Continental Collision Zones
Alpe Arami, Switzerland
There is a large and sometimes acrimonious literature concerning the first discovery of rocks exhumed from great depth. Microstructures in the Alpe Arami peridotite of southern Switzerland (Figure 1) reflect events that were originally proposed to have occurred at depths exceeding 300 km (Dobrzhinetskaya et al., 1996; 2000; Bozhilov et al., 1999; 2003) but that eventually could be ascribed to depths approaching 400 km (Liu et al., 2007a). The critical observations are exsolution of ilmenite rods plus spinel flakes in olivine (Figure 1a,b) and exsolution of high-pressure clinoenstatite/pigeonite in diopside (Figure 1c) in the same rocks, in crystals separated only by 10s to 100s of microns. Although alternative interpretations of these observations were cited in rebuttal to the original publications, no alternative interpretations have been published that can offer a viable interpretation of both observations with a single alternative model. Although the olivine observations were center stage during most of the discussions in the literature, the pyroxene observations turned out to be definitive because a second instance of high-pressure clinoenstatite/pigeonite exsolution from diopsidic pyroxene in the Bixiling complex of the Dabie Mountains UHPM terrane in eastern China (Liu et al., 2007a), coupled with high-pressure experimental studies (Tribaudino et al., 2000; 2002; Nestola et al., 2004), allowed unambiguous identification of the clinoenstatite lamellae in diopside as having been precipitated as the high-pressure polymorph.
Figure 1. Optical micrographs of ilmenite and chrome spinel precipitates
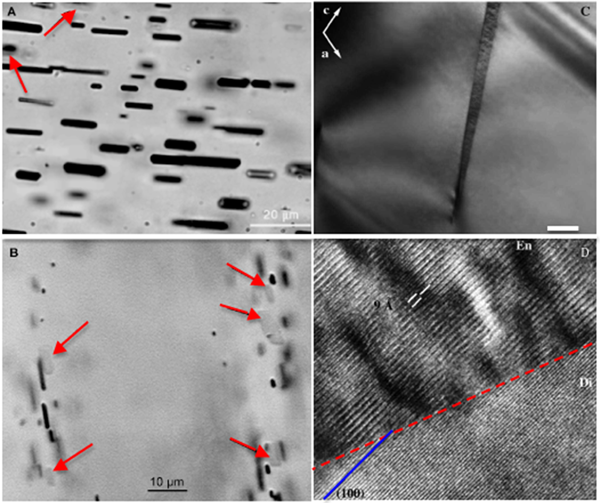
Optical micrographs of ilmenite and chrome spinel precipitates in first-generation Alpe Arami olivine (A&B) and Transmission electron micrographs of precipitates of high-pressure clinoenstatite/pigeonite in diopside (C&D) A. Ilmenite rods (black) are oriented parallel to [010] of olivine; spinel platelets (gray; difficult to see in this image) are oriented parallel to (100) of olivine. Two spinels that are in focus and attached to ilmenite rods are indicated by red arrows (see Dobrzhinetskaya et al., 1996; Green et al., 1997). B. Precipitates are in a few cases concentrated on (001) planes, but exhibit the same topotaxy as in A. In this image, virtually all ilmenite rods have a spinel platelet attached; arrows point out spinels without ilmenite (see Bozhilov et al., 2003). C. Precipitate of clinoenstatite in diopside. Note large angle between c-axis of diopside and the lamella. D. High resolution image viewed down the b-axis showing (100) lattice fringes in both host (Di) and precipitate (En) and the boundary between them (outlined by red dashed line). Angle between blue and red lines is the angle between the lamella boundary and the c-axis of diopside. Short white lines show the ½ unit cell offset across antiphase boundaries in the lamella. The antiphase domains and the angle between the c-axis and the lamella unambiguously demonstrate that the originally precipitating phase was high-pressure clinoenstatite/pigeonite and that it precipitated at approximately 400 km (see Bozhilov et al., 1999 and Liu et al., 2007a for discussion). Panel A is modified after Figure 1 of Green et al. (1997); Panel B is modified after Figure 3C of Bozhilov et al. (2003); panels C&D are modified after Figure 1C and Figure 3 of Bozhilov et al., 1999, respectively.
Realization that the pressure of exsolution could be estimated from the orientation of the lamellae then allowed deduction that the Bixiling complex (commonly interpreted to have originated as shallow ultramafic cumulates) was subducted to more than 300 km and returned to the surface and that the Alpe Arami peridotite was exhumed from perhaps as great as 400 km. In the case of Bixiling, the accompanying quartzofeldspathic host rocks have been determined to have been similarly exhumed from great depth based on abundant coesite inclusions in zircons. For Alpe Arami, the accompanying eclogites also have been shown to have a UHPM history (Dobrzhinetskaya et al., 2002) but the surrounding Lepontine gneisses have not been systematically examined for zircon inclusions. Nevertheless, there is no alternative carrier for the garnet peridotites and eclogites included within the Lepontine gneisses.
Alten Tagh, China
Although it may be ambiguous whether or not the Bixiling ultramafic rocks have a low-pressure history that preceded their subduction to great depths, such is not the case for pelitic gneisses from the Alten Tagh UHPM terrane of western China in which rods of kyanite and spinel were precipitated in stishovite (Liu et al., 2007b). In those rocks, abundant groups of rods of kyanite and spinel in polycrystalline quartz domains exhibit exsolution morphology but cross high-angle boundaries without deflection (Fig. 2a). The abundance of the rods is much more than could be dissolved in quartz or coesite but consistent with stishovite. Moreover, and most importantly, the rods in each polycrystalline quartz domain have orientations requiring that the host from which they precipitated had tetragonal symmetry (4/m 2/m 2/m) consistent with stishovite (Fig. 2b). The inferred topotaxy between the precipitates and host is also consistent with stishovite structure. The deduction that the rods precipitated within stishovite requires that the rocks began their return to the surface sufficiently deep that the solubility of kyanite and spinel components could exsolve at the amounts observed before leaving the stishovite stability field. That, in turn, requires that the upward journey began at 350km or more.
Figure 2. Optical micrograph of kyanite and spinel needles
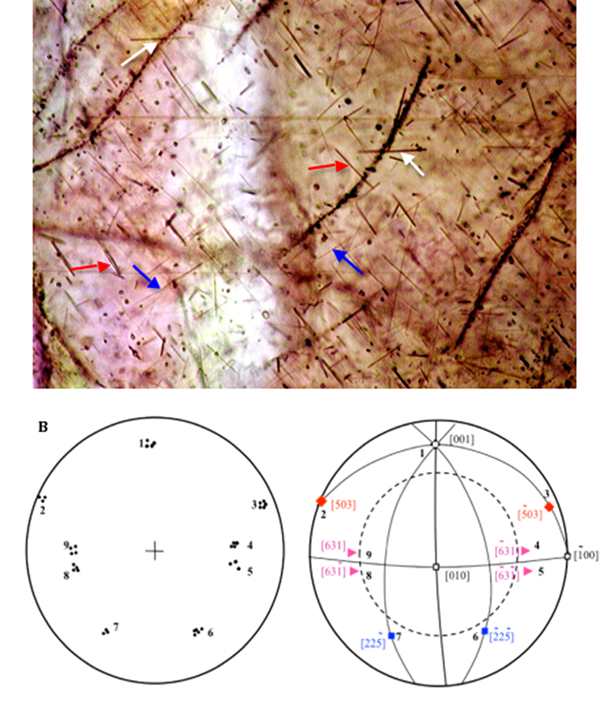
Optical micrograph of kyanite and spinel needles crossing high-angle quartz grain boundaries (A) and stereographic projections of needle orientations (B). (A) Bright band is a left-dipping high-angle grain boundary viewed between crossed polarizer’s (see Liu et al., 2007b for the EBSD-measured orientations of these two crystals) showing multiple sets of needles crossing the boundary undisturbed (most abundant group trends NE (red arrows); two other groups shown by white and blue arrows). There are more groups; can you find them? (B) Left diagram shows that needle orientations form tight clumps. The most abundant orientation (#1) is assumed to be the c-axis because that is the only unique direction in tetragonal crystals. Right diagram shows that the 9 groups imply 4 mirror planes through the c-axis and one normal to the c-axis, establishing that the rods exsolved from a host with tetragonal symmetry. Dotted circle marks 30˚ to the thin section, beyond which Universal stage measurements cannot establish orientations (See Liu et al., 2007b for further explanation). Figures modified after Liu et al. (2007b).