Ophiolites
The Luobusa ophiolite, Tibet, lies along the suture between Asia and India. It was emplaced early in the Tertiary epoch (Aitchison et al., 2002). Although faulted and moderately serpentinized, it consists primarily of harzburgite and dunite and exhibits minor pillow basalts and cherts (Zhou et al., 1996). It has been interpreted as having formed at a mid-ocean ridge and subsequently modified in the mantle wedge above a subduction zone (Malpas et al., 2003). It shows no evidence of either subduction-zone metamorphism or meteoritic impact.
Since the 1980s there have been periodic publications reporting diamonds and a bewildering array of other phases including silicon carbide and exotic metals (see Yang et al., 2003 and Robinson et al., 2004 and references therein) extracted from a massive chromite deposit found at an altitude of about 5,000 meters. The overwhelming evidence that ophiolites form at oceanic spreading centers has led to extreme reluctance of the community to accept that the reported materials, not found in place but only in heavy mineral separates from massive chromite, are real, indigenous, minerals of the ophiolite. However, Yang et al. (2007) described a diamond inclusion within a grain of OsIr alloy and a pellet of Fe-Ti metal to which is attached a small fragment of silicate rock consisting primarily of coesite and kyanite (Figure 3a). Loose diamonds previously reported from Luobusa chromite had essentially been rejected as probable contamination. The presence of a diamond included within a grain of OsIr alloy was more convincing as indigenous to the rock because many OsIr pellets have been identified in intact specimens of the massive chromite ore. Most importantly, however, was the identification of coesite by Raman spectroscopy that finally presented an observation from these remarkable rocks that is impossible to reject as contamination. The presence of coesite requires that the chromite crystallized at a depth in excess of 90 km and diamond extends that depth to a minimum of ~120 km. Further, the coesite exists as elongated prisms that strongly suggested pseudomorphic replacement of stishovite, which would imply much greater depth; demonstration that the elongated prisms of coesite are polycrystalline, greatly enhanced the stishovite interpretation.
Figure 3. Ultra-High-Pressure minerals found in massive chromite of Luobusa Ophiolite, Tibet.
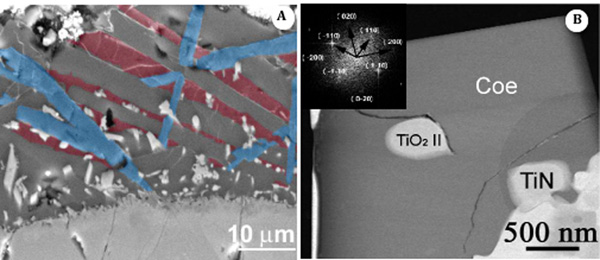
(A) SEM false-color image of prisms of coesite (gray) and kyanite (blue) with interstitial unknown amorphous phase (red) attached to pellet of Ti-Fe alloy (light gray). (Modified after Yang et al., 2007). (B) TEM bright field image of FIB-prepared foil of material in (A): A single crystal of TiO2 II is included in coesite (Coe); at the lower right corner are inclusions of TiN (osbornite). The insert is an electron diffraction pattern of the crystal of TiO2 II calculated from a high-resolution image by Fast Fourier Transform (Modified after Dobrzhenitskaya et al., 2009).
Additional investigation of this specimen by transmission electron microscopy (TEM) by Dobrzhinetskaya et al. (2009) confirmed the presence of coesite. Furthermore, included within the coesite is abundant titanium nitride (osbornite) and the cubic (high-pressure) form of boron nitride (BN). This was the first finding of nitrides in mantle rocks. Osbornite has been found only once previously on Earth but is common in meteorites, especially iron meteorites; BN had not been found in nature previously. Lastly, and perhaps most importantly, two observations point to a very high pressure history of the chromite. The coesite also contains inclusions of: (i) TiO2 II, the high-pressure polymorph of rutile with the alpha lead oxide (αPbO) structure (Figure 3b); (ii) native iron.
The presence of TiO2 II confirms the earlier implication that the coesite in this rock originally crystallized as stishovite. That is because the phase boundary between rutile and its high-pressure polymorph has a very steep positive slope; the intersection of that boundary with that for coesite-stishovite occurs at ~9.2 GPa, 1220˚C (Withers et al., 2003). A likely minimum temperature for upwelling upper mantle material beneath an ocean ridge is 1300˚C, which would require a depth in excess of 300 km to stabilize TiO2 II, well into stishovite stability. The presence of native iron is also critically important because it requires very low oxygen fugacity. Nevertheless, the ferric/ferrous ratio in the host massive chromite is elevated whereas the nodular and disseminated chromite of the ophiolite have normal Fe3+ content (Ruskov et al., 2010). This paradox is resolved by the hypothesis that this is the first naturally-occurring example of disproportionation of ferrous ion into ferric ion plus native iron at very high pressures induced by the mineral assemblage being dominated by phases that have no place in their structures for the ferrous ion (see extended discussions in Dobrzhinetskaya et al., 2009 and Ruskov et al., 2010).
A critical question arises following the remarkable observations described in the four previous paragraphs: “Is the Luobusa ophiolite unique in displaying a high-pressure highly reduced mineral assemblage in Fe3+-rich massive chromite or is there a subset of ophiolites that carries this very high-pressure signal?” The data to answer this question remain few but they are sufficient to confirm that the Luobusa ophiolite is not unique. Diamonds and silicon carbide have been reported from an ophiolite in the polar Ural mountains (Trumbell et al., 2009) and several ophiolites display elevated ferric/ferrous ratios in massive chromite (see references and discussion in Ruskov et al., 2010).