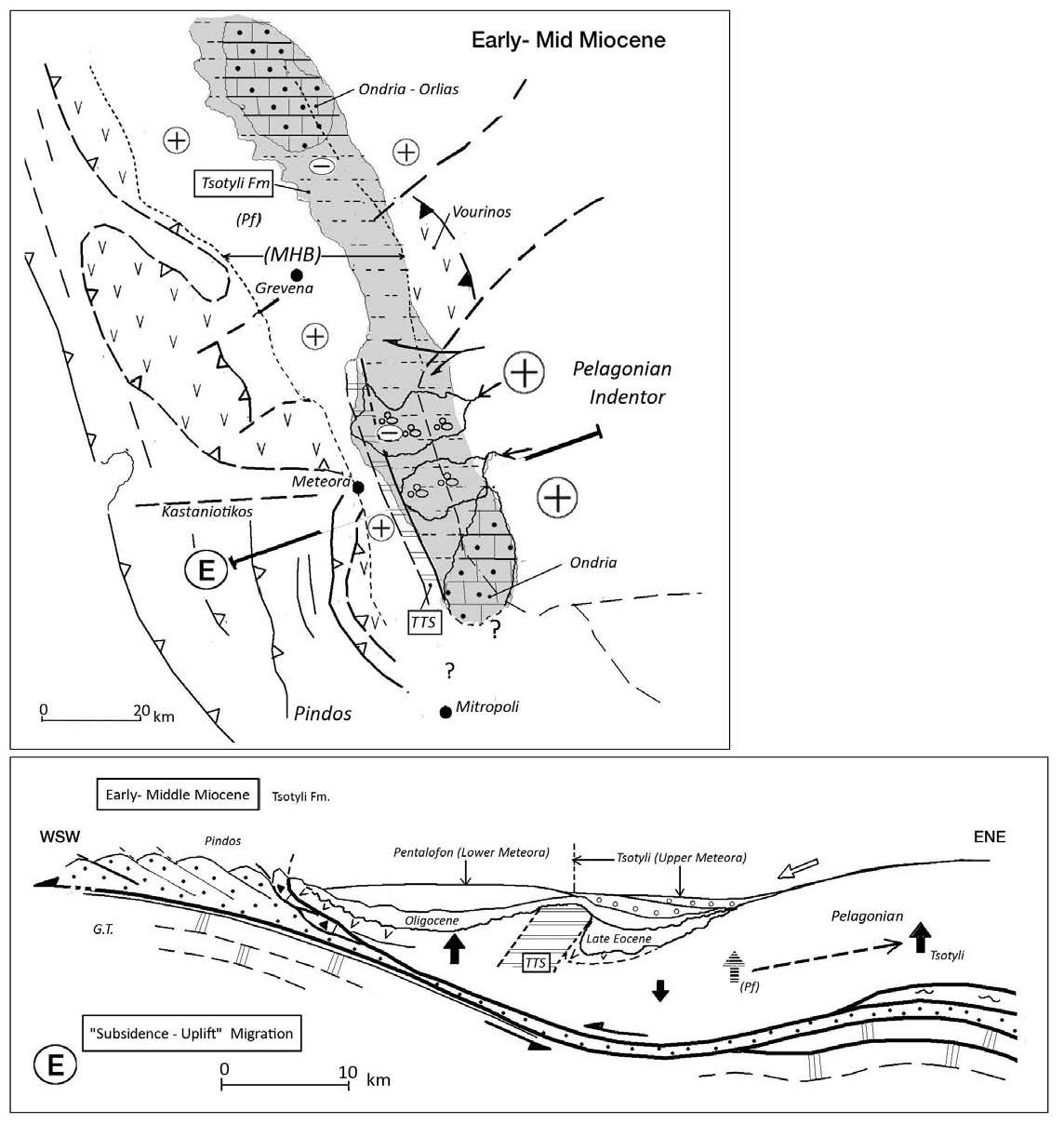
Early-mid Miocene (Tsotyli and Ondria-Orlias Fms). The main event at this time is the migration toward the east of the subsiding and uplifted areas. In the southern MHB, a structural high (TTS) works as a boundary between the old Pentalofon and the new Tsotyli sub-basins. The Ondria-Orlias Fms are the last known marine sediments in the MHB.
This 10 to 15 km brutal eastward shift of the subsidence area to the other side of the Theopetra- Theotokos structure (TTS) can be interpreted as an eastward displacement of the zone of tectonic erosion beneath the basin crust, coupled with an increasing uplift rate in the hinterland because of underplating. These eastward migrations of subsidence and uplifted areas give probably rise to major normal faults well expressed in the MHB (Fig. 4).
The final stage of MHB development: Ondria – Orlias Formations
The latest stratigraphic Formations are only preserved on both southern and northern tips of the MHB. Up North, they only rest on top of Tsotyli Formation whereas in the South, they are lying unconformably on various lithological units ranging from Tsotyli Formation to Mesozoic and Paleozoic basement (Pelagonian zone). The sedimentological trend is a significant increase in carbonate content and a general decrease of water-depth of deposition (shallow water Orlias Formation).
The present-day MHB
The end of deposition in the basin is linked to the general uplift of the MHB area (Fig. 20F) possibly emphasized by the eustatic sea-level drop proposed for the mid-late Miocene [Abreu and Haddad, 1998].
Flexuration between Rizoma and Lagadia (southeastern MHB, Fig. 16A) and folding east of Theotokos carry on during and after basin uplift, illustrate renewal of compressional tectonic activity at this eastern side of the basin. The uplift affects the whole MHB and its amplitude can be estimated to about 1000 m in the North and about 700 to 800m in the South. A Late Miocene compressional tectonic event is generally accepted [e.g. Vamvaka et al., 2006; Tranos et al., 2010] and happened before the well-known Plio-Quaternary extensional period in this part of the Hellenides [Aubouin, 1959; Mercier et al., 1989].
Figure 20F. The Quaternary map of the MHB
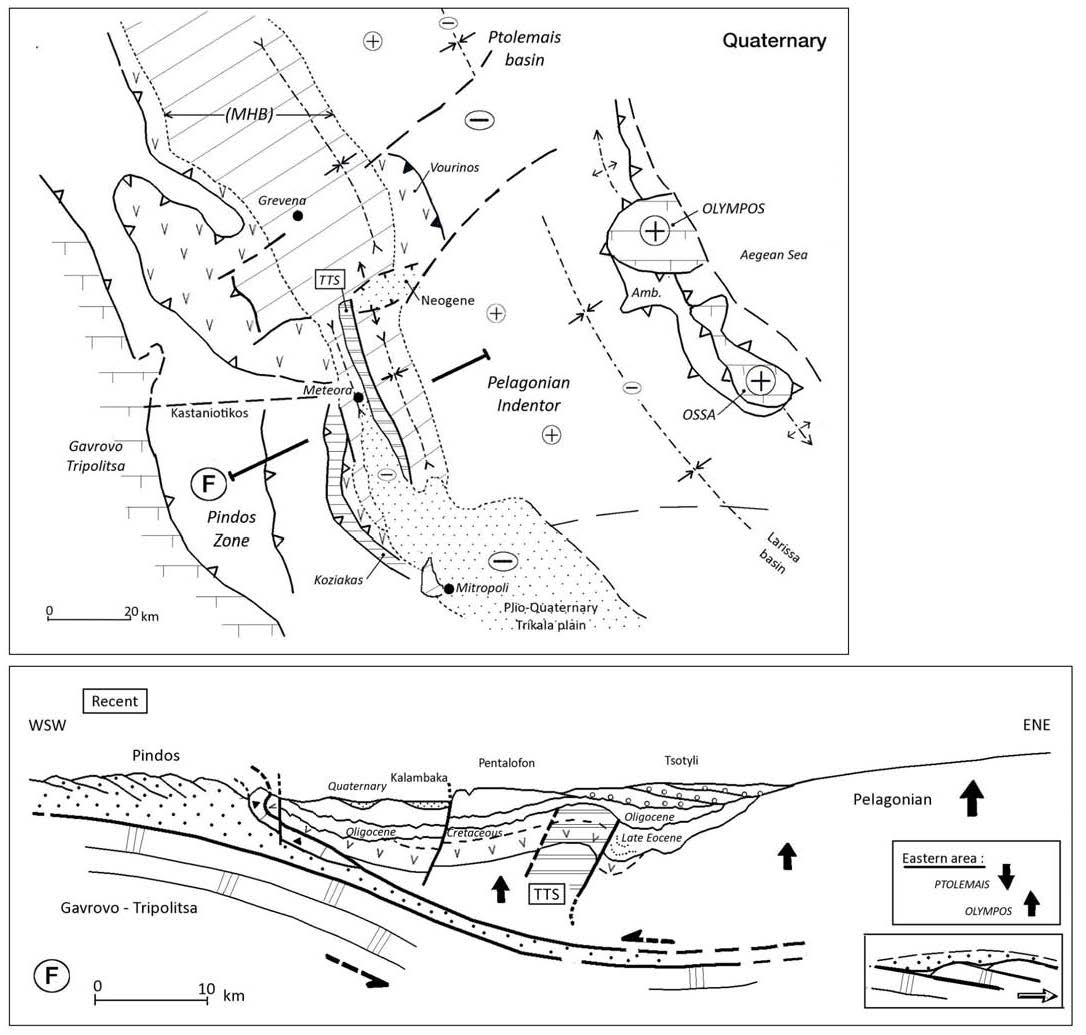
The Quaternary map of the MHB with the Olympos tectonic window on the east which argue in favor of the piggyback nature of the MHB. The MHB has been largely uplifted. The migration toward the east of the subsiding (Ptolemais basin) and uplifted (Olympos-Ossa) areas is the result of a Neogen-Quaternary tectonic activity.
During that post-MHB period, the related subsidence and uplift axis migrated toward the East : Plio-Quaternary subsidence in Ptolemais Basin and uplift in Olympos and Ossa domain (Figs. 20F and 21).
Figure 21. Main stages of MHB evolution
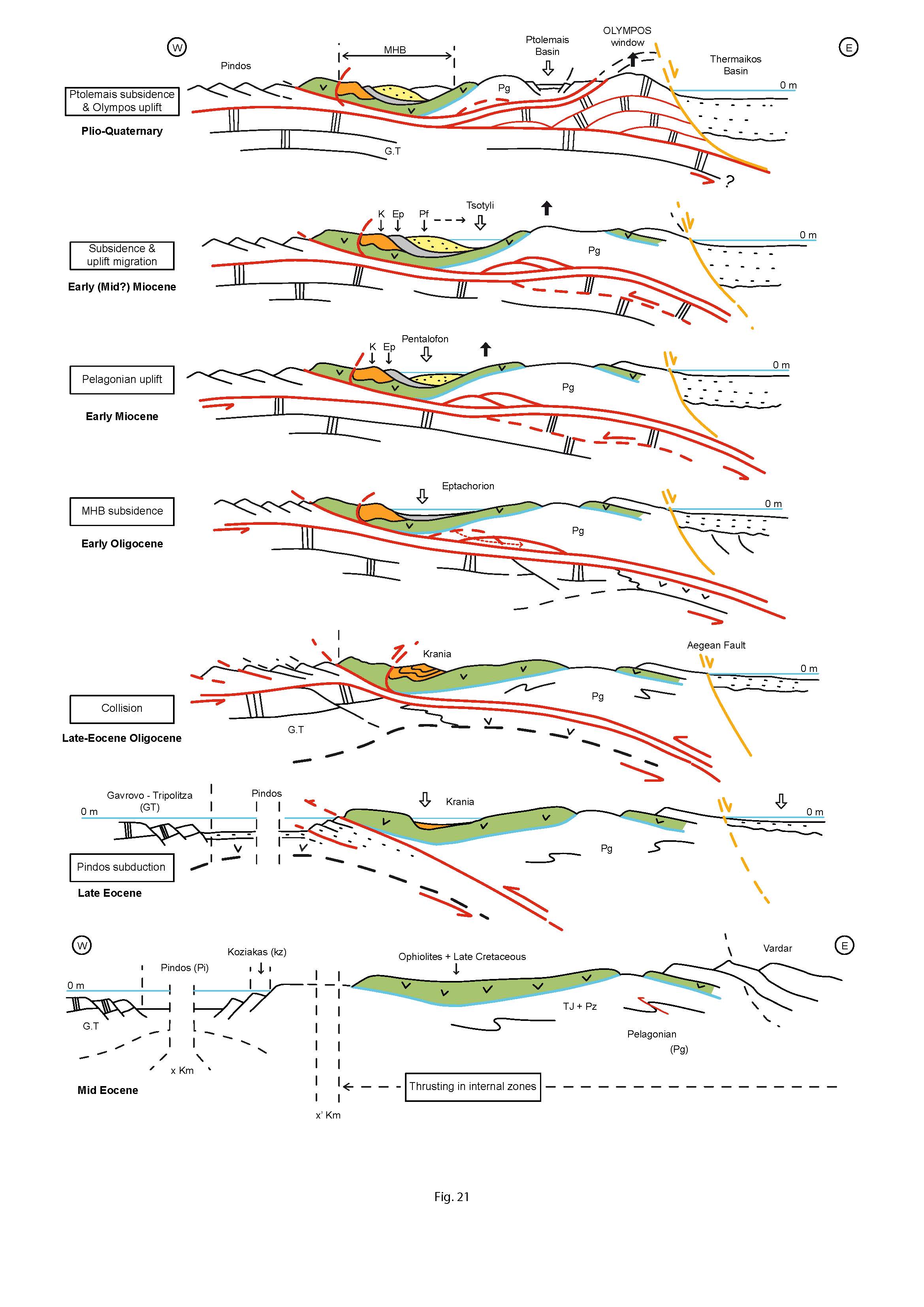
Main stages of MHB evolution in the context of the Tertiary geodynamic evolution of the domain between the Gavrovo- Pindos external zones and the eastern Pelagonian unit above the Olympos window. See text and Figures 19 for detailed discussion on mechanisms.