Discussion
Manning and Hastings oroclines
The four separate datasets, described above, provide persuasive arguments for the presence of a southern Manning oroclinal hinge and a northern Hastings oroclinal hinge. Together these hinges define an orogen-scale, west-verging, sinistral fold pair with a wavelength of ~150 km. In summary, the four datasets are: i) rotation of the fault boundary between subduction complex rocks and the basement to the forearc basin, the Tamworth Group; ii) key Devonian-Carboniferous stratigraphic units, correlated on the basis of fossil and in situ zircon U-Pb SHRIMP ages, which can be tracked around the Hastings orocline and which extend south along the western limb that is shared with the Manning orocline. iii) The change in outcrop shapes of the early Permian overlap assemblage lying above subduction zone rocks and above the boundary between forearc basin and subduction zone rocks; and iv) the curvature of arc proximal rocks and their inferred offshore location.
Six points require further elaboration. Firstly, the forearc basin/subduction complex boundary outlines a southern, south-closing Manning orocline and is inferred to link outcrops of the boundary on opposite limbs of the Hastings orocline around the north-closing hinge before deposition of the early Permian overlap strata. Folding of this boundary around the Manning orocline is most clearly seen in greyscale 1VD image where it is outlined by ultramafic rocks emplaced within the intervening Peel-Manning Fault System (Fig. 1c). The vector pointing oceanward swings from easterly to westerly around the Manning Orocline and then back to easterly on the eastern side of the Hastings Orocline.
Secondly, there is a lack of continuity of forearc basin rocks around the Manning orocline. This reflects the break along the Taree Fault in the eastern part of the hinge zone (Figs. 3, 5), coupled with the strong D2 deformation in the hinge, where stratigraphy is broken up into a series of N to NNW-trending fault slices that fan around the hinge and which lie at high angles to the contact between the forearc basin and the subduction complex (Fig. 3). Map scale and cross section repetition of stratigraphy across these faults (Figs 4, 8b-c) rules out previous ideas wherein they were regarded as extensional faults on the outer arc of a regional fold. Many of these faults may have a strike-slip component to them that is not apparent from construction of cross sections and is not clear from degraded, sporadic outcrop.
Thirdly, amplitudes of the two oroclinal folds vary markedly along the traces of their hinge lines. At the arc/forearc datum line, these folds have smaller amplitudes and are much more open, involving much less limb rotation, than the at the forearc basin/subduction complex datum line (Fig. 1b). These relations suggest that: i) the involvement of the arc/forearc boundary in the oroclines means that emplacement of the Hastings Block in its current location was not simply due to translation and rotation of isolated blocks of the forearc along major faults (see later); and that ii) most of the folding strain was taken up in the more outboard strata, necessarily involving formation of decoupling horizons (see below).
Fourthly, our data do not extend into the subduction complex itself, which has undergone up to seven deformations (Dirks et al. 1992). As a result, we have no independent information from these rocks to indicate whether there is stratal rotation mirroring that suggested by the four datasets above. However, Cawood (1982) suggested that the dominant cleavage also rotated around the orocline, from a 190-200° trend in the west into a 140-150° trend in the east. Rosenbaum et al. (2012) briefly discussed the rotation of foliations in these subduction complex rocks, and noted general dips of 70 to 80º indicative of hinge areas. The faulted contact between forearc basin strata and subduction complex rocks in the hinge region of the Manning orocline implies there must have been mechanical decoupling between the two rock packages.
Fifthly, Rosenbaum (2010) and Rosenbaum et al. (2012) also suggested that the Manning orocline and the Hastings orocline (equivalent to his Nambucca orocline) are also outlined by rotation of granite bodies, linking the Bundarra suite (granite age 294-284 Ma) in the west with the Hillgrove suite (299 Ma to 287 Ma) in the east.
Sixthly, the presence of the Manning and Hastings oroclines implies that the Peel-Manning Fault System has been folded around both hinges. Although largely obscured by early Permian strata, Lennox and Offler (2009) showed discontinuous serpentinites wrapping around the Hastings orocline, although some of these are Devonian in age rather than Cambrian as occurs farther north along the Peel-Manning Fault System. In this interpretation, serpentinites in the Port Macquarie block and between that block and the eastern part of the Hastings Block are parts of the folded fault system, overprinted now in the east by WNW-trending faults.
We thus argue that there are two megafolds in the southern part of the south New England Orogen. The Hastings orocline is outlined only by strata in forearc basin: subduction complex rocks are obscured by early Permian rift basins. Folds in the hinge of this fold plunge both north and south (see Appendix A.), possibly a result of an earlier fold event. The Manning orocline is outlined by the curvature of serpentinites and Permian strata. The western limb of this orocline is outlined by folded stratigraphy, bedding surfaces, D1 faults and D1 fold axial traces. In the hinge and eastern limb of this orocline, D2 NNW-NNE structuring has destroyed map-scale evidence of bedding rotation, although outcrop scale D1 structures can still be identified.
These structural complications mean that neither the Manning nor the Hastings megafold fit the strict definition of Weil and Sussman (2004) that an orocline must have undergone vertical axis rotation. However, they fulfil another criterion, in that these folds developed from a linear orogenic belt. We thus follow Rosenbaum et al. (2012) in suggesting that the tem orocline can be broadened, in this case to cover structurally complex hinges involving multiple deformations.
Where did the Hastings block come from?
Most models of the New England Orogen agree that the original location of the Hastings block was south of its present location, and somehow connected to the southeastern part of the Tamworth belt. A critical question is whether the Tamworth belt and the Hastings block were ever physically connected, with continuity of strata before covering by early Permian overlap sequence, or whether they were they separated by major strike-slip and/or contractional faults. Roberts et al. (1995) used stratigraphic and facies differences to suggest there was similarity, but no continuity of strata between the Tamworth belt between the two. They concluded that the two regions, although not directly contiguous, formed in a sedimentary basin close to a major long-lived arc at or near the western margin of the New England Orogen. Thus, the Hastings Block is not an exotic terrane.
This conclusion precludes a palaeogeography wherein the Hastings Block was a simple along-strike extension of the most easterly part of the outcropping Tamworth belt, and that there was simple folding of continuous stratigraphy around both oroclines. Either a now-missing part of the forearc basin lay between them, or the Hastings Block formed farther from the arc, again with intervening strata missing. Where could these missing strata be? One possibility is in the footwall of the Taree fault that separates the Tamworth belt from the Hastings Block (Figs. 3, 5). Stratigraphic separation across the fault suggests reverse south-block up displacement of Famennian strata on the south against Carboniferous strata on the north, and Lennox and Offler (2009) presented evidence for left-lateral strike-slip. If the fault had a moderate to shallow southerly dip, the missing strata could lie in its immediate footwall.
Our preferred possibility, however, is that the missing part of the forearc basin has been overthrust from southwest to northeast (in the present reference frame) by the eastern part of the Tamworth belt, producing the east vergent structures and the west-dipping detachment in the cross sections C and G (Figs. 4, 8b, c). In this interpretation, the amount of missing forearc basin is ~40 km in section C and ~60 km in section G. These are minima assuming no imbrication or folding of the underthrust plate.
Timing of deformation
In the Hastings Block (Fig. 5), the earliest signs of uplift of the subduction complex and redeposition of detritus into Late Carboniferous (Namurian) strata of the forearc basin are recorded by clasts of high-level granite, slate, phyllite, minor quartzose sandstone and especially radiolarian chert, in the Majors Creek, Kullatine and Youdale (c) formations, although they are subordinate to dominant dacitic and andesitic material from the arc (Roberts et al. 1995). More direct constraints on this Namurian deformation of the subduction complex is provided by the ~311 Ma early metamorphism of sedimentary rocks in the Wongwibinda complex, west of the Nambucca Block (Fig. 3) up to 18 myr before emplacement of the ~293 Ma Abroi pluton (Craven 2010). Similarly, the Tia Granodiorite was emplaced at ~296 Ma, during the peak D5 deformation in the Tia complex (Fig. 3) (Dirks et al. 1992; Cawood et al. 2011b) and after ~318 and ~312 K-Ar white mica ages (Watanabe et al. 1988) that approximate D2 deformation (Dirks et al. 1992).
In the northern Hastings Block, deformation at the Carboniferous-Permian boundary is reflected by erosional unconformities between latest Carboniferous (Namurian strata, extending up to ~312 Ma) and Asselian strata (300-295 Ma) and below Artinskian strata (~280 Ma) that are chert-bearing. At Cranky Corner in the southern Tamworth belt (Fig. 3), this Carboniferous-Permian deformation is recorded by a probable 13 m.yr. time gap between latest Carboniferous volcanogenic sedimentary strata (303.7 to 300.3 ± 2.4 Ma), and the oldest U-Pb SHRIMP age, 287.1 ± 2.4 Ma, in overlying late Asselian to early Sakmarian strata (Stevenson 2003; Claoué-Long and Korsch 2003). The widespread presence of chert detritus in the early Permian (Asselian to Artinskian, Briggs 1998) Manning Group, deposited across the boundary between the forearc basin and subduction complex in the core of the Manning orocline, attests to widespread continuous or intermittent deformation of the subduction complex during early Permian deposition.
Younger Permian deformation is well-defined at Cranky Corner in the southern Tamworth belt. Conglomerate in coal measures dated as upper Artinskian (approximately ~277 Ma) has eroded down into older Permian strata with SHRIMP ages of 285.4 ± 2.2 and 285.8 ± 3.0 Ma, suggesting a hiatus of ~7 million years (Balme and Foster 2003; Claoué-Long and Korsch 2003). Clasts of green chert and red jasper occur in this conglomerate, derived from uplift of the subduction complex (Stevenson 2003).
A minimum age for deformation of the southern part of the Tamworth belt is constrained by middle Permian strata in the D2 Stroud-Gloucester and Myall synclines located in Fig 3. In the Stroud-Gloucester Syncline, the youngest unit is a conglomerate with inferred palynology late stage 5, Kazanian to Tatarian flora. While Briggs (1998) suggested this boundary lay at ~257 Ma, Gradstein et al. (2004) showed it to be at 267 Ma and middle Permian. Middle Permian strata lie with an angular unconformity to disconformity on early Permian volcanics, with a time gap corresponding to the Artinskian and Kungurian stages (Roberts et al. 1991). Deformation is thus no older than 267 Ma. The Crowthers Road Conglomerate, the top unit in the Myall Syncline, contains detritus of chert as well as volcanic rocks from underlying units (Roberts et al. 1991), suggesting that regional deformation at this time affected the arc, the forearc basin and the subduction complex. This minimum age of deformation of the southern Tamworth belt is tightly constrained by the emplacement of the stitching Barrington Tops Granite SHRIMP dated at 267.2 ± 1.4 Ma (Cawood et al. 2011b).
Other age constraints on oroclinal folding include; i) the folding of inliers of early Permian Manning Group around the Manning orocline. Briggs (1993) estimated an upper age on these units as Late Artinskian ( ~280-275 Ma); ii) onlap of Permian turbidites of the southern Nambucca Block onto the early Permian strata in the northern Hasting block; iii) formation of an S1, east-west cleavage in early Permian strata in the northern part of the Hasting block, correlated by Lennox et al. (1999) in age and orientation with east-west trending cleavages in the adjacent Nambucca block to the north and northeast. These cleavages have 260 Ma Ar-Ar cooling ages, following a deformation inferred at 264-260 Ma (Offler and Foster 2008); and v) folding of the early Permian (~280 Ma, Artinskian) Yessabah Limestone around the northern hinge of the D2 Parrabel anticline in the Hastings Block.
The last major ductile deformation recorded in the forearc basin occurs in the southeastern part of the Hastings Block where open folding occurred between deposition of late Early Triassic fluviatile strata and Late Triassic to Early Jurassic intrusions has also deformed the underlying Carboniferous strata (Pratt 2010).
A new model of oroclinal folding
Our new model of oroclinal folding is based on coupling new structural datasets (the recognition of thrust geometry, the recognition of two deformation phases in the core of the Manning orocline, and the new interpretation of the Hastings Block) with previous ideas on sedimentary facies distributions in the forearc basin, and also taking the Offshore Uplift into account. Our model suggests that oroclinal folding not only affected the subduction complex and forearc basin, but also the interface between the arc and the forearc basin. We use the variations in shortenings along the inboard edge of the New England Orogen as indicators of more general variations in shortening in the New England Orogen, but we admit that this point needs to be more thoroughly tested. Finally, interpretations of our datasets, which suggest fold–dominated rotation of blocks and minimal translations, differ from models invoking strike-slip fault displacements of hundreds of kilometres, guided in many cases by interpretation of palaeomagnetic data.
Figure 10. Cartoon showing preferred model for the evolution of oroclines in the New England Orogen.
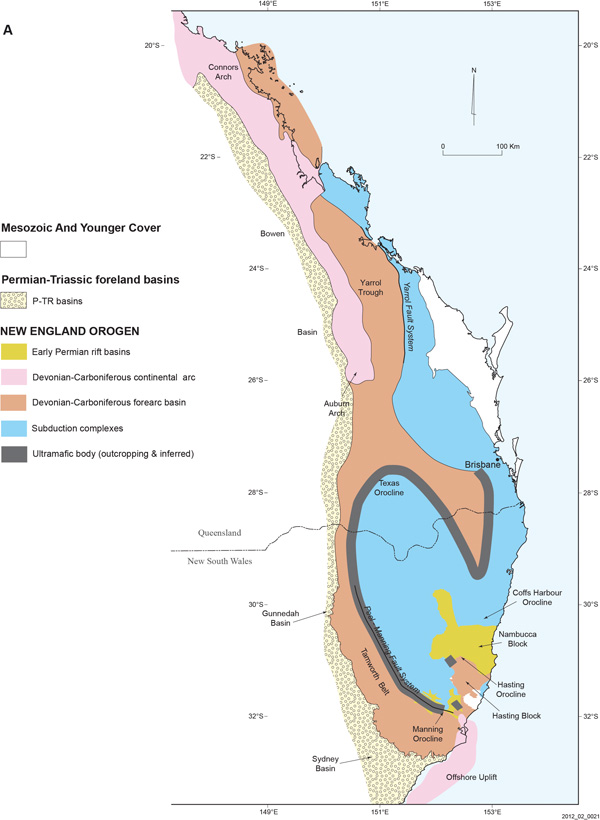
A. Simplified regional map of the whole New England Orogen, based on figure 1b, but showing tectonic elements interpreted beneath Mesozoic and younger cover that obscures join between northern and southern parts of the orogen. Western margin of forearc basin based on Korsch et al. (2009); eastern margin based on interpretation of figure 1c.
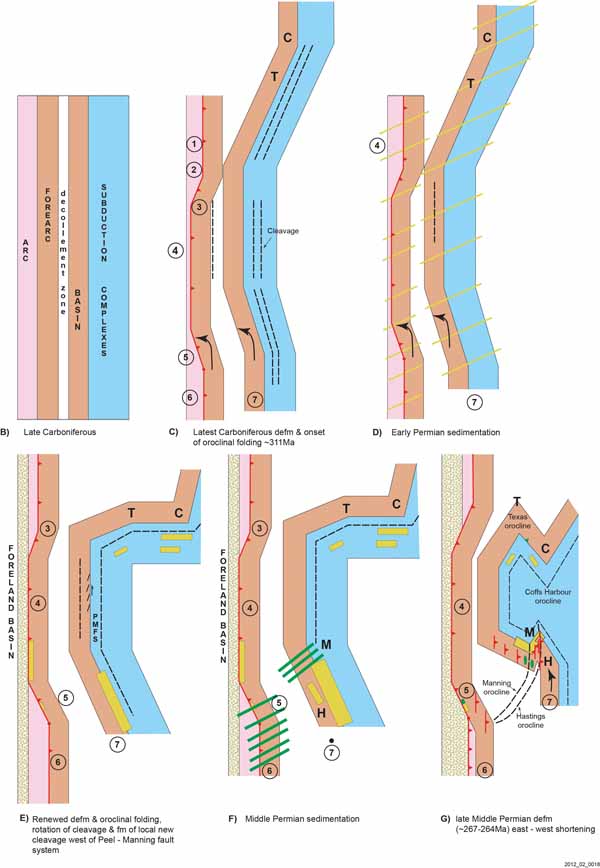
B-G. Outline model for orocline formation. See text for descriptions. Yellow stripes in D indicate approximate area of early Permian sedimentation; preserved areas are shown in yellow polygons. Green stripes in F indicate approximate area of middle Permian sedimentation; preserved areas are shown in green polygons in G. Note that in G, green middle Permian strata on the eastern side of the Texas orocline represents the Gilgurry Mudstone that postdates megafolding. Other schematic green outcrops in G predate east-west shortening. Abbreviations: C=Coffs Harbour orocline; H=Hastings orocline; M=Manning orocline; T=Texas orocline.
Our model of the Manning and Hastings oroclines suggests that the stratigraphy in both hinges formed in nearby, but not contiguous, parts of forearc basin. The simplest configuration is one in which the two sequences were along strike from each other but separated by an unknown distance. We suggest this minimum distance was 40-60 km. Imposed on this stratigraphic architecture is a structural one, in which the forearc basin was converted to a largely west-vergent fold thrust belt, developed above one or more crustal detachments. Largely north to northeast-dipping bedding panels in D1 thrust sheets were increasingly overprinted towards the hinge of the Manning orocline by D2 NNW to NE-trending, west-dipping structures (Glen and Roberts 2010).
The model begins with a ~2000 km largely linear Carboniferous convergent margin now deformed as sshown in Fig. 10a. We develop it, somewhat simplistically, in subsequent figures 10b to 10g, with discrete deformation pulses, but are aware that these might have been continuous. We focus on the southern segments of this margin as possessing a simple linear meridional configuration (western arc, central forearc arc basin and eastern subduction complexes) (Figs. 10a, b). Because the major mismatch in orocline geometry between two datum lines referred to above requires the development of internal discontinuities in the forearc basin during deformation, we have shown the forearc basin in two parts, separated by this ‘future’ decollement zone.
Early shortening in the forearc basin and subduction complex, which we suggest was associated with the first stages of orocline formation, occurred in the latest Carboniferous, below regional Permian unconformities and during waning subduction or after cessation of subduction at ~305 Ma (Fig. 10c). We attribute the subvertical dips of bedding and (sub) parallel cleavage in the Texas and Coffs Harbour oroclines (Lennox and Flood 1997; Korsch 1993) to this deformation (Fig. 10c), although fabric and steepening could have formed during earlier accretion. Similarly, we suggest that the sporadically developed regional cleavage in the Tamworth belt might be of this age. As part of this deformation, we show the first stages of westward thrusting of the forearc basin over arc rocks (Fig. 10c). Different amounts of shortening must have been a feature of the system and this is shown somewhat simplistically in Fig. 10 by how much of the arc has been overthrust by the forearc basin. Thus the NE-tending segment 3 links lesser amounts of shortening in segment 2 to the north with more shortening in segment 4 to the south. Similarly, segment 5, represented by the frontal Hunter Thrust, is pinned to the south in a structural recess, but increases in displacement as it sweeps to the north, to link with more shortening in segment 4. This event was followed by deposition of early Permian sedimentary and volcanic rocks in rifts or transtensional basins above this deformed package (Fig 10d), the latter increasingly characterised by backarc and MORB-type signatures, as the plate boundary retreated eastwards.
The second phase of shortening occurred in the late early Permian, around 284-277 Ma, constrained by Permian strata folded around the Texas orocline (Lennox and Flood, 1997; Li et al. 2012) and by the unconformity in the southern Tamworth belt. We suggest that this deformation involved renewed westward thrusting of the forearc basin over the arc, in segment 4 especially, the beginning of major folding in the Texas and Coffs Harbour oroclines, and the deformation of Permian overlap strata to form outliers along or near the forearc basin/subduction complex boundary (Fig. 10e). Formation of the Peel-Manning Fault System, containing early Palaeozoic lithologies in a serpentinite matrix, occurred then, with limited left-lateral kinematics reflected by a NE-trending cleavage along some eastern parts of the forearc basin (eg. Cao and Durney, 1993). This was followed by deposition of middle Permian strata (Fig. 10f).
Tightening of the Texas and Coffs Harbour oroclines, and final formation of the Manning and Hastings oroclines, is attributed to the middle Permian (Fig. 10g). In the Manning orocline, this deformation postdates 267-264 Ma sedimentation that is stitched by a 267.2 ± 1.4 Ma granite. The Hastings orocline may be a few million years younger, folding an early cleavage correlated with the 264-260 Ma cleavage in the Nambucca block to the north. In segment 5, this shortening is reflected by formation of D2 folds and thrusts, overprinting D1 structures, the tightness and displacement of which increase towards the hinge of the Manning orocline, increasing the outcrop width of the forearc basin (Fig. 10g). Thrusting of southern Tamworth belt a minimum of ~60 km northeast over the ‘southwestern part’ of Hastings Block is a late part of this D2 deformation, and is responsible for juxtaposition of different forearc basin packages against each other.
The amplitude of the Texas and Coffs Harbour oroclines is magnified by post-granite dextral slip on the N-trending Demon Fault, although this is estimated to be only ~ 17 km (Korsch et al. , 1978) or ~23 km (McPhie and Fergusson, 1983). The amplitude of the Hastings orocline is magnified by plunge changes in the Hastings Block, where variable and low plunges (Lennox and Roberts 1988) contrast with subvertical dips in subduction complex rocks in the Coffs Harbour and Texas oroclines, though in the latter Lennox and Flood (1997) showed low plunges in folded Permian outliers.
As this stage we cannot be certain about the causes of the variations in shortening directions and amounts outlined in Fig. 10. We cannot tell whether they reflect changes in the direction of push from the plate boundary, or were caused by irregularities in the underlying basement (Devonian–Carboniferous arcs or older Lachlan orogen crust). Because we think the bulk of orocline bending occurred during a lull in continental arc magmatism, coeval with the formation of rift basins and changes in volcanic geochemistry from subduction to rift to MORB-like, we suggest it occurred during rollback of the plate boundary. Such an environment, with less coupling between plates, would have allowed the spatial freedom for orocline formation to occur (see for example a modern example, in Rosenbaum and Lister 2004). Our working model is thus that the variations in shortening reflect an irregular continental margin, with embayments and promontories in the continental margin, corresponding to salients and recesses in the thrust belt, which influence the amounts and directions of maximum shortening. These irregularities must underlie shape variations in the foreland basins, implying that at deeper levels, soft or hard-linked weak zones of flowage occur below the basal detachments that propagated west of the forearc basin into the foreland basins.
This shortening model for the formation of New England Orogen oroclines differs from most previous models in which the megafolds were inferred to have developed in response to sinistral or dextral simple shear on a major N to NE to NNW-trending master fault located either onshore or offshore. These models are now discussed and commented upon.
Previous orocline models for the New England Orogen
Murray (1997) summarised the evolution of ideas that led from the concept of simple cross faulting to the recognition of oroclinal bending in the southern parts of the New England Orogen. Korsch and Harrington (1987), Harrington and Korsch (1987), Cawood and co-workers and Collins et al. (1993) recognised only three oroclines: Texas, Coffs Harbour and Manning. Lennox and coworkers and Offler and Foster (1998) argued that Manning orocline did not exist, but accepted that the Hasting Block was most likely allochthonous in its current position. Rosenbaum et al. (2012) supported the presence of the Texas, Coffs Harbour and Manning oroclines, and recognised the northern expression of the Hastings orocline (Nambucca orocline).
Dextral strike-slip models
Murray et al. (1987) suggested the Texas and Coffs Harbour oroclines formed by dextral slip on an inferred Gogango-Baryulgil Fault At the same time Harrington and Korsch (1987) proposed that oroclinal bending occurred in response to dextral strike-slip faulting, but they argued that the Mooki Thrust was the master fault. Lennox and Flood (1997) suggested dextral transpression in eastern Australia occurred in a broad shear zone oriented SE-NW. Offler and Foster (2008) inferred oblique subduction on the plate boundary. Instead of partitioning deformation with formation of a dextral fault onland, these authors invoked pinning in the south, which led to buckling of the subduction complex about a vertical axis. Vertical axis rotation is consistent with subvertical dips measured in subduction complex rocks in the hinge of the Coffs Harbour orocline (Korsch 1993) and with subvertical folds and intersection lineations in the hinge of the Texas orocline (Lennox and Flood 1997; Li et al. 2012). There, Lennox and Flood (1997) showed that bedding in the subduction complex had been folded about an axis plunging at 76°-164°, with cleavage folded about an axis plunging 82°- 316°. More recently, Li et al. (2011) recorded a fold axis in S1 cleavage plunging 80° - 255°. Lennox and Flood (1997) also reported that the intersection (=fold) lineation in Permian outliers around Texas orocline plunged at 28° - 315°, implying steepening of bedding dips in subduction complexes before the Permian. Lennox and Flood (1997) also reported a weak fanning S2 cleavage and an overprinting E-W S3, but Li et al. (2011) did not record any penetrative S2 cleavage. The dextral model for the Texas and Coffs Harbour oroclines of Li et al. (2011) suggested that oroclinal folding at 280-265 Ma occurred in response to dextral strike-slip on an offshore meridional fault. This folding was followed by <265 Ma E-W shortening and was imposed upon a non-linear margin, either acquired during early Permian rollback of the subduction zone or to an older palaeogeography.
Sinistral strike-slip models
In contrast to the views above, several authors suggested that the New England oroclines formed by strike-slip movement on sinistral faults in response to a change in the nature of the plate boundary from convergent to transform. Cawood (1982) and Cawood and Leitch (1985) suggested that the Hastings Block originally formed immediately south of the southern Tamworth belt. Cawood (1982) invoked sinistral strike-slip movement on a series of rotating N-NNW-trending faults to produce the Parrabel anticline in the Hastings Block and the broad curvature of the southern Tamworth belt. Collins et al. (1993) extended the reconstruction of Cawood and Leitch (1985), suggesting that the Hastings Block originated ~500 km south of the southern Tamworth belt, and underwent ~1000 km of northward translation, accompanied by anticlockwise rotation. Collins et al. (1993) also suggested that the Nambucca block had been translated northwards along with the Hastings Block, in contrast to previous ideas that the block formed in situ and that the E-W structural fabric (Leitch 1978) formed by squeezing it between the northward-moving Hastings Block and the southward-moving Coffs Harbour block (eg. Scheibner 1976) (see Offler and Foster (2008) for a counter argument). Based on palaeomagnetic measurements, Schmidt et al. (1994) favoured a clockwise 130°rotation of the Hastings Block as part of any displacement.
The reconstruction of Cawood al. (2011b) was guided by existing palaeomagnetic data, which they pointed out were limited in nature and thus incapable of producing an unequivocal tectonic model. However, they noted that these data suggested that dextral strike-slip models were not permissible, whereas sinistral translation was permissible. Their model was able to generate all four of the oroclines by buckling in response to sinistral movement along an offshore NNW-trending fault, the tip point of which lay in the core of the Coffs Harbour orocline. Figure 5 of their paper envisages large scale displacements, with the Hastings Block forming 1600 km south of present location, the southern Tamworth belt 1080-830 km to south, and the northern Tamworth belt and Texas block 470-400 km to the south of their present locations. In addition to this northward movement, their figure 5 suggests that there were hundreds of kilometres of westwards movement: the hinge of Texas orocline was translated 340 km; the north Tamworth belt 440 km and the hinge of Manning orocline 400km.
Timing of oroclinal bending
Murray et al. (1987) suggested that the New England oroclines formed in the latest Carboniferous (310-300 Ma). However, more recent papers favoured formation in the Permian, before emplacement of the large, latest Permian–Triassic granites of the New England Batholith. Offler and Foster (2008) suggested that the Texas and Coffs Harbour oroclines formed between 273 and 260 Ma. Rosenbaum et al. (2012) suggested formation between 295-285 Ma and a second phase prior to ~260 Ma. Cawood et al. (2011b) suggested that oroclinal bending occurred between 270 and 265 Ma. In contrast, Aubourg et al. (2004) argued that in the Texas orocline, ~40° of rotation had occurred before extrusion of volcanics in one of the deformed early Permian outliers, with a further ~80° rotation afterwards. They further showed that this rotation had been overprinted by the ‘Kiaman’ reversal dated at ~265 Ma, and predating deposition of flat-lying late Permian strata in the Emu Creek Block. However, the age of these strata is controversial. Briggs (1998) suggested that these sedimentary rocks had a maximum age of ~252 Ma, whereas Waterhouse (1967) suggested a late Kungurian or early Kazanian age, i.e. around 270 Ma (Gradstein et al. 2004).
Comparison with previous models
Our fold model above considers that the Hastings block arrived at its (near) present position due to oroclinal folding coupled with underthrusting. This is not compatible with models in which the Hastings block is inferred to be a separate terrane that drifted in along faults (eg. Cawood 1982; Collins et al. 1993; Schmidt et al. 1994) and underwent rotation as a separate microplate. The rotation model of Schmidt et al. (1994) favoured a clockwise 130° rotation of the block to restore it to the Carboniferous part of the Gondwana Apparent Polar Wander path (APWP), but they indicated that a 230° anticlockwise rotation was also possible. This was placed in a context wherein the southern Tamworth belt was subdivided by facies into three structural blocks separated by N to NE-trending faults ~ 40 km apart (Roberts et al. 1991). Geeve et al. (2002) used palaeomagnetic data to infer these blocks had undergone anticlockwise rotations of 80°, 80° and 120°. However, the continuity of stratigraphy coupled with two generations of thrusting (see above) allow the possibility that the vertical axis rotations are the result of D2 overprinting D1 thrusts with variable displacements in segment 5 of Fig. 11. As a result, we suggest the limb rotation of our model is ~90-120° anticlockwise. This amount is closer to the anticlockwise rotation of ~150° suggested by Klootwijk (2009) if the block is rotated back to the Klootwijk-Giddings (KG) variant of the Gondwana APWP rather than the Schmidt-Li-Powell (SLP) variant used by Schmidt et al. (1994).
Although it did not discuss the Manning or Hastings oroclines, the model of Li et al. (2011) has two similarities with our model. Firstly, it envisages multiphase orocline formation, beginning before the Permian. Secondly, it discusses the possibility that the early phase may have involved a non-linear margin of Gondwana. The model by Rosenbaum et al. (2012) also has some similarities. It envisages enhancement of a primary curvature during variable early Permian roll back of the plate boundary to the northeast, accompanied by dextral strike-slip faulting offshore followed by fold tightening during E-W shortening and re-establishment of subduction.
Our model of minimal displacements is an alternative to the large displacement model of Cawood et al. (2011b). They suggested that the arc, forearc and subduction complexes in the northern (Queensland) part of the orogen were autochthonous, whereas those in the southern (NSW) part of the New England Orogen were allochthonous. Beginning at ~275 Ma, their model involved translations of 1600-~500 km northwards and ~400 km westwards, with convergent margin elements presumably thrust onto the pre- or early Permian Gondwana margin. These north-to-south differences, however, do not appear to be reflected in the evolution of the Permian-Triassic Bowen-Gunnedah-Sydney basin system to the west. The >1200 km long, latest Carboniferous-early Permian volcanic-filled, mid-crustal rift (Meandarra gravity ridge) that marks the beginning of this basin system extends from the beneath the Sydney basin to beneath the central part of the Bowen basin without deflection (Krassay et al. 2009). And foreland basin loading phases of the Bowen Basin (in Queensland in the north) and the Gunnedah Basin (in NSW in the south) show parallel and similar sedimentary development (Brakel et al. 2009; Totterdell et al. 2009). Movement of elements of the southern New England Orogen 400 km to the west would thrust them above crust of the Lachlan Orogen that lies west of the New England Orogen, thereby leading to possible Lachlan sources for granites in the western parts of the New England Orogen. Both Chappell (1994) and Shaw et al. (2011) have emphasised the differences between Lachlan and New England Orogen granites, although the Bathurst granites in the Lachlan Orogen have some similarity to the Mooki granite in the New England Orogen.
Finally, although we are unable to differentiate between deformation and uplift of subduction complex rocks and oroclinal bending at ~311 Ma, our suggestion for latest Carboniferous onset of oroclinal folding is similar to the suggestion of Aubourg et al. (2004) that formation of the Texas orocline began before early Permian sedimentation at ~299 Ma. Li et al. (2012) have also suggested prolonged formation of the Texas and Coffs Harbour oroclines, with the first phase inferred to be synchronous with, or older than, Carboniferous subduction.