The Quaternary Magmatic events: Roman and Lucanian Magmatic provinces
Early in the twentieth century, Washington (1906) defined the Roman Magmatic Province as a unique magmatic association characterized by leucite-bearing ultrapotassic volcanic rocks, extending from Northern Latium to the Neapolitan area. Since then several authors re-defined the Roman Province limits on the basis of different criteria (e.g., Hawkesworth & Vollmer, 1979; Vollmer & Hawkesworth, 1980; Ayuso et al., 1998; Paone, 2004; Peccerillo, 2005a). In the present paper we keep the original definition using the petrographic criterium adopted by Washington (1906) and later resurrected by Turner & Verhoogen (1960), because this is coherent with an easy recognition from other timely close volcanic association worldwide, and because this division correlates with main mechanisms of magma genesis and geodynamic events in the area (Conticelli et al., 2004; Avanzinelli et al., 2009). Indeed the Roman Province is characterized by Pleistocene to Holocene leucite-bearing ultrapotassic igneous rocks, whereas the Tuscan Magmatic Province by Pliocene to Pleistocene leucite-free ultrapotassic igneous rocks, the Lucanian Magmatic Province by Pleistocene haüyne/leucite-bearing ultrapotassic rocks. This would be a simple, but consistent, criterium to assess magmatic domains. With an eye to the geochemical and isotopic characteristic we further divide internally the Roman rocks between the Latian districts, in which ultrapotassic leucite-bearing volcanic rocks dominate over shoshonites, and the Neapolitan one, where shoshonite volcanic rocks are more abundant than ultrapotassic leucite-bearing volcanic rocks (Fig. 11).
The Roman Magmatic Province
The Roman Magmatic Province comprises several volcanic districts that can be made up by a cluster of two/three volcanic apparata (e.g., Vulsini, Sabatini, and Neapolitan, Fig. 11), by a monogenetic volcanic field (e.g., Middle Latin Valley, and Campi Flegrei, Fig. 11), or just by a single volcanic apparatus with a summit caldera and a post-caldera monogenetic activity (e.g., Vico, Colli Albani, and Roccamonfina volcanoes, Fig. 11). The Roman Magmatic Province, in Northern Latium, overlaps the volcanic rocks belonging to the Tuscan Magmatic Province (Fig. 11). The volcanism of the Roman Magmatic Province begun at about 760 ka (Florindo et al., 2007) and protracted till the present time, being the last eruption at Vesuvius of 1944 A.D., with a maximum production of volcanic rocks between 400 and 200 ka in the Latian districts and between 200 ka and the present for the Neapolitan district. Minor centers of the Roman Magmatic Province are found in Umbria, in an intramontane region, at San Venanzo and Cupaello.
The Latian districts
The Latian volcanic districts occupy prevalently the Latium region although the Roccamonfina volcano is well within the campanian region. They have been active coevally during the Pleistocene and presently most of them are just quiescent. On the basis of petrographic data the Latian districts are prevalently made up by leucite-bearing ultrapotassic magmas, in some cases preceeded by hybrid Tuscan-Roman magmas, as in the cases of early activity at Vulsini, Vico, Sabatini (see below), and followed by less alkaline leucite-bearing and leucite-free shoshonitic volcanic rocks in the post caldera activity. The Leucite-bearing ultrapotassic magmas are predominat over the late leucite-free magmas (i.e., shoshonitic to calc-alkaline magmas; Avanzinelli et al., 2009, and references therein).
1. Vulsini district
The Vulsini district is the northernmost volcanic cluster of the Roman Province and it is formed by the coalescence of four large volcanic apparata: the Palaeo-Bolsena, Bolsena, Montefiascone and Latera volcanoes (Nappi et al., 1987, 1998; Palladino et al., 2010) (Fig. 15). The volcanic rocks cover an area larger than 2,000 km2, filling up a depressed area represented by the Siena-Radicofani and Paglia-Tevere grabens. The coalescence of the four volcanic apparatus formed a shield-like area with a central depressed zone occupied today by the Bolsena lake (Fig. 15). All four volcanic complexes are made up mainly of ignimbrites with subordinate lava flows, although a lava plateau has been recorded in the early history of the Latera volcano (e.g., Conticelli et al., 1987, 1989; Vezzoli et al. 1987).
Figure 15. Geological sketch map of the Vulsini district (Roman Magmatic Province).
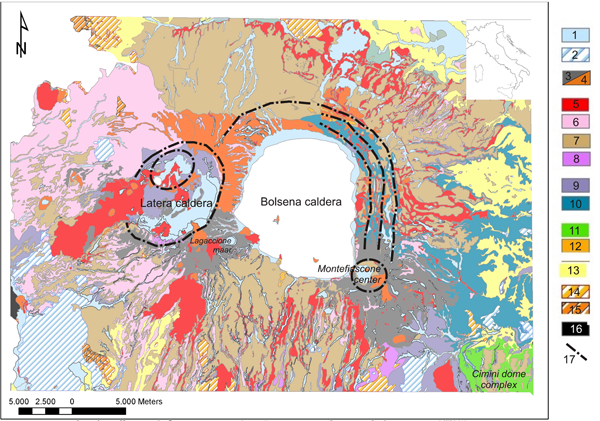
Geological sketch map of the Vulsini district. Legend: 1) Holocene alluvial and lacustrine deposits; 2) travertine; 3-9) Bolsena, Latera and Montefiascone calderas; 3) scoria cones; 4) phreatomagmatic deposits; 5) leucite-bearing lavas; 6) pozolanaceous ignimbrites; 7) fallout and reworked pyroclastics; 8) spatter-rich ignimbrites; 9) lithoidal ignimbrites; 10) PaleoBolsena volcano welded to unwelded ignimbrites and leucite-bearing tuffs; 11) rhyodacite Peperino Tipico ignimbrite (Cimini dome complex); 12) rhodacitic domes; 13) post-orogeny Pliocene-Pleistocene marine sediments; 14) Allochtonous Flysch; 15) Meso-Cenozoic pre-syn-orogenic successions; 16) Paleozoic basement; 17) caldera.
Palaeo-Bolsena volcanic apparatus has been supposed to be the oldest volcanic center and it is made up by the large trachytic, partly welded, ignimbrite called “Nenfro”, associated to leucititic to tephri-phonolitic lava flows and plinian pyroclastic fall horizons (Nappi et al., 1987, 1991, 1994, 1995). Volcanic activity begun with a plinian fall layer dated at 0.59 Ma (40Ar-39Ar, Barberi et al., 1994) and 0.58 Ma (K/Ar, Nappi et al., 1995). Several conflicting age data are published on “Nenfro” ignimbrite that likely caused the Paleo-Bolsena calderic collapse: 0.88 to 0.4 Ma (K/Ar, Nicoletti et al., 1981), 0.5 Ma (40Ar-39Ar, Barberi et al., 1994) and 0.51 Ma (K/Ar, Nappi et al., 1995). Single crystal, laser total fusion 40Ar-39Ar dating performed on a new mineral separation from the original six samples of Barberi et al. (1994) gives a well constrained age for this formation of 0.498 Ma (Laurenzi & Deino, 1996). The Bolsena volcanic complex begun its activity after the formation of the Palaeo-Bolsena caldera and lasted for few hundred thousand years (Palladino et al., 2010, and references included). It developed mainly in the eastern sector of the Vulsinisan district with the formation of a large caldera depression partly occupied by the Bolsena Lake, and produced two thick ignimbrite sheets, fall deposits and lava flows which built an ignimbrite shield (Freda et al., 1990; Nappi et al., 1998; Palladino et al., 2010). Fissural lava flows formed a lava plateau south of Bolsena Lake in the Marta-Tuscania area (Palladino et al., 1994). The Montefiascone volcano (~0.29 - ~0.23 Ma: Nappi et al., 1995; Brocchini et al., 2000) evolved within the period of activity of Bolsena and partially overlapped with the Latera Volcano, developed in the western sector (~0.28 - ~0.15 Ma: Metzeltin & Vezzoli, 1983; Turbeville, 1992). Both Montefiascone and Latera volcanoes are characterized by different activities that brought to the formation of a small stratovolcano with a small summit caldera (ca. 2.5 km wide) at Montefiascone, compared to a large flat ignimbritic volcanic plateau with a large central polyphasic nested caldera (ca. 9 km wide) at Latera (Sparks, 1975; Varekamp, 1980; Conticelli et al., 1986, 1987, 1991; Vezzoli et al., 1987; Coltorti et al., 1991; Turbeville 1992, 1993; Di Battistini et al., 1998, 2001). The final activity is represented by the Bisentina and Martana Islands, and likely other centers below the Bolsena Lake, tentatively still active around 0.13 Ma (K/Ar, Nappi et al., 1995).
The volcanic products are mainly characterised by leucite-bearing ultrapotassic rocks (Tables 4 and 5) with few leucite-free shoshonitic rocks confined in the post-caldera activity of the Latera volcano (Table 6; e.g., Conticelli et al., 1991) and some melilite-bearing leucitites (kamafugites) in the early stages of the Montefiascone volcano (di Battistini et al., 2001).
The ultrapotassic rocks range in composition from leucite-bearing basanites, leucitites, tephrites, phonolitic-tephrites, tephritic-phonolites, and phonolites (Fig. 16a). Extremely differentiated products dominated volumetrically over mafic terms, but in some cases syn-depositional formation of analcite after leucite allow the K2O and alkalis loss, a characteristic capable to drive the juvenile
Figure 16. Classification and incompatible trace element characteristics of Pleistocene volcanic rocks from Monti Vulsini volcanoes.
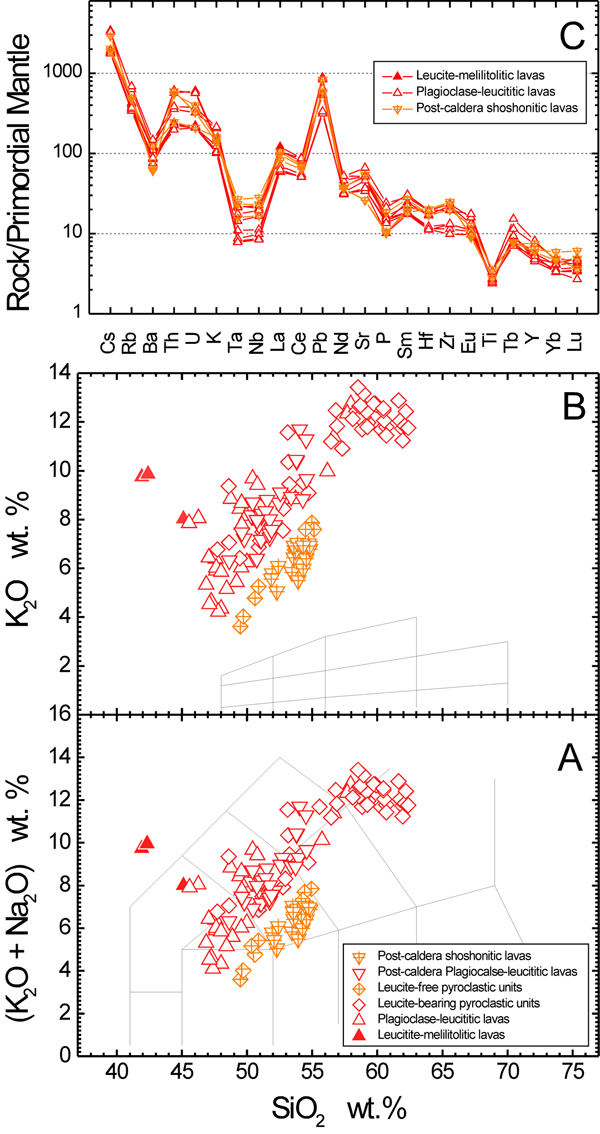
Classification and geochemical characteristics of the Vulsini leucite-bearing ultrapotassic rocks and associated shoshonitic ones. A) Total Alkali-Silica (TAS) classification diagram (Le Maitre, 2002). B) K2O wt.% vs. SiO2 wt.% classification diagram with reported the grid for orogenic volcanic rock suites (Peccerillo & Taylor, 1976). C) Incompatible trace element patterns for mafic volcanic rocks normalised to the primordial mantle values of Sun & McDonough (1989). Data from Holm et al. (1982); Civetta et al. (1984); Rogers et al. (1986); Conticelli et al. (1987, 1991, 2002, 2007); Coltorti et al. (1991); Di Battistini et al. (2001, 2002); Gasperini et al. (2002).
clasts (pumice) in ignimbrite toward a trachy-phonolitic to trachytic compositions (Conticelli et al., 1987; Parker, 1989) (Fig. 16a). The most mafic compositions are always found among lava flows and they are found mainly in the plateau-like structure of the southern sector of the Vulsinian district and of Montefiascone volcano where leucite-bearing basanitic to tephritic lavas do occur (Civetta et al., 1984; Rogers et al., 1985; Conticelli et al., 2002). Post caldera activity was particularly intense at the Latera volcano with bimodal magmatism both leucite-bearing and –free lavas (Fig. 15). Leucite-bearing post-caldera Latera lavas have compositions ranging from tephritic to tephri-phonolitic (Fig. 16a), whereas leucite-free lavas are particularly abundant within and external to the caldera in the southern eastern sector of the volcano with the Selva del Lamone lava flow (Fig. 15) and they have a clear shoshonitic affinity with lavas ranging in composition from K-trachybasalts to latites (Figs. 16a,b).
The shoshonitic trachy-basalts have mineralogy assemblages made up of abundant olivine, clinopyroxene and plagioclase, but they differ significantly in terms of geochemistry with respect to other post-caldera shoshonitic rocks from other volcanic districts of the Roman Province (e.g., Holm et al., 1982; Varekamp & Kalamarides, 1989; Conticelli et al., 1991, 2009b; Turbeville, 1993). The most abundant mafic mineral in the Roman rocks is the clinopyroxene, which differ significantly from volcanic rocks crystallized in equilibrium with leucite or not. Indeed, clinopyroxene from lamproite-like ultrapotassic rocks are generally aluminium poor, with Si+Al not sufficient to fill completely the tetrahedral site, whereas clinopyroxene from Roman rocks, either leucite-free or –bearing ones, are characterized by excess Al that patitioned between tetrahedral and octahedral sites (Barton et al., 1982; Holm, 1982; Cellai et al., 1994; Bindi et al., 1999; Chelazzi et al., 2006). Clinopyroxene in shoshonite from Latera volcano are transitional between those typical of leucite-free (Tuscan lamproites) and Roman rocks, whereas those from shoshonites in other Roman districts have also Al excess (Perini & Conticelli, 2002; Boari & Conticelli, 2007; Cellai et al., 1994). Leucite is found both as phenocryst and as groundmass phase in plagioclase leucitites and leucitites (Fig. 3h). Sanidine is present both in the most evolved phonolitic and trachy-phonolitic terms, and it is also found also in leucite-free rocks of the Palaeo-Bolsena volcano and of the post-caldera Latera volcano.
Apparently normalized incompatible trace element patterns of plagioclase leucititic rocks of the Vulsinian district are similar to those of earlier magmatisms (Fig. 16c) characterized by leucite-free ultrapotassic rocks (i.e., lamproite) and associated shoshonites and calc-alkaline rocks (i.e. Western Alps, Western Tyrrhenian Sea, Tuscany). Vulsinian plagioclase leucititic rocks and associated shoshonites, however, display larger throughs at Nb, Ta, and Ti, the appearance of a small through at Hf, and a small peak at Sr, concomitantly to the inversion of the U/Th and Ta/Nb normalized ratios (Fig. 16c), with respect to the older leucite-free ultrapotassic and associated shoshonites and calc-alkaline rocks (Figs. 8c, 10c, 12c).
2. Vico district
The Vico district consists of a single Pleistocene volcanic edifice built in the form of a conic stratovolcano cut by a summit polygenetic caldera (Mattias & Ventriglia, 1970; Perini et al., 1997, 2004). Post-caldera activity, subordinate in volume, both within and on the edge of the caldera do occur (Fig. 17; Perini et al. 1997, 2004). The Vico volcano like the other Roman volcanoes North of Rome (Fig. 11) developed within the NW–SE Siena-Radicofani and Paglia-Tevere extensional basin (Barberi et al., 1994), and it covers almost completely an older volcanic apparatus belonging to the Tuscan Magmatic Province, the Monte Cimino Volcanic Complex.
Figure 17. Geological sketch map of the Vico district (Roman Magmatic Province).
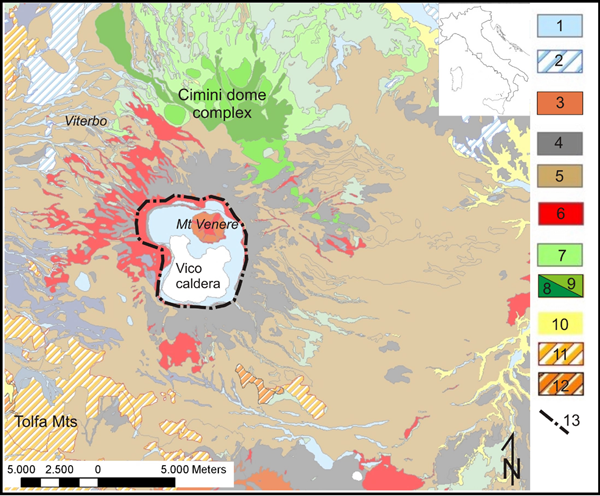
Geological sketch map of the Vico volcano. Legend: 1) Holocene alluvial and lacustrine deposits; 2) travertine; 3) Monte Venere period: post-caldera scoria cones (third period); 4-6) Lago di Vico period (second period): 4) Carbognano phreatomagmatic ignimbrite; 5) caldera forming phonolitic ignimbrites; 6) stratocone building leucite-bearing lavas; 7-9) Cimini dome complex (1300-900 ka): 7) rhyodacitic Peperino Tipico ignimbrite; 8) rhyodacitic domes; 9) lamproitic lava; 10) post-orogeny Pliocene-Pleistocene marine sediments; 11) Allochtonous Flysch; 12) Macigno Flysch; 13) caldera.
The stratigraphy of Vico volcano is divided into three main rock successions: the Rio Ferriera, the Lago di Vico, and the Monte Venere successions (Perini et al., 1997, 2004). The early activity, during the Rio Ferriera period (Ist) produced pyroclastic fall and flow units interbedded to minor lava flows (Cioni et al., 1987; Barberi et al., 1994; Perini et al., 1997, 2000). The onset of the Rio Ferriera period is constrained by the age of the basal plinial fall at about 0.42 Ma (Sollevanti, 1983; Barberi et al., 1994). All K/Ar model ages older than 0.42 Ma (Nicoletti, 1969) are clearly biased. The Lago di Vico period (IInd) involved an early stratovolcano-building phase (0.30–0.26 Ma; Sollevanti, 1983; Laurenzi & Villa, 1987; Barberi et al., 1994) with emplacement of leucite-bearing lava flows (~ 50 km3; Bertagnini & Sbrana, 1986; Perini et al., 1997, 2004). Villemant & Fléhoc (1989) report a K/Ar age of 0.18 Ma and a U-Th isochron age of 0.21 (+0.028/-0.022) Ma for the final episode of the stratovolcano building period. Towards the end of the Lago di Vico period four explosive ignimbrite-forming eruptions caused the destruction of the Vico edifice and the formation of an 8 km diameter polygenetic caldera (Perini et al., 1997, 2004). These were the Farine, Ronciglione (0.16 Ma), Sutri (Tufo Rosso a scorie nere, 0.15 Ma) and Carbognano (0.14 ka) eruptions (Sollevanti, 1983; Laurenzi & Villa, 1987; Barberi et al., 1994; Perini et al., 1997, 2004; Bear et al., 2009a,b). The post caldera activity (i.e., Monte Venere period - IIIrd) comprises the so called “Tufi Finali”, several monogenetic cones, and minor lava flows. The Monte Venere is an intra-caldera cinder cone, whereas other three cinder cones are found along the northern caldera rim from Poggio Nibbio to Poggio Varo (Fig. 17). A small shoshonitic lava flow has been vented from a fracture on the caldera margin flowing down both into the caldera and along the external northern flank of the volcano (Perini et al., 1997, 2004). K/Ar ages comprised between 0.095 and 0.085 Ma are from Monte Venere rocks (Laurenzi & Villa, 1985; Villemant & Fléhoc, 1989); a 40Ar-39Ar age equal within error to K/Ar ones was obtained on scoriaceous deposits drilled into the Vico lake sediments (Magri & Sadori, 1999; Laurenzi, unpublished datum).
The Rio Ferriera volcanic rocks have a mild potassic nature, resembling closely previous Tuscan rocks of the underlying Cimino volcano, but leucite appears randomly. They range in composition from latite to trachyte and rhyodacite (Fig. 18a, 18b). Large sanidine phenocrysts are also found but they are not in equilibrium with Vico magmas, corroborating the hypothesis that Rio Ferriera formations represent hybrid rocks due to magma mixing between differentiated Tuscan high-K calc-alkaline magmas and newly arrived ultrapotassic silica-undersaturated leucite-bearing Roman magmas (Perini et al., 2000, 2003). The Lago di Vico period of activity was dominated by plagioclase-leucititic lavas and pyroclastic rocks, ranging in compositions from leucite-bearing tephrites to leucite-bearing phonolites, passing through phonolitic-tephrites and tephritic-phonolites (Fig. 18a). Syn-depositional alkali loss in pyroclastic rocks is observed at Vico volcano similarly to Vulsinian district (Fig. 18a).
Vico post caldera activity, analogously to what observed at Latera volcano, diplays a clear bimodal petrologic affinity (Tables 5 and 6). Leucite-bearing tephrites (Monte Venere scoria and lavas) beside leucite-free olivine latites with a shoshonitic affinity (Poggio Nibbio lavas) and olivine trachybasalts (Poggio Nibbio scoria), the latter showing seldom leucite both as phenocrysts and in the groundmass, occur in the post caldera period (Fig. 18a).
Normalized incompatible trace element patterns of leucite-bearing Vico rocks differs significantly from those of Vulsinian ones (Fig. 18c). Apart the general pattern with HFS elements fractionated with respect to LIL elements, which is a general rule for the overall western Mediterranean ultrapotassic and associated rocks (e.g., Conticelli et al., 1986, 1997, 2002, 2004, 2007, 2009a), the Vico rocks show larger troughs at Ba, P, and Ti but smaller ones at Ta and Nb, with larger peaks at Pb than Vulsinian plagio-leucititic rocks. Other differences with Vulsinian rocks are observed in the normalized Th/U, Ta/Nb, Nd/Sr, Zr/Hf ratios (Fig. 18c). Fractionation of Th/U has been found to be a peculiar characteristic of the Vico volcano with respect to the other Roman volcanoes (Villemant & Palacin, 1987; Villemant & Fléhoc, 1989; Avanzinelli et al., 2008).
Figure 18. Classification and incompatible trace element characteristics of Pleistocene volcanic rocks from Vico volcano.
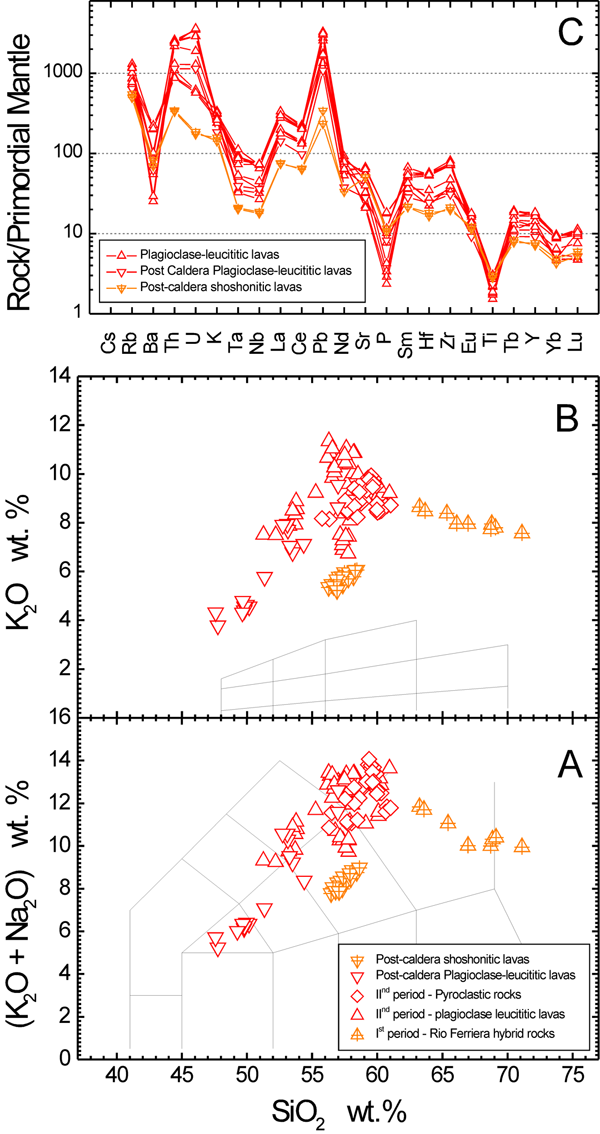
Classification and geochemical characteristics of the Vico leucite-bearing ultrapotassic rocks and associated shoshonitic ones. A) Total Alkali-Silica (TAS) classification diagram (Le Maitre, 2002). B) K2O wt.% vs. SiO2 wt.% classification diagram with reported the grid for orogenic volcanic rock suites (Peccerillo & Taylor, 1976). C) Incompatible trace element patterns for mafic volcanic rocks normalised to the primordial mantle values of Sun & McDonough (1989). Data from Barbieri et al. (1988); Perini et al. (1997, 2000, 2003, 2004).
3. Sabatini district
The volcanic style of the Sabatini volcanoes was mainly explosive but lava flows are also abundant and vented from several different centers spread out over the entire district area (Fig. 19; Conticelli et al., 1997). There are three caldera depressions, which are from east to west: Sacrofano, Baccano and Bracciano caldera (Fig. 19; De Rita et al., 1983, 1988 1993a, 1993b; De Rita & Zanetti, 1986; De Rita & Sposato, 1986). The Sabatini district developed within the southeastern prosecution of the NW–SE Siena-Radicofani and Paglia-Tevere extensional basin (e.g., Baldi et al., 1974; Locardi et al., 1976; Barberi et al., 1994). Analogously to the Vico district also the Sabatini overlap partially, on its western portion an older volcanic complex belonging to the Tuscan Magmatic Province, which is the Tolfa-Ceriti Dome Complex (Figs. 11, 19), made of leucite-free shoshonitic to high-K calc-alkaline rocks belonging to the Pliocene lamproite-shoshonite-calc-alkaline suite of the Tuscan Magmatic Province (Fig. 12b). These pre-Sabatini volcanic rocks outcrop within the volcanic cover of the Sabatini district at Manziana and Monte Calvario areas, and define the southwestern and northwestern edges of the district (Fig. 19). On the basis of geological and volcanological records the volcanic activity of Sabatini district has been divided in five different periods (e.g., Conticelli et al., 1997; Karner et al., 2001a).
Figure 19. Geological sketch map of the Sabatini district (Roman Magmatic Province).
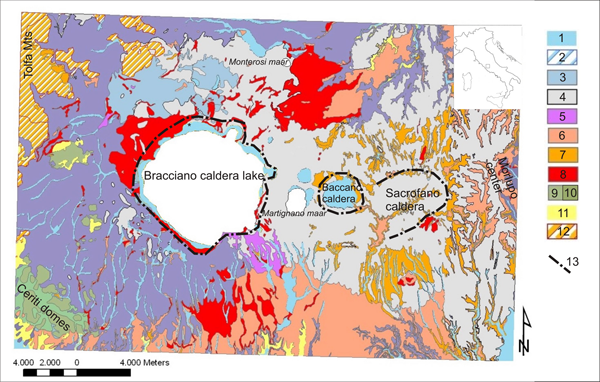
Geological sketch map of the Sabatini district. Legend: 1) Holocene alluvial and lacustrine deposits; 2) travertine; 3) scoria cones; 4) phreatomagmatic deposits from the final Sacrofano caldera phase and the recent maars; 5) pozolanaceous ignimbrites; 6) fall deposits; 7) lithoidal ignimbrites; 8) undersaturated lavas; 9-10) Manziana-Ceriti rhyodacitic domes, lavas and ignimbrites; 11) Messinian-Pliocene post-orogenic marine deposits; 12) Allochtonous Flysch.
All ages mentioned in the following discussion are 40Ar-39Ar ages, unless otherwise stated. Distal tephra layers probably related to the early Sabatini history, found in well cores, are dated between 0.8 and 0.76 Ma (Florindo et al., 2007), followed by other pyroclastic deposits, always in distal sections, that cover the time span from 0.65 Ma (Karner et al., 2001a) till the emplacement of Morlupo block and ash deposit (0.59 Ma, Cioni et al., 1993). Continuous volcanic activity, characterized by large volumes of products, started at about 0.59 Ma and lasted till about 0.4 Ma. The activity of the first period continued in the eastern sector (Fig. 19) with the emplacement of the “Tufo Giallo della Via Tiberina” ignimbrite (0.55 Ma, Karner et al., 2001a), and then moved also to the western sector of the district where strong parossistic eruptions produced the “Prima Porta” ignimbrite (0.51 Ma: Karner et al., 2001a), the “Grottarossa ” pyroclastic sequence (0.52 Ma: Karner et al., 2001a), the “Tufo Terroso con Pomici Bianche” (0.49 Ma: Karner et al., 2001a), the “Tufo Grigio Sabatino” (also called “Tufo Rosso a Scorie Nere Sabatino”) a red tuff with black pumice (0.43 Ma, K/Ar: Evernden & Curtis, 1965; 0.45 Ma: Cioni et al., 1993, Karner et al., 2001a), the “Peperini Listati” ignimbrite (0.45 Ma: Cioni et al., 1993). The third period of activity was mainly characterised by effusive eruptions with lava flows alternated to large volume of fallout and surges deposits, the latter grouped in the “Tufi Varicolori della Storta” (0.41 Ma: Karner et al., 2001a), and the “Tufi Stratificati Varicolori di Sacrofano” pyroclastic sequences (Fig. 19). The activity of this period was concluded by the “Tufo Giallo di Sacrofano” ignimbrite (0.29 Ma: K/Ar, Fornaseri, 1985; 40Ar-39Ar, Karner et al., 2001a; Sottili et al., 2010). During this period both the Bracciano and Sacrofano calderas were formed (De Rita et al., 1983, 1993a). The fourth period of activity produced several monogenetic centers widely distributed over the entire district (Fig. 19). Most of them produced a single lava flow associated to scoria cones. The most intense monogenetic activity was concentrated in the Rocca Romana - Trevignano area, in the north-western sector, but cinder cones have been also found in the eastern sector as well (Monte Maggiore, Colle Aguzzo, Casale Francalancia; Fig. 19), and at the border of the Bracciano caldera (Trevignano, Vigna di Valle). The fifth period of activity concentrated in the central sector of the district, in the area between the Bracciano and Sacrofano calderas (Fig. 19), with hydromagmatic activity that produced the tuff ring and tuff cones of Stracciacappe, Martignano, and Baccano. Age data just out clarify the more recent activity of this volcanic district, with the hydromagmatic centres concentrated at about 0.1-0.09 Ma (Sottili et al., 2010); these ages confirm the previous youngest recorded age, 0.09 Ma (K/Ar, Fornaseri, 1985a).
Apart the Morlupo trachyte, which represents a very small outcrop of hybrid products at the very beginning of activity, and the Vigna di Valle leucite-bearing latite, a Bracciano post caldera lava, the rest of the Sabatini volcanic rocks are made up exclusively by silica-undersaturated volcanic rocks (Cundari, 1979; Conticelli et al., 1997), ranging in composition from leucite-bearing tephrites to phonolites (Fig. 20a). Alkali and potassium lost (Fig. 20a,b) during syn-depositional transformation of leucite in analcite is observed in pyroclastic juvenile fragments of the Sabatini volcanoes (Parker, 1989). Clinopyroxene and leucite are the most abundant phenocryst phases with olivine restricted to the most evolved terms. Groundmasses are made up of leucite, clinopyroxene, plagioclase, nepheline, phlogopite, magnetite, and apatite. Titanite and haüyne have been found as accessory minerals in the most extreme differentiated phonolites (authors’ unpublished data). The Vigna di Valle lavas are the less silica undersaturated lavas, and beside the centimetric leucite megacrysts/xenocrysts the rocks is made up of hyalophane and Barium-phlogopite crystals as well. Clinopyroxene are salitic in composition with strong zoning and abundant aluminium, enough to fill completely the tetrahedral site and to partition it in the octahedral (Cundari & Ferguson, 1982; Dal Negro et al., 1985; Cellai et al., 1994).
Incompatible trace element contents of Sabatini volcanic rocks have similar distribution and fractionation to leucite-bearing rocks of other volcanoes of the Roman Province (Fig. 20c), with negative spikes at Ba, Ta, Nb, P, Hf, and Ti, and peaks at Rb, Pb, Sr. Although mostly ultrapotassic rocks are observed in the Sabatini volcanic activity, a slight decrease in K2O and incompatible element is observed passing from pre-caldera to post-calderas periods (Conticelli et al., 1997). The observed decrease in the total amount of incompatible trace elements at the same level of differentiation has been already seen
Figure 20. Classification and incompatible trace element characteristics of Pleistocene volcanic rocks from Sabatini volcanoes.
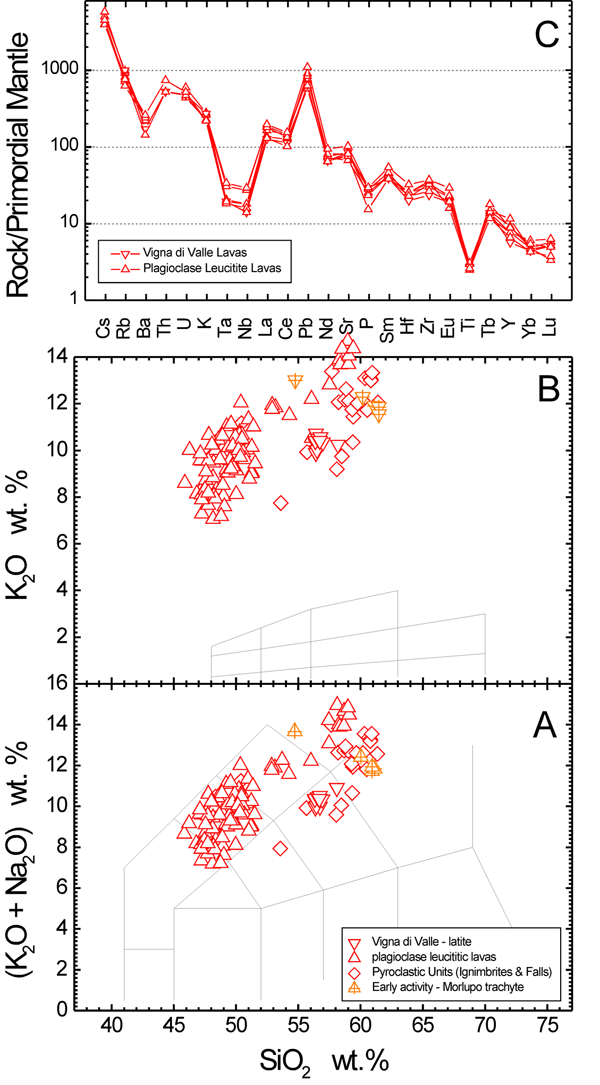
Classification and geochemical characteristics of the Sabatini leucite-bearing ultrapotassic rocks. A) Total Alkali-Silica (TAS) classification diagram (Le Maitre, 2002). B) K2O wt.% vs. SiO2 wt.% classification diagram with reported the grid for orogenic volcanic rock suites (Peccerillo & Taylor, 1976). C) Incompatible trace element patterns for mafic volcanic rocks normalised to the primordial mantle values of Sun & McDonough (1989). Data from Conticelli et al. (1997).
at the passage from pre- to post-caldera activity in the leucite-bearing series of Vulsinian volcanoes and Vico ones. In the latter cases, however, beside these leucite-bearing post-caldera series, leucite-free rocks have been erupted in the very last phases of volcanism. Indeed at Latera volcano (Vulsinian district) and at Poggio Nibbio (Vico district) leucite-free olivine-bearing shoshonitic trachybasalts are observed as the last eruption of the volcanic cycle in these two districts (Figs. 16 and 18). At the Sabatini district none of these mildly alkaline compositions have been observed, but it might not be excluded that magmas involved in the final hydromagmatic activity of Baccano-Martignano-Stracciacappe might have a shoshonitic affinity rather than ultrapotassic.
Figure 21. Geological sketch map of the Colli Albani (Alban Hills) district (Roman Magmatic Province).
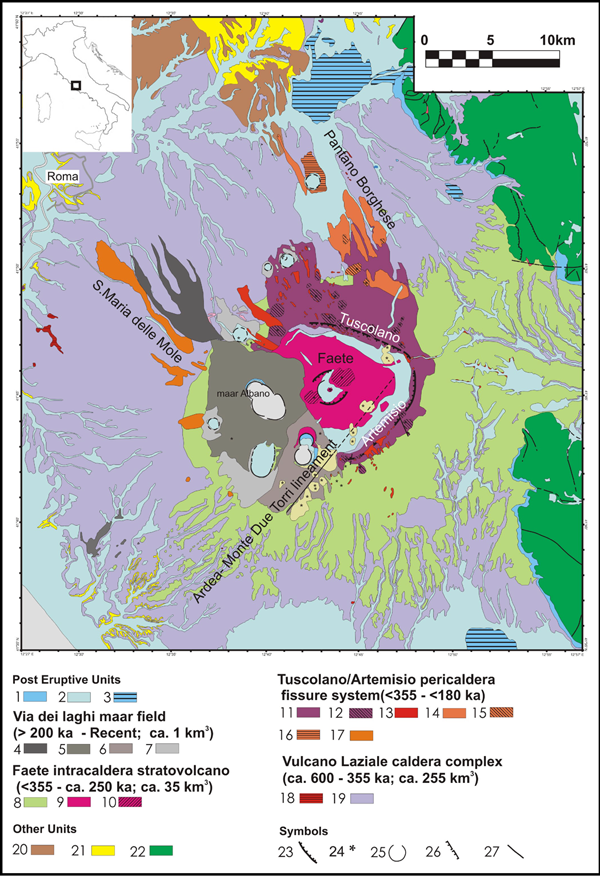
Geological sketch map of Colli Albani volcano with reported the three main periods of activity: I) Vulcano Laziale Period (pre-caldera), characterised by central activity with predominant paroxysmal explosive eruptions and fissural lava flows; II) Tuscolano Artemisio – Monte delle Faete period (post-caldera), characterised by the occurrence of six different sectors of volcanic activity: a) external to the caldera rim, with the Santa Maria della Mole lavas, the Pantano-Borghese centers and the Monte Due Torri cones and lavas; b) caldera-rim monogenetic activity that occurred along the northern and western edges of the Caldera (Tuscolano and Artemisio alignements); c) internal to the caldera, with the formation of the composite volcano of Monte delle Faete; III) The Via dei Laghi period, characterised by phreatomagmatic activity with little magmatic activity.
Legend: 1 & 2) alluvial deposits; 3) travertine; 4) Tavolato formation; 5) Albano maar; 6) Nemi maar; 7) other maars; 8) subplinian fall; 9) lower Faete succession; 10) upper Faete succession; 11) Tuscolano Artemisio (T.A.) scoria; 12) T.A. welded scoria; 13) T.A. lavas; 14 & 15) Pantano Borghese scoria & lavas; 16 & 17) Santa Maria delle Mole scoria & lava; 18 & 19) plateau lavas & ignimbrites; 20) Sabatini volcanic products; 21 & 22) sedimentary sequences; 23) caldera rim; 24) cinder cones; 25) maars; 26) inferred caldera rim; 27) fault. Re-drawn after Giordano et al. (2006), and Boari et al. (2009a).
4. Alban Hills (Colli Albani) district
The Colli Albani (Alban Hills) is a large flat stratovolcano with a central polygenetic caldera, and a dispersed post-caldera activity (Fig. 21). It was active from 0.6 Ma till recent times (e.g., Giordano et al., 2006, 2010; Marra et al., 2008, and references therein). It is located immediately south of Rome, some 15 km from the center of the city, on whose rocks the Ethernal city was built on and by (Funiciello et al., 2008). Volcanic activity occurred at the intersection of a NW-SE extensional basin, the Latin Valley, which represent the natural southward prosecution of the Siena-Radicofani-Paglia extensional basin, with a NE-SW and N-S systems of extensional and strike-slip faults (Faccenna et al., 1994a, 1994b, 1994c; De Rita et al., 1995). Volcanic rocks lie over Pliocene-Pleistocene marine sands and clays, and over the bordering horsts made of Triassic/Miocene platform carbonate units (Funiciello & Parotto, 1978; Danese & Mattei, 2010).
According to Giordano et al. (2006, 2010), volcanic activity of the Colli Albani volcano can be divided into three main periods: i) the “Vulcano Laziale” period, ii) the “Tuscolano Artemisio – Monte delle Faete” period, and iii) the “Via dei Laghi” period (Fig. 21). Polygenetic caldera collapse is the main volcano-tectonic characteristic delimiting the activity of the first period. The volume of the erupted volcanic rocks decreases greatly with time: from some 300 km3 during the first period to ca. 1 km3 during the last period. The Vulcano Laziale volcanic edifice cover an area of about 1,600 km2, with an ignimbrite plateau associated with a piece-meal caldera complex, with over 300 km3 of dominantly pyroclastic products and subordinate lava (Fornaseri et al., 1963; De Rita et al., 1995; Giordano et al., 2006, 2010). Early volcanic activity generated the Pisolitic Tuffs succession (0.6-0.5 Ma; De Rita et al., 2002 and reference therein), which is interbedded with distal early pyroclastic from Sabatini volcanoes (Karner et al., 2001a, 2001b; Marra et al., 2009). All ages mentioned in the following discussion are 40Ar-39Ar ages, unless otherwise stated. The largest volumetric units are represented by the Pozzolane Tuffs succession (0.46-0.35 Ma, Giordano et al., 2010 and references therein), a sequence of three large ignimbrites with intermediate compositions (Fig. 23a). The "Pozzolane Rosse" (0.457 Ma, Karner et al., 2001a), the "Pozzolane Nere" (0.407 Ma, Karner et al., 2001a; 0.405±0.003 Ma, K/Ar, Karner et al., 2001c) and the "Tufo Lionato-Pozzolanelle" are the main ignimbrites (Watkins et al., 2002; Giordano et al., 2006, 2010). The "Tufo Lionato-Pozzolanelle" forms the "Villa Senni" Formation, related to the last caldera collapse of the Colli Albani volcano, and responsible for the present day configuration of the caldera. Several ages are present in literature for the "Tufo Lionato" (0.355 Ma, Karner et al., 2001a; 0.365 Ma, Marra et al., 2009) and "Pozzolanelle" [0.35±0.03 Ma, average of 3 Rb/Sr isochron ages, and 0.34 (0.35 Ma updating the age of the used monitor) ±0.007 Ma, average of 7 40Ar-39Ar ages on leucites, Radicati et al., 1981; 0.357 Ma, Karner et al., 2001a]. Indeed, today the caldera has an 8 km diameter and it is asymmetric with a horse-shaped wall. Between major caldera forming eruptions volcanic activity was intra-caldera and localised along peri/extra-caldera fissures: the Vallerano lavas (0.46 Ma, Bernardi et al., 1982, K/Ar; Karner et al., 2001a, 40Ar-39Ar), Corcolle and Fontana Centogocce units, which, are dominantly made up of tephra fall units and lava flows.
With the formation of the caldera in its present day morphology, the volcano changed drastically its volcanic style and feeding system, with arrival to the surface of magmas through several different pathways. Lavas of the post caldera period have been dated between 0.35 and 0.15 Ma (K/Ar, Bernardi et al. 1982; 40Ar-39Ar, Karner et al. 2001a, Marra et al. 2003). Five main sectors of post-caldera vocanic activity have been recognized (Giordano et al. 2006, 2010 and Boari et al., 2009a) and produced ca. 40 km3 of erupted products: i) the Monte delle Faete, which is a stratovolcano (944 m a.s.l.) built up in the middle of the caldera; ii) the Tuscolano Artemisio composite edifice, which is made of coalescent scoria cones and lava flows aligned along circum- and extra-caldera fracture; iii) the Pantano Borghese monogentic alignement, which is external to the caldera rims on the NE sector of the district; iv) the Santa Maria della Mole monogenetic alignement, which is external to the caldera rims on the NW sector of the district; v) the Monte Due Torri-Ardea monogenetic alignment, which is made of an alignement of scoria cone across the caldera alon a NE-SW trending fracture.
The most recent period of volcanic activity (i.e., “Via dei Laghi” period) was concentrated on the south-western flank of the volcano (Giordano et al., 2006; Freda et al., 2006) with prevalent but small phreatomagmatic eruptions (Fig. 21), for the interaction with shallow productive aquifers (e.g., De Benedetti et al., 2008). The phreatomagmatic activity brought about the disruption of the SW rim of the caldera, with the formation of several coalescent maars aligned along both NNW-SSE and NS regional trends (e.g., de Rita et al., 1988, 1995; Funiciello et al., 2003). The temporal extent of the last activity of the Alban Hills has been matter of debate for years and involved U/Th and 40Ar-39Ar datings: an experiment performed with the two methods on the same samples gave on average discordant ages, being U/Th data below ~ 0.025 Ma, noticeably younger than 40Ar-39Ar data (Villa, 1992; Voltaggio et al., 1994). The polygenetic Albano maar is the last formed and produced phreatic activity since about 0.07 Ma (Marra et al., 2003; Freda et al., 2006; Giaccio et al., 2009). The end of the Albano activity is debated too: Freda et al. (2006) and Giaccio et al. (2009) established the last volcanic episode at ~0.04 and ~0.03 Ma, respectively, while other authors extend the activity throughout the Holocene (Villa et al., 1999; Funiciello et al., 2003; De Benedetti et al., 2008).
The most stricking feature of the Colli Albani rocks is their more silica-undersaturated character with respect to the other leucitites and plagio-leucitites of the Roman Province (Fig. 22a, 22b), which is accompayned by higher MgO and CaO contents of volcanic rocks (Tables 4 and 5). Also the four main ignimbrites have chemical compositions of the juvenile fragments, representing the composition of the magma triggering the eruption, never exceeding tephritic-phonolite compositions, but with
Figure 22. Classification and incompatible trace element characteristics of Pleistocene volcanic rocks from Colli Albani volcano.
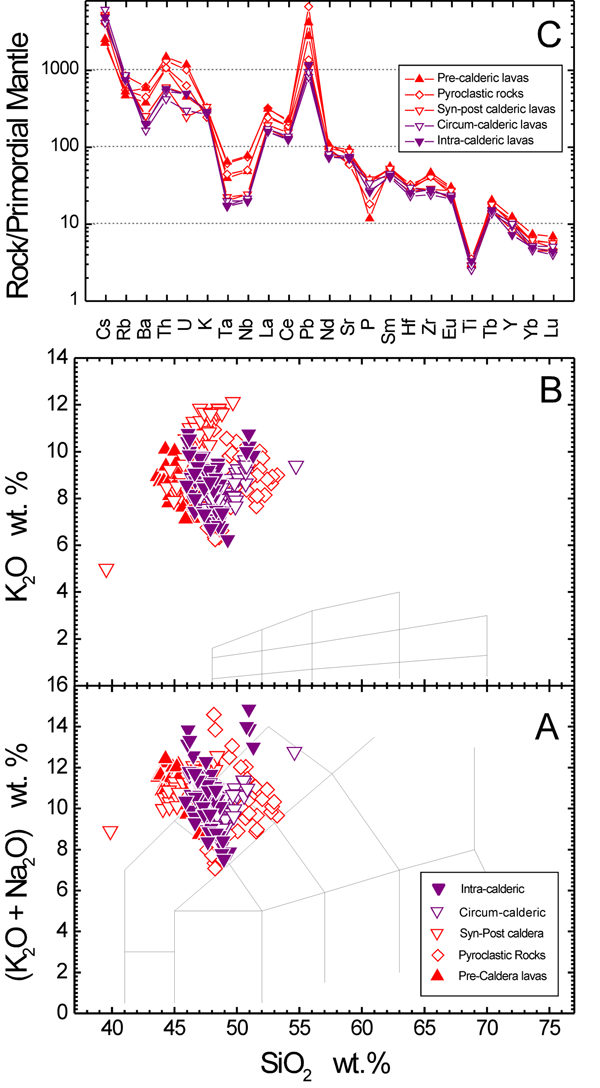
Classification and geochemical characteristics of the Colli Albani volcanic rocks. A) Total Alkali-Silica (TAS) classification diagram (Le Maitre, 2002). B) K2O wt.% vs. SiO2 wt.% classification diagram with reported the grid for orogenic volcanic rock suites (Peccerillo & Taylor, 1976). C) Incompatible trace element patterns for mafic volcanic rocks normalised to the primordial mantle values of Sun & McDonough (1989). Data from Fornaseri & Scherillo (1963); Laurenzi (1980); Peccerillo et al. (1984); Ferrara et al. (1985); Francalanci et al. (1987); Trigila et al. (1995); Freda et al. (1997); Palladino et al. (2001); Conticelli et al. (2002, 2007, 2010); Freda et al. (2006); Gaeta et al. (2006); Giordano et al. (2006); De Benedetti et al. (2008); Boari et al. (2009a).
some ignimbrites (i.e., Pozzolane Rosse, and Pozzolanelle) with phonolitic tephritic and tephritic compositions as well (Figs. 22a, 22b). This is the major compositional difference with other Latium volcanoes, where ignimbrites have invariably a phonolitic to trachyphonolitic compositions (Figs. 16a, 18a, 20a). This has been thought to represent a problem for the passive enrichment of volatites needed to trigger parossistic eruptions, and for this reason Freda et al. (1997) claimed for a supply of CO2 to early trigger eruptions from carbonate syntexis. This process has been widely discussed by several authors who found in some specific cases the evidence to support it, but in other cases questioned the abuse of this differentiation process (e.g., Freda et al., 2007; Iacono Marziano et al., 2007, 2008; Boari et al., 2009a; Gaeta et al., 2009; Conticelli et al., 2010; Peccerillo et al. 2010). These compositional differences indicate that Colli Albani magmas have lower silica activity and alumina saturation than other Roman magmas, such to stabilize melilite in many silica undersaturated volcanic rocks, to prevent crystallization of either plagioclase or K-feldspar (Conticelli et al., 2010). Among phenocrysts, Leucite is among the most abundant mineral in pre-caldera pyroclastic rocks, and in the Monte delle Faete lavas and pyroclastic rocks, whereas is confined in the groundmasses of Pre-caldera plateau melilititic lavas, and post-caldera ones. Clinopyroxene is a ubiquitous phenocryst mineral, often showing complex zoning (Aurisicchio et al., 1988; Gaeta et al., 2005). Olivine is confined in the most mafic pre- and post-caldera lavas, and like in all other ultrapotassic volcanoes it has euhedral chromite inclusions. In the groundmasses beside minerals found as phenocrysts, Ba-phlogopite, Ti-magnetite, nepheline, Ca-Fe olivines and apatite are also found (e.g., Gaeta et al., 2000; Conticelli et al., 2010; Melluso et al., 2010). Akermanitic melilite crystals are confined to the groundmasses of most silica-undersaturated foidites of the pre- and post-caldera periods. The Capo di Bove (e.g., Cecilite, Washington, 1906) and the Osa foidites are the best examples among post-caldera lavas. Melanite garnet, gehlenitic melilite and Al-spinel are typical minerals of skarn ejecta (Federico & Peccerillo, 2002), and they are found as xenocrysts in pyroclastic rocks of the Via dei Laghi hydromagmatic activity (Conticelli et al., 2010).
The primordial mantle normalized patterns (Figs. 22c) show significant differences between mafic MgO-rich magmas from pre- to post-caldera. Indeed, pre-caldera leucititic and melilititic magmas show smaller throughs at Ba, and Hf, with respect to post-caldera ones. In additions differences in LIL elements total abundances are also observed (Figs. 22c). Boari et al. (2009a) explained this characteristic as due to a different supply of magma from a slightly different mantle source. Pre-caldera magmas should have been produced by smaller degrees of partial melting, at higher pressure, of a veined metasomatised upper mantle than post-caldera parental magma.
Figure 23. Geological sketch map of the Middle Latin Valley district (Roman Magmatic Province).
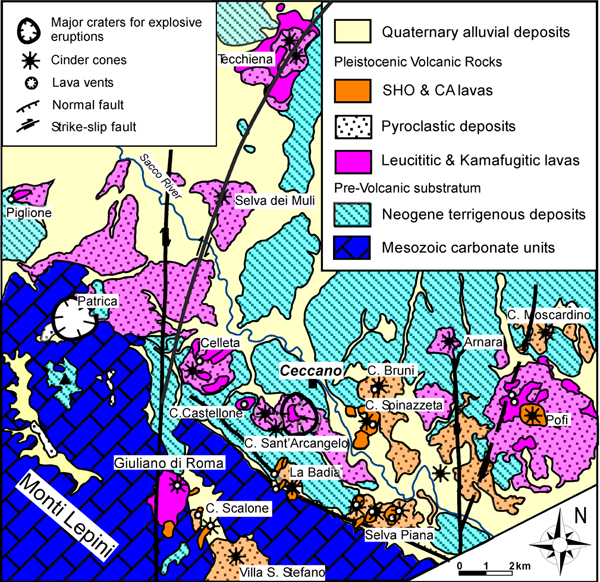
Geological sketch map of the Middle Latin District. Redrawn after Pasquaré et al. (1985), Sani et al. (2004), Boari & Conticelli (2007), Boari et al. (2009b). Main monogenetic volcanoes are represented by cinder cones, tuff rings and small plateau-like lava flows.
5. Middle Latin Valley district
The Middle Latin Valley district is located in the Southern Latium area (Fig. 11), some 100 km south of Rome, and some 60 km from Ernici Mounts, the locality to which this volcanism refers to with the name of Hernican District in the early scientific literature (Branco, 1877; Viola, 1899, 1902; Washington, 1906). Being the volcanic field developed well within the Latin Valley, in its middle sector, several authors (e.g., Angelucci et al., 1974; Dolfi, 1981; Acocella et al., 1996; Boari, 2005; Boari & Conticelli, 2007; Frezzotti et al., 2007) used that the name “Middle Latin Valley” to refer the volcanic rocks of this district; a name that better defines the outcrop locality and the geographic area than Hernican, the original name choosen for this volcanic field and kept for several years in the Earth Science literature.
The volcanism of the Middle Latin Valley district developed during the Pleistocene (Basilone & Civetta, 1975; Fornaseri, 1985a; Boari et al., 2009b). Among the Roman districts the Middle Latin Valley is the only one that lacks a large volcanic edifice; only small volumes of primitive magmas erupted from small scattered volcanic centers (Civetta et al., 1979, 1981; Pasquaré et al., 1985; Boari, 2005; Boari & Conticelli, 2007; Frezzotti et al., 2007; Boari et al., 2009b; Nikokossian & van Bergen, 2010). The total volume of erupted magma is significantly lower than that observed in all the other volcanic districts of the Roman Magmatic Province, probably due to the distinctive geological and geodynamic evolution of the Apennine chain and Adriatic foreland in this area. The volcanic field is clustered in a narrow area well within the Sacco River valley, the median sector of the Latin Valley, located between the Apennine chain and the Monti Lepini (Fig. 11). The Latin Valley is a NNW-SSE extensional basin. The volcanic field is made up of cinder cones, small lava plateau, short lava flows, and tuff rings with associated small volume of hydromagmatic pyroclastic flows (Patrica pyroclastic flows, Fig. 23). The vents are arranged along the NNW-SSE fault at the foot of Monti Lepini, which delimited the southwestern edge of the Latin Valley, and the N-S and NNE-SSW dextral strike-slip faults (Sani et al., 2004) (Fig. 23), both tectonic systems provided preferential pathways for the mafic low viscosity magmas uprise (Acocella et al., 1996).
The absence of a large central volcanic edifice and of a large area covered by pyroclastic deposits, which might be used as stratigraphic markers, makes it difficult to reconstruct a reliable stratigraphic succession. Therefore the volcanic history of the Middle Latin Valley volcanic fields has been reconstructed using geochronologic data on each single volcanic center (Basilone & Civetta, 1975; Cortesi C. reported as personal communication in Fornaseri, 1985a; Boari et al., 2009b). In addition, because the grouping has been made on the basis of geochemical and petrological informations, the time succession is strongly integrated with compositional characteristics change (Boari et al., 2009b).
Figure 24. Classification and incompatible trace element characteristics of Pleistocene volcanic rocks from Middle Latin Valley monogenetic volcanoes.
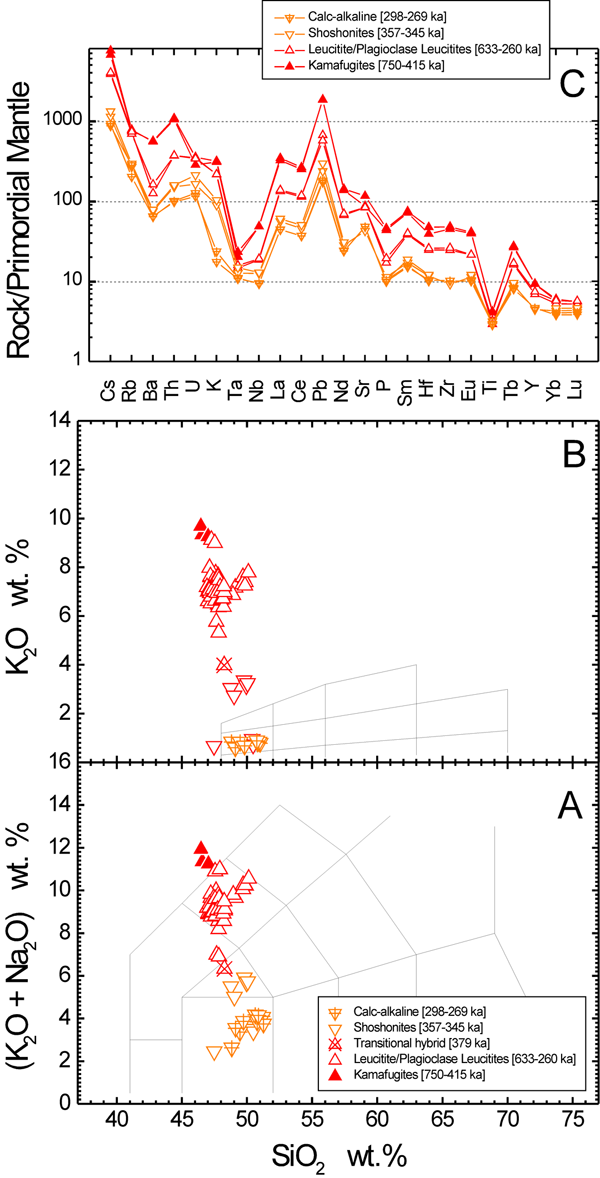
Classification and geochemical characteristics of the Middle Latin Valley volcanic rocks. A) Total Alkali-Silica (TAS) classification diagram (Le Maitre, 2002). B) K2O wt.% vs. SiO2 wt.% classification diagram with reported the grid for orogenic volcanic rock suites (Peccerillo & Taylor, 1976). C) Incompatible trace element patterns for mafic volcanic rocks normalised to the primordial mantle values of Sun & McDonough (1989). Data from Boari et al. (2009b).
Kamafugites are the early magmatic rocks to appear on surface in the volcanic field (0.7-0.6 Ma; Basilone & Civetta, 1975; Boari et al., 2009b); they are represented by leucite-bearing melilitites with a paragenesis made of melilite, olivine, clinopyroxene, phlogopite, Ti-magnetite, nepheline and apatite; secondary calcite is also found to fill vesicles and fractures. The Patrica hydromagmatic tuffs have neither juvenile fragments nor glass shards as large as to perform whole rock or EPM analyses, respectively, thus their evolved kamafugitic nature was established on the basis of mineral compositional data on loose crystal fragments (Boari & Conticelli, 2007). Patrica pyroclastic units display a much younger 40Ar-39Ar age, ~0.42 Ma (Boari et al., 2009b), which matches the K/Ar value of 0.4 Ma given by Fornaseri (1985a). All ages mentioned since now in the following discussion are 40Ar-39Ar ages, unless otherwise stated. Leucitites appears as early as kamafugites at Piglione center at about 0.61 Ma (Boari et al., 2009b). Plagioclase leucitites appears slightly later (Giuliano di Roma, and Tecchiena), between 0.41 and 0.39 Ma (Boari et al., 2009b), although a younger age of 0.25 Ma has been found for the Colle Sant’Arcangelo leucitite (Boari et al., 2009b). The leucitites and plagio-leucitites are mostly phonolitic tephrites (Fig. 24a), with no or limited differentiation (Figs. 24a, 24b). Few tephrites are also found at Selva dei Muli cinder cone and Pofi volcano. Mineralogy of leucitites and plagio-leucitites is made preferentially by leucite + clinopyroxene ± olivine ± plagioclase ± Ti-magnetite ± apatite ± phlogopite. Monogenetic volcanoes that produced leucitites and plagioclase leucitites are generally located along the N-S and NNE-SSW dextral strike-slip faults (Fig. 23). Volcanic rocks with shoshonitic affinity (Fig. 24b) appear as late as 0.36 and 0.35 Ma (Boari et al., 2009b). These volcanic rocks have trachy-basaltic compositions (Fig. 25a) with phenocrysts of olivine and clinopyroxene, set in a groundmass made of clinopyroxene, olivine, plagioclase, and Ti-magnetite; in some samples leucite crystals are also found in the groundmass (Boari & Conticelli, 2007). Basalts with calc-alkaline affinity (Boari, 2005; Boari & Conticelli, 2007; Frezzotti et al., 2007) have been vented from the same centers of soshonitic products at Colle Spinazzeta, Selva Piana, and La Badia, where basalts are stratigraphically younger than shoshonitic ones. Basaltic scoriae from the Pofi scoria cone has given an age of 0.29 Ma (Boari et al., 2009b), which disagrees with the K/Ar ages on the underlying lava flows of the plateau, 0.11 and 0.08 Ma (Fornaseri, 1985a). The leucititic lavas that gave these very young K/Ar ages show stratigraphic correlation with the lavas of the Pofi plateau (Angelucci & Negretti, 1963), dated at 0.40 Ma, (K/Ar, Basilone & Civetta, 1975). A basaltic lava from Colle Vescovo, a final center in the Selva Piana area, gave an age of 0.3 Ma (Boari et al., 2009b), coherent with the scoria sample of Pofi. These calcalkaline-like basalts (Fig. 24a) have significantly lower K2O than any other Middle Latin Valley rocks, overlapping partially with altered shoshonites where K2O has been removed by secondary processes (Fig. 24b). These altered rocks are also characterized by secondary minerals and weathering textures (Boari, 2005; Boari & Conticelli, 2007). Basalts with calc-alkaline affinity are characterized by sub-porphyritic textures with olivine and clinopyroxene phenocrysts with groundmass made of plagioclase + glass + clinopyroxene Ti-magnetite + olivine (Boari, 2005; Boari & Conticelli, 2007; Boari et al., 2009b). Monogenetic volcanoes and centres that vented lavas with shoshonitic and calc-alkalic petrologic affinities are generally located along the NNW-SSE normal fault delimiting the Monti Lepini-Monti Ausoni ridge (Fig. 23).
The lack of a large volcanic apparatus and therefore of a structured system of magma chambers beneath the Middle Latin Valley volcanic field is probably the reason for missing magmatic differentiation at shallow depth, and therefore for outcropping of mafic primitive igneous compositions (Tables 4, 5, and 6). This characteristics permit to observe a large compositional spectrum in terms of silica and K2O contents (Fig. 24b) that might be present also in the other volcanoes of the Roman province, hidden by shallow level differentiation and compositional buffering of the different magmas produced with time in the mantle source. This permits also to observe the variation of trace element contents with time and potassium contents of primitive MgO-rich magmas (Fig. 24c). Indeed as already shown for other magmatic provinces of the Mediterranean region it is possible to argue a direct and positive correlation between incompatible trace element contents and potassium enrichment in mafic primitive MgO-rich volcanic rocks (Conticelli et al., 2009a). The same holds true for the Italian ultrapotassic volcanic rocks, from Western Alps (Fig. 8) to Corsica (Fig. 10), Tuscany (Fig. 12), and Roman Province (Figs. 16, 18, 20, and 22). Indeed, the most K2O enriched mafic rocks of the Middle Latin Valley (i.e., kamafugites), have also the highest abundance in incompatible trace elements, and the normalized incompatible trace element patterns recall closely those of pre-caldera melilitites and leucitites of the Colli Albani district (Fig. 22c), with peculiar smaller negative spikes at Ba, and larger at Ta, Nb, P, and Zr with respect to younger magmas (Fig. 24c). Passing from kamafugite to leucitite/plagio-leucitite mafic rocks with time, it is observed also an increase of the negative spikes at Ba, Sr and P, which is coupled to a decrease of the troughs at Ta, Nb, and Ti (Fig. 24c). This trend is continuous with time passing also from leucitites to shoshonite and calc-alkaline mafic rocks. In addition, it is worthy to note the normalized Ta/Nb ratio that pass from a value <<1 in kamafugites to values > 1 in the youngest volcanic rocks (i.e., calc-alkaline; Fig. 24c).
Figure 25. Geological sketch map of the Roccamonfina district (Roman Magmatic Province).
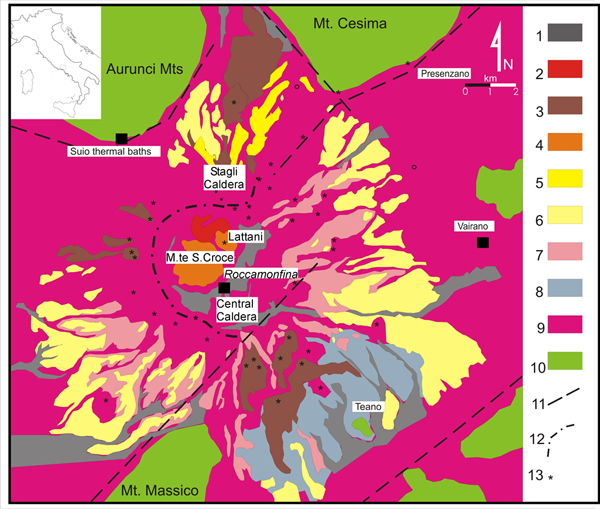
Geological sketch map of the Roccamonfina volcano (redrawn after: Giannetti, 1964, 2001; Taylor et al., 1979; Cole et al., 1992; Giordano et al., 1998a, 1998b; Conticelli et al., 2009b). Legend. 1 - Campanian Ignimbrite (erupted from Campi Flegrei); 2-5 Late phase of post-caldera activity (Vezzara synthem; 155-50 ka); 2 – HKCA final lavas; 3 – Shoshonitic mafic lava and pyroclastics from monogenetic centers; 4 – Shoshonitic domes; 5 – Yellow Trachytic Tuff; 6-8 - Early phase of post-caldera activity (Riardo synthem; 385-230 ka); 6 – White Trachytic Tuffs; 7 – Teano pyroclastic succession; 8 – Brown Leucitic Tuff; 9 – Pre-caldera activity Leucite-bearing lava and pyroclastics (HKS) (Roccamonfina synthem; 630-385 ka); 10 – Mesozoic-Cenozoic pre-orogenic carbonatic-terrigenous succession; 11 – Main extensional faults; 12 – caldera rim; 13 – scoria cones.
6. Roccamonfina district
The volcano of Roccamonfina is stratovolcano characterized by sector collapses and an apical central caldera (Fig. 25) (Cole et al., 1992; De Rita & Giordano, 1996; Giannetti, 2001; Rouchon et al., 2008). It belongs to the Roman Magmatic Province (Washington, 1906; Avanzinelli et al., 2009). It was the first volcano in which a “low potassium series” accompanying leucite-bearing ultrapotassic rocks was recognized (Appleton, 1972).
The Roccamonfina volcano is located at the intersection of a NNW-SSE extensional basin with important NE-SW and N-S tectonic lineaments, which cut the Mesozoic-Cainozoic Apennine carbonatic sequences (Accordi, 1963; Incoronato et al., 1985; Accordi & Carbone, 1988; Mattei et al., 1995; Giordano et al., 1995; Bosi & Giordano, 1997). The volcanic succession lies on transgressive marine sedimentary sequence that filled the NE-SW Garigliano Graben (Ippolito et al., 1973; Watts, 1987; Giordano et al. 1995). The Roccamonfina volcano is made up by lavas and pyroclastic rocks erupted in three main periods of activity (Ballini et al., 1989a; De Rita & Giordano 1996; Giannetti, 2001; Rouchon et al., 2008). The beginning of the volcanic activity relies on the dating of the oldest products found in the well Gallo 85-1, above the sedimentary substratum: whereas K/Ar ages of the deepest lava are slightly discordant, the 40Ar-39Ar age on a lava sample lying ~50 m above the bottom of the volcanic pile gives 0.59 Ma, (Ballini et al., 1989a). All ages older than about 0.6 Ma present in literature are likely biased at some extent (Gasparini & Adams, 1969; Cortini et al., 1973; Giannetti et al., 1979; Radicati et al., 1988). The first period of activity was dominated by leucite-bearing lava flows interbedded to minor ash fall and mud-flow deposits (San Carlo lavas), peripheral dikes, parasitic monogenetic centres, and eccentric domes on the flank of the volcano were also emplaced (Di Girolamo et al. 1991). The volcanic rocks emitted during this period have leucititic to plagioclase-leucititic affinity, ranging form basanite to phonolite (Fig. 26a), and with a mineralogy made up of olivine, clinopyroxene, leucite, plagioclase, Ba-phlogopite, apatite, Ti-magnetite and amphibole, with sanidine and rare sodalite. A first stratocone destroying event occurred at 0.44 Ma with the formation of a lateral sector collapse (Rouchon et al., 2008), but leucite-bearing volcanic activity did not interrupt and continue with the building up of a new stratocone, which covered almost completely the old volcano-tectonic depression (Cole et al., 1992a; De Rita & Giordano, 1996; Giannetti, 2001).
Figure 26. Classification and incompatible trace element characteristics of Pleistocene volcanic rocks from Roccamonfina volcano.
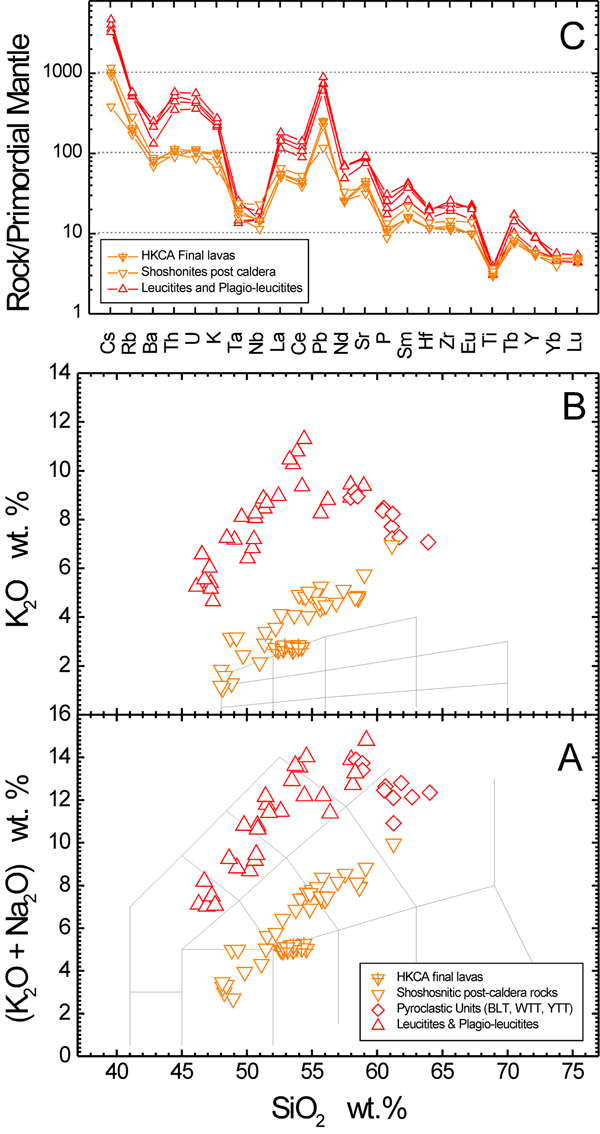
Classification and geochemical characteristics of the Roccamonfina volcanic rocks. A) Total Alkali-Silica (TAS) classification diagram (Le Maitre, 2002). B) K2O wt.% vs. SiO2 wt.% classification diagram with reported the grid for orogenic volcanic rock suites (Peccerillo & Taylor, 1976). C) Incompatible trace element patterns for mafic volcanic rocks normalised to the primordial mantle values of Sun & McDonough (1989). Data from Ghiara et al. (1973) Ghiara & Lirer (1977), Hawkesworth & Vollmer (1979), Vollmer & Hawkesworth (1981), Rogers et al. (1985); Giannetti & Luhr (1983), Luhr & Giannetti (1987), Conticelli & Peccerillo (1992); Giannetti & Ellam (1994); Conticelli et al. (2002, 2007, 2009b), Rouchon et al. (2008).
The beginning of the formation of the summit caldera marked the passage to the second period of activity (De Rita & Giordano, 1996), which was characterised by plinian activity, with the eruption of five main, caldera forming pyroclastic flow units (Giannetti & Luhr, 1983; Luhr & Giannetti, 1987; Ballini et al., 1989b; Cole et al., 1993; Giannetti, 1996; Giordano 1998a, 1998b; Giannetti & De Casa, 2000), which are namely the Brown Leucitic Tuff and the succession of the White Trachytic Tuffs (Cole et al., 1992, 1993; De Rita et al., 1998; Giannetti & De Casa, 2000). Pyroclastic units have a variable composition from phonolitic to trachytic. Recent K/Ar and 40Ar-39Ar ages of the Brown Leucitic Tuff agree at 0.35 Ma (Rouchon et al., 2008; Scaillet et al., 2008). The White Trachytic Tuff succession is stratigraphically much more complicated. Giannetti & De Casa (2000) present a range of ages comprised between 0.31 Ma for the lower unit till 0.23 Ma for the upper one. Laurenzi et al. (1990) suggested an interval between 0.32 Ma and <0.28 Ma for the whole serie, whereas Quidelleur et al. (1997) present an age of 0.33 Ma for the Lower Unit of the White Trachytic Tuff. The largest compositional range is observed among the juvenile clasts of the Brown Leucitic Tuff, which is thought to be made by a single eruption, and thus that variability might be interpreted as well as due to syn-depositional alkali-loss due to leucite and glass transformation (Giannetti & Masi, 1989; Parker, 1989). The White Trachytic Tuff falls well within the trachytic field (Fig. 26a).
The third and last period of post-caldera activity was characterized by dome to monogenetic volcanism within the caldera, along the caldera edge, and on the flanks of the primordial Roccamonfina volcano (Fig. 25). The geochronological data on the rocks of this period do not permit to define exatly the last lava flow eruption which is vented from a fracture on the western flank of Monte Santa Croce Dome and flowed down the miffle of the caldera at the foot of Monte Lattani intra-caldera dome. Indeed the Monte Santa Croce dome yielded the youngest ages found at Roccamonfina (K/Ar, 0.16 Ma, Radicati et al., 1988; 0.15 Ma, Rouchon et al., 2008). Samples from cinder, scoria cones and lava flows on the caldera rim and external to the caldera range between 0.33 Ma (Casale Robetti; 40Ar-39Ar; Laurenzi’s unpublished data) and 0.27 Ma (Colle Friello; K/Ar, Rouchon et al., 2008). Post caldera volcanic rocks are leucite-free, and mostly belonging to the shoshonitic series (Fig. 26b). The youngest leucite-free lava flows, which have been vented from a fracture on a flank of Monte Santa Croce dome, have revealed compositional variations pointing to the HK-calc-alkaline field (Fig. 26b).
Beccaluva et al. (1991) pointed out that ultrapotassic rocks outcropping below the 41st parallel display clear difference in trace element distribution with respect to those from above this parallel (Tables 5 and 6). The Roccamonfina volcano, is just few degree north from the 41st parallel and then ultrapotassic leucite-bearing rocks have incompatible trace element distribution similar to the other Latian districts. On the other hand, the post-caldera shoshonitic rocks from Roccamonfina have significantly lower LIL elements with respect to any other Roman rocks, and in particular with the other post-caldera shoshonites of the northernmost Latian districts (Fig. 16c). Shoshonitic rocks from the nearby Middle Latin Valley district (Fig. 24c) display significant differences with respect to shoshonitic rocks from Roccamonfina both in terms of total enrichments and of fractionation of HFS with respect to LIL elements (Fig. 26c). With this respect, the Roccamonfina post-caldera shoshonites display trace element distribution similarities with volcanic rocks from the Neapolitan district (Tables 6 and 7) and at a certain scale with those from Lucanian Magmatic Province (Conticelli et al., 2009b). As a corollary the leucite-bearing pre-caldera ultrapotassic rocks have no significant compositional differences with other Roman volcanic rocks from either Latium or Umbria regions. In summary, the leucite-bearing ultrapotassic volcanism of Roccamonfina is well within the chronological, mineralogical and compositional limits of the Latian districts of the Roman Magmatic Province.
7. Umbrian District
Washington (1906) fails to describe the ultrapotassic rocks found in the intra-apennine area. The monogenetic volcano of San Venanzo and the lava center of Cupaello are the major volcanic outcrops of the Umbria districts (Rodolico, 1937; Mittempergher 1965; Sartori, 1965) and they were already known in the Italian scientific literature since the end of the XIXth century (Verri, 1880; Sabatini, 1899). Several other sites in intra-apenninic areas with occurrence of volcanic rocks have been reported recently (e.g., Accordi & Angelucci, 1962; Durazzo et al., 1984; Bellotti et al., 1987; Stoppa, 1988; Michetti & Serva, 1991; Traversa et al., 1991; Brunamonte et al., 1992; Stoppa & Lavecchia, 1992; Cipollari et al., 1998; Tallini et al., 2002). In some cases these outcrops are just ancient volcanoclastic levels (e.g., Peglio and Petrasecca; Cipollari et al., 1998), in other cases are levels of airfall tuffs from either the main Roman volcanoes or Etna (Sabatini, Colli Albani, Neapolitan volcanoes, Mirco, 1990; Tallini et al., 2002; Wulf et al., 2004), and eventually they are pyrometamorphic rocks originate either by carchoal pit burning or by natural coal combustion (e.g., Melluso et al., 2003, 2005a, b; Capitanio et al., 2004; Grapes, 2006). Only for Monte Autore, La Queglia, and Polino outcrops the magmatic and the provenance nature have not been questioned. In the first case, the strong alteration allows the obtaining neither of a petrographic description nor of a bulk chemical analysis; based on clinopyroxene composition the Roman nature of the Monte authore dyke was inferred (Mirco, 1990). The La Queglia dyke is a typical alkaline lamprophyre intruded in a carbonate platform during the Eocene, much earlier than orogenic processes involved this unit in the Apennine chain (Durazzo et al., 1982; Mirco, 1990). The Polino rock has a clear alvikitic bulk composition although a hot debate within the scienfic literature questioned its primary mantle nature versus carbonate contamination with surrounding limestones (e.g., Stoppa & Lupini, 1993; Peccerillo, 1998, 2004, 2005b; D’Orazio et al., 2008).
In the lights of the strong diversity on emplacement mechanisms, nature and genesis of the rocks found within the Apennine chain we focus and limit our attention only on the classical outcrops that present a wide consensus about their ultimate magmatic nature and local venting: the San Venanzo, Cupaello, and Polino centers.
San Venanzo and Cupaello monogenetic volcanic centers (Fig. 11) are the only ones in which compositional features of volcanic rocks are unaltered and testify the original characteristics of ultrapotassic magmatism (Gallo et al., 1984; Taylor et al., 1984; Peccerillo et al., 1988; Conticelli, 1989; Stoppa & Cundari, 1995; Stoppa, 1996; Zanon, 2005). They are located within the Apennine orogenic chain. The San Venanzo lies on a horst bordering the western sector of the Tiber River Valley extensional basin, whereas the Cupaello lava vented from a NW-SE normal fault bordering the southeastern foot of the Monti Reatini at the edge of the Rieti extensional basin (Cavinato et al., 1989; Zanon, 2005).
Figure 27. Geological sketch map of the San Venanzo volcanic field (Roman Magmatic Province).
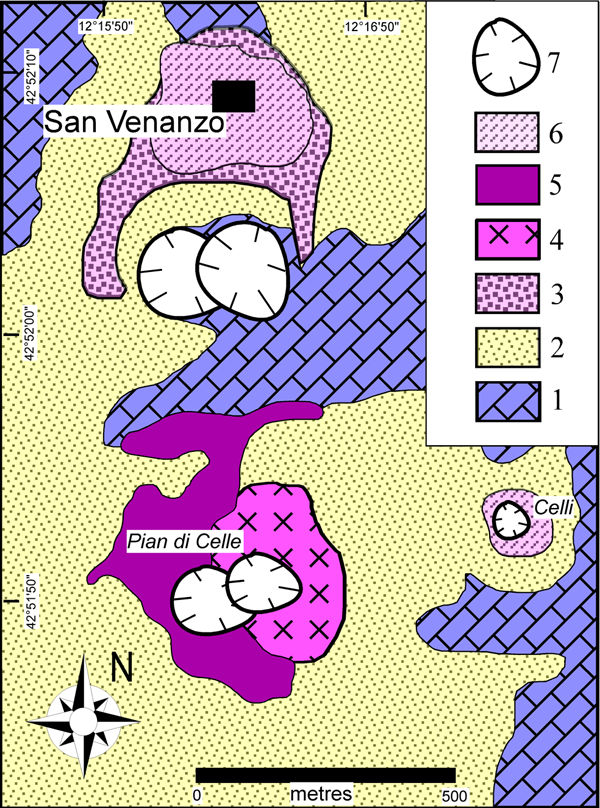
Geological sketch map of the San Venanzo volcanic field (Umbria district). Legend: 1) Miocene sandstones and marly flysh of the Umbrian sedimentary sequence; 2) Plio-Pleistocene clay and sand; 3) San Venanzo maar – breccia flow deposit; 4) Pian di Celle tuff cone – pyroclastic deposits; 5) kalisilite-bearing olivine melilitoite; 6) pyroclastic fall deposits of the San Venanzo and Celli volcanic centers; 7) volcanic centers. Redrawn after Stoppa (1996) and Zanon (2004).
The San Venanzo volcanic field includes three distinct eruptive vents: the San Venanzo maar, the Pian di Celle tuff ring, and the Celli tuff cone (Fig. 27). A K/Ar age of 0.46 Ma on leucite separated from a pegmatoid facies was provided by Laurenzi & Villa (1984). After, a more detailed chronological study gave a much younger 40Ar-39Ar age of 0.27 Ma for a sanidine from the basal tephra (Pian di Celle) (Laurenzi et al., 1994). Both phlogopite and leucite of the pegmatoid veinlets showed saddle-shaped age spectra, which account for the difference between K/Ar and Ar-Ar ages (Laurenzi et al., 1994).
Figure 28. Classification and incompatible trace element characteristics of Pleistocene volcanic rocks from Umbrian monogenetic volcanoes.
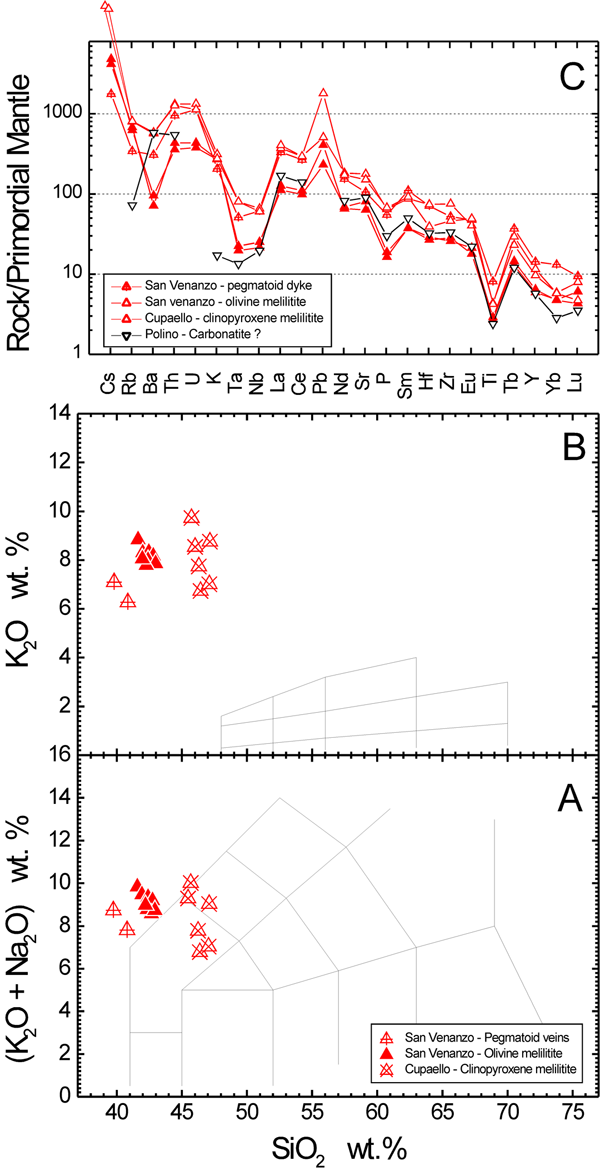
Classification and geochemical characteristics of the Umbrian kamafugitic rocks. A) Total Alkali-Silica (TAS) classification diagram (Le Maitre, 2002). B) K2O wt.% vs. SiO2 wt.% classification diagram with reported the grid for orogenic volcanic rock suites (Peccerillo & Taylor, 1976). C) Incompatible trace element patterns for mafic volcanic rocks normalised to the primordial mantle values of Sun & McDonough (1989). Data from Peccerillo et al. (1988); Conticelli (1989), Conticelli & Peccerillo (1992); Conticelli et al. (2002, 2007).
The Cupaello is a small lava tongue that has flowed down for few hundred meters above the lacustrine deposits of the Rieti valley (Rodolico, 1937; Gragnani, 1972; Cavinato et al., 1989; Stoppa & Cundari, 1995). For the Cupaello lavas, a kalsilite-enriched separate gave a saddle shaped spectrum with the only indication of a maximum age of ~ 0.64 Ma (Villa et al., 1991). The Polino outcrop is a narrow plug, some two meters in diameter, embedded in a heavy limestone sequence (ca. 6,000 meters; Michetti, 1990). Two 40Ar-39Ar ages are available for the Polino outcrop: ~ 0.4 Ma on sanidine from pyroclastic deposits and of 0.28 Ma on a massive block (Laurenzi et al., 1994).
The three outcrops of melilitites are characterized by different mineralogical assemblages of the relatively restricted whole rock compositional range (Table 4). Indeed all the samples from Umbria fall in the foiditic field (Fig. 28a) if exception is made for some Cupaello samples in which the weathering have decreased the total K2O with consequent slight increase of SiO2 (Fig. 28a, 28b). On the other hand, the samples from Polino rock do not fall within the range of the diagrams of figures 28a and 28b, but it has consistently lower silica, K2O and Na2O (Table 4) to claim for a possible carbonatitic nature (Stoppa & Lupini, 1993; Stoppa & Woolley, 1997; D’Orazio et al., 2008; Stoppa et al., 2008).
On the basis of major element chemistry and mineralogy the Umbrian rocks belongs to the kamafugitic clan (Table 1). Åkermatite-rich melilite is the most characteristic mineral of Umbrian rocks (Fig. 3), which reflects the very low silica activity, which is a consequence of the strong silica-undersaturation of the magma. Melilite-bearing rocks display the lowest silica and the highest CaO among volcanic rocks such to determine the appearance of larnite in their CIPW norm. Melilite coexists with a limited number of common minerals and appears to be equally stable with K-rich and Na-rich minerals. In igneous rocks melilite has never been found in equilibrium with either plagioclase or K-feldspar (Yoder, 1972). In the Umbrian rocks melilite is found in equilibrium with feldspatoids, both leucite and kalsilite. In the San Venanzo rocks large phenocrysts of olivine are found, whereas clinopyroxene is restricted to the groundmass together with melilite, phlogopite, kalsilite, magnetite, and nepheline, with accessory perovskite, apatite, chromite, and monticellite (Conticelli, 1989; Cundari & Ferguson, 1992). On the other hand, in the Cupaello melilitites, olivine is replaced by monticellite within the groundmass, which is found in equilibrium with melilite, clinopyroxene, phlogopite, and kalsilite. Clinopyroxene and phlogopite are present as large phenocrysts. The Polino rock is far from being an exhaustive and clear carbonatite. Indeed, phlogopite and olivine are the only phenocrysts phases, and the groundmass is made of melilite, clinopyroxene, phlogopite, kalsilite, perowskite and monticellite. Calcite is also found but its primary nature is still strongly debated (Lupini & Stoppa, 1993; Barker, 1996; Peccerillo, 1998; Wolley et al., 2005; Peccerillo, 2005b; D’Orazio et al., 2008).
Incompatible trace element concentration is among the highest total abundances observed in Roman rocks. The same distribution of other Mediterranean ultrapotassic rocks is observed for normalised patterns with high field strength element, but not Th, fractionated with respect to large ion lithophile elements, a characteristic typical of orogenic magmatic rocks (Fig. 28c). Apart this general rule, San Venanzo, Cupaello and Polino display some differences from each other. The San Venanzo olivine melilitites display a pattern strongly similar to those of ultrapotassic leucitites and plagioclase-leucitites of the Roman rocks (Figs. 16c, 18c, 20c, 22c, 24c and 26c) with comparable Ta/Nb, Nd/Sr, and Zr/Hf normalized ratios. Indeed the Cupaello clinopyroxene melilitites have the highest incompatible trace element abundances, but they do not show negative anomaly at Ba, and have reverse Ta/Nb normalized ratios (Fig. 28c). The Polino ailvikite have the lowest incompatible trace element abundances among the overall Umbrian rocks with very low K, which is a noticeable exception for carbonatite and melilite pairs, where usually the carbonatite has more than three times the incompatible trace element contents of the companion silicate rocks (e.g., Le Bas, 1987; Green et al., 1992; Kjarsgaard et al., 1995; Le Roex & Lanyon, 1998). D’Orazio et al. (2008) have shown that Polino ailvikite is strongly depleted in incompatible trace elements with respect to other similar primary mantle carbonatites.
The Neapolitan district
The Neapolitan district is the southernmost cluster of volcanoes of the Roman Magmatic Province, just south of the 41st parallel, and it is formed by four main volcanic apparata: the Ischia, Procida, Campi Flegrei and Somma-Vesuvius (Fig. 29). Most of the Neapolitan district volcanoes developed during the Upper Pleistocene but with an intense volcanic activity piercing the Pleistocene-Holocene boundary (Brocchini et al., 2001; De Vivo et al., 2001; Deino et al., 2004), and important historical eruptions recorded (e.g., Ischia, Campi Flegrei, Vesuvius; e.g., Santacroce, 1987; Rosi & Sbrana, 1987; Vezzoli, 1987). A wealth of data regarding the recent phases of activity of these volcanoes is available, but we know little about the Middle Pleistocene history of Neapolitan volcanoes, because most of them buried by Holocene volcanic activity, or just beneath the sea level. It is clear that shoshonitic volcanic products dominate over leucite-bearing ultrapotassic rocks. In addition, in most of the Neapolitan volcanoes, the silica-undersaturated ultrapotassic rocks are completely missing in the geological records, with the exception of Mt. Vesuvius, where they appears lately, after a long period characerized by either leucite-free or –poor volcanic rocks (e.g., Peccerillo, 2005a; Avanzinelli et al., 2009, and references therein).
Figure 29. Geological sketch map of the Neapolitan district (Roman Magmatic Province).
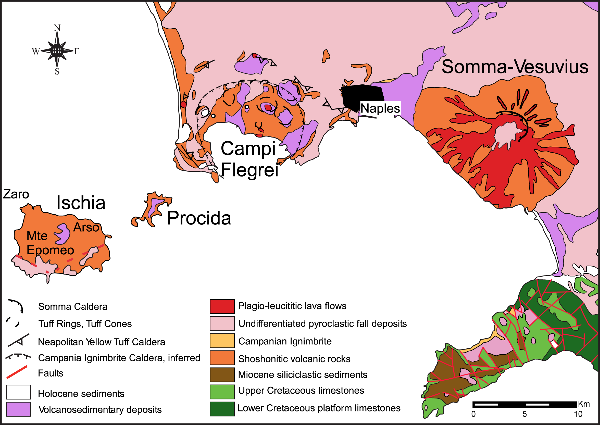
Geological sketch map of the Neapolitan volcanic district (redrawn after Bonardi et al., 1988.
The absence of Pliocene marine sediments in the drills of the Campanian Plain, indicate that the southern part of the plain was above sea level during the Middle Pleistocene (Brancaccio et al., 1991; Cinque et al., 1993), the period to which are dated back the older potassic and ultrapotassic rocks of the district (330 ka; Brocchini et al., 2001).
The volcanic rocks cover an area as large as the entire Campanian plain, and some tephra levels originated from the largest eruptions of the Campi Flegrei and Ischia can be found throughout the Mediterranean basin and over. The geological evolution of the Campanian plain and the relationships with volcanic activity have been studied in detail by several authors (Ippolito et al., 1973; Cinque et al., 1987, 1993; Albore Livadie et al., 1989; Brancaccio et al., 1991; Romano, 1992). The volcanic complexes are made up maily of pyroclastic rocks with subordinate lava flows but their structures and morphological appearance vary widely.
1. Ischia Island
The Ischia volcanic complex emerges from the sea with a height of 787 m. a.s.l. at Monte Epomeo and with an extension of about 40 km2 (Fig. 29). The island is made up prevalently by volcanic rocks, with pyroclastic ones predominant over lavas, and lava domes. The morphology and geological setting of the island is controlled by the regional geology, and local features related to dome resurgence that built the Mt. Epomeo horst (Orsi et al. 1991, 1992a; Acocella & Funiciello, 1999; Acocella et al., 2001). The subaerial volcanic activity has been divided in five main phases (Vezzoli, 1988). The eruption of the Mt. Epomeo Green Tuff marked the distinction between the old (first two periods) and the young cycle of activity.
The oldest outcropping products have not been dated and are made up by a thick sequence of pyroclastic rocks and lava flows (subordinate). Their source is unknown, and they are considered to preceed the oldest dated activity, 0.15 Ma of the lava flow of "Punta della Guardiola", outcropping in the southeastern and southwestern edges of the island (Poli et al., 1987). The former, as well as all the other ages reported for Ischia are K/Ar model ages. During the first cycle, scattered volcanic activity developed on the island with the emplacement mainly of lava domes and pyroclastic rocks, with subordinate lava flows. The cycle ended with the "Parata" lavas dated at 0.07 Ma (Poli et al., 1987). The second cycles started with the eruption of the "Monte Epomeo" Green Tuff at 0.055 Ma (Cassignol & Gillot, 1982; Gillot et al., 1982), followed by an intense pyroclastic activity that brought to the emplacement of the "Pignatiello" and "Citara" (0.033 Ma; Gillot et al., 1982) pyroclastic units, which are interbedded with deposits of several minor pyroclastic eruptions. At the end of this intense period of pyroclastic activity, whose emission centers have not been recognized, volcanism moved in the south-western sector of the island where several monogenetic centers produced an intense but scattered strombolian activity with formations of cinder cones and lava flows. This phase protracted till about 0.018 Ma (Poli et al., 1987). The last phase of activity, which started from 0.010 Ma b.p. and continued till historical times (1302 A.D.), developed in the northeastern and northwestern sectors of the island through several small monogenetic eruptive centers that erupted lava flows and pyroclastic deposits (Poli et al. 1987; Chiesa et al., 1988).
The volcanic rocks of Ischia island range in composition from shoshonites through latite to alkali trachyte and trachyphonolite (Fig. 30a), these latter rarely having a weak peralkaline affinity (Poli et al. 1987, 1989; Crisci et al., 1988; Di Girolamo et al. 1995; Avanzinelli et al. 2008; Tommasini’s unpublished data). Trachytic rocks are dominant throughout the volcanic sequence, whereas mafic rocks (shoshonites and latites) are more frequent in the late stage of activity (<0.01 Ma), in the form of scoria cones aligned along preexisting structures (Vateliero, Molara and Cava Nocelle, Fig. 29). Moreover, several eruptions produced volcanic rocks varying in composition from mafic to intermediate/evolved rocks (latite/trachyte; e.g., 1302 A.D., Arso lava flow, Fig. 29). Mingling processes are also noticed in some recent lava flows (e.g. Zaro; Di Girolamo et al., 1995). The parageneses contain clinopyroxene ± plagioclase ± olivine, with inclusions of chromiferous spinel, and rare phlogopite. Latites have less olivine, less An-rich plagioclase and more Fe-rich clinopyroxene, whereas typical trachytes have sanidine phenocrysts, to which hedenbergitic clinopyroxene, sodalite, magnetite and several accessory phases complete the mineral assemblages. Trachytes and trachyphonolites have also aenigmatite and sodic clinopyroxene within the groundmasses, thus demonstrating their peralkaline nature (Melluso’s unpublished data).
Figure 30. Classification and incompatible trace element characteristics of Pleistocenic to Holocenic rocks from Ischia volcano.
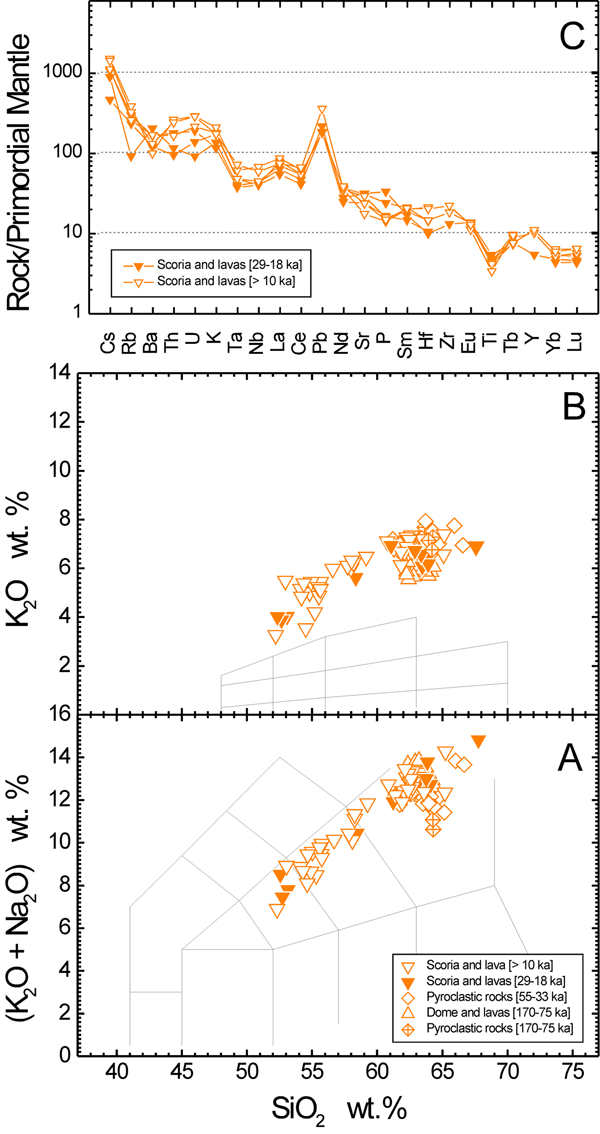
Classification and geochemical characteristics of the Ischia volcanic rocks. A) Total Alkali-Silica (TAS) classification diagram (Le Maitre, 2002). B) K2O wt.% vs. SiO2 wt.% classification diagram with reported the grid for orogenic volcanic rock suites (Peccerillo & Taylor, 1976). C) Incompatible trace element patterns for mafic volcanic rocks normalised to the primordial mantle values of Sun & McDonough (1989). Data from Poli et al. (1987, 1989), Crisci et al. (1988), Di Girolamo et al. (1995), Avanzinelli et al. (2008) Tommasini’s unpublished data).
No ultrapotassic rocks have been observed at Ischia (Fig. 30b), The Ischia mafic rocks display the lowest abundances in incompatible trace elements among the whole Roman and Italian shoshonites, but surprisingly is also the fractionation of HFE with respect to LIL elements is very weak (Fig. 30c). Troughs at Ta, Nb, and Ti as well as peaks at Th and U are negligible with respect to adjacent normalized elements (Fig. 30c). These geochemical characteristics are argued to testify the channelling of a within-plate component into the upper mantle source of Italian magmas (Avanzinelli et al., 2008).
2. Campi Flegrei and Procida Island
The Campi Flegrei volcanic field, including Procida and Vivara islands, is a volcanic area formed by small volcanic apparata and several monogenetic volcanoes, in the form of tuff rings, tuff cones and rarely cinder cones and lava domes, cropping out outside, on the borders, and within a large polygenetic caldera formed by the eruptions of the Campanian Ignimbrite and the Neapolitan Yellow Tuff (e.g., Rosi et al., 1983; Armienti et al., 1983; Di Girolamo et al., 1984; Rosi & Sbrana, 1987; Orsi et al., 1992, 1995, 1996a, 1999; Cole & Scarpati, 1993; Di Vito et al., 1999; De Vivo et al., 2001; Ort et al., 2003).
The oldest volcanic activity is represented by the pre-Campanian Ignimbrite deposits, which are found as loose remnant cropping out outside and on the borders of the Campanian Ingnimbrite caldera, and it dates back till ~0.08 Ma (Pappalardo et al., 1999) (Fig. 29). Other ignimbritic deposits are found in the Campanian Plain with 40Ar-39Ar ages of ~0.21 Ma (De Vivo et al., 2001) and ~0.29 Ma (Rolandi et al., 2003). The large volume Campanian ignimbrite (Fisher et al., 1993; Rosi et al., 1996: ca. 300 km3 of dense rock equivalent magma - D.R.E.), whose proximal products are found at Procida, Monte di Procida, Giugliano, Quarto, Cuma, bottom of Camaldoli and San Martino Hills have been produced by a large eruption, on which several age data were published (40Ar-39Ar ages: ~0.037 Ma, Deino et al., 1994; ~0.039 Ma, De Vivo et al., 2001; ~0.038 Ma, weighted average of 10 samples in Fedele et al., 2008). An intense volcanic activity occurred after the formation of the Campanian Ignimbrite caldera, with the formation of pyroclastic deposits from several volcanic centers. A second large eruption dates back at 0.015 Ma (Deino et al., 2004) with the emplacement of the Neapolitan Yellow Tuff (Cole & Scarpati, 1993; Wohletz et al., 1996), which has been estimated at 12 km3 D.R.E (Rosi & Sbrana, 1987). The most recent volcanic activity, placed within the Neapolitan Yellow Tuff caldera and on its borders, dates back to 1538 A.D. with the eruption of Monte Nuovo (e.g., de Vita et al., 1999; Isaia et al., 2004; D’Oriano et al., 2005). At present, only fumarolic and bradeyseismic activity is ongoing.
The Campi Flegrei volcanic rocks are predominantly of pyroclastic nature and vary in composition from shoshonitic basalts to trachytes and trachyphonolites (Fig. 31a), belonging to the shoshonitic series (Fig. 31b); some trachyphonolites are weakly peralkaline (e.g., Armienti et al., 1983; Villemant, 1988; Beccaluva et al., 1990; Civetta et al., 1991a; Melluso et al., 1995; Pappalardo et al., 1999, 2002; D’Antonio et al., 1999b; Paone, 2004). The most mafic compositions are present at Procida volcano as lava clasts in phreatomagmatic eruptions and scoriae from monogenetic activity (e.g., D’Antonio et al., 1999a; De Astis et al., 2004; Fedele et al., 2006). Differentiated compositions are rarely found in the mainland within the Campi Flegrei volcanic field (e.g., Concola, Cigliano, Montagna Spaccata, Nisida) and they have been achieved by open system shallow level differentiation processes (e.g., Pappalardo et al., 2002). The majority of the Campi Flegrei volcanic rocks are trachytes and trachyphonolites, including the chemically zoned Campanian Ignimbrite and Neapolitan Yellow Tuff deposits (e.g., Scarpati et al., 1993; D’Antonio et al., 1999a, Fedele et al., 2008). Evidence of interaction between mafic and evolved magmas is clearly seen in most pyroclastic eruptions (Fedele et al., 2009), and is more frequent in the latest stage of activity (<0.015 Ma), where less evolved compositions are frequently found.
Mineralogically, the primitive basalts have typical phenocryst assemblage formed mainly by Fo-rich olivine with chromiferous spinel inclusions, with Mg-rich clinopyroxene, and Ca-rich plagioclase. Latites have more Na-rich plagioclase, with clinopyroxene, phlogopite, magnetite phenocrysts and microlites, whereas trachytes have the typical assemblage K- to Na-rich sanidine, Na-plagioclase, Fe-rich clinopyroxene, Fe-rich amphibole and several accessoriy phases, with additional groundmass or microphenocryst sodalite ± nepheline. Mixed phenocryst assemblages are frequently found in both lava domes and pyroclastic rocks (e.g., Fedele et al., 2008).
Figure 31. Classification and incompatible trace element characteristics of Holocenic volcanic rocks from Campi Flegrei and Procida.
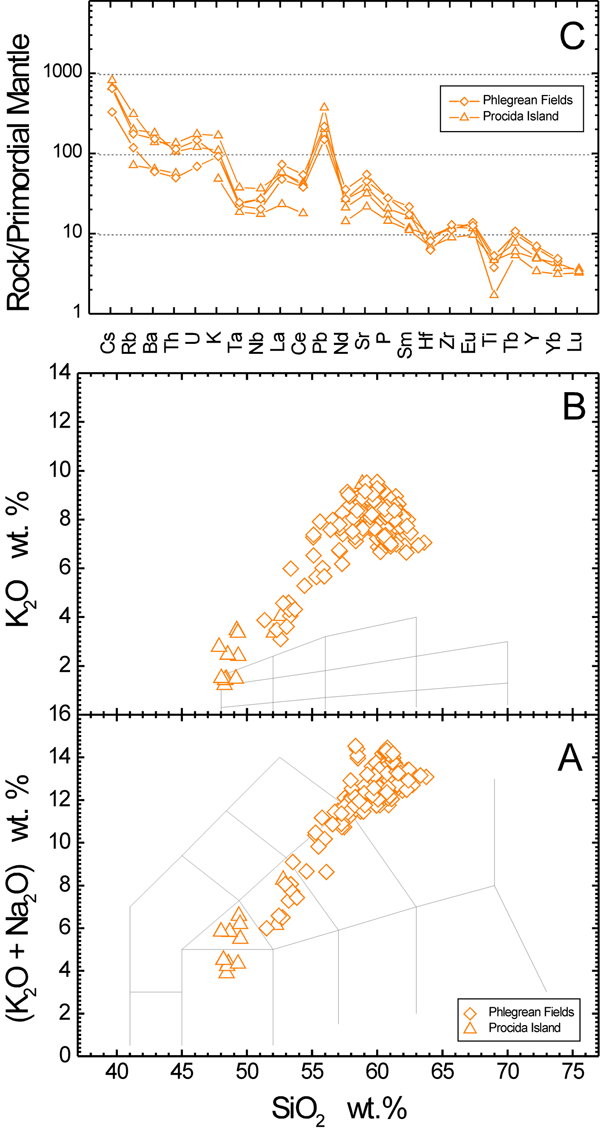
Classification and geochemical characteristics of the Campi Flegrei and Procida volcanic rocks. A) Total Alkali-Silica (TAS) classification diagram (Le Maitre, 2002). B) K2O wt.% vs. SiO2 wt.% classification diagram with reported the grid for orogenic volcanic rock suites (Peccerillo & Taylor, 1976). C) Incompatible trace element patterns for mafic volcanic rocks normalised to the primordial mantle values of Sun & McDonough (1989). Data from Pappalardo et al. (1999, 2002), D’Antonio et al. (1999b), Fedele et al. (2006, 2008), Avanzinelli et al. (2006, 2008).
Figure 32. Classification and incompatible trace element characteristics of Pleistocenic to Holocenic volcanic rocks from Somma-Vesuvius volcano.
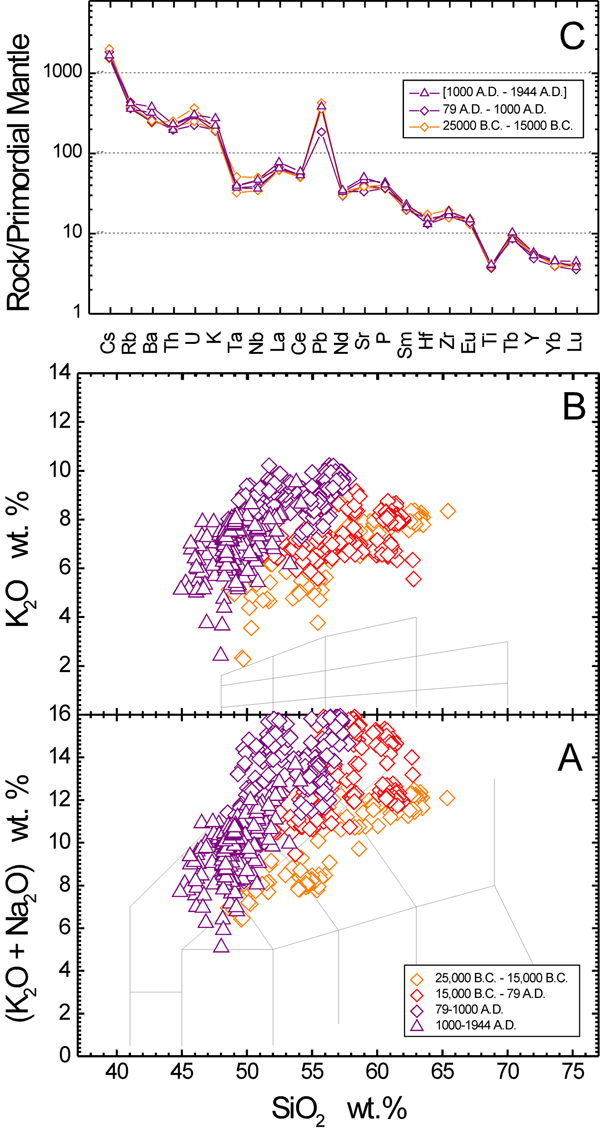
Classification and geochemical characteristics of the Somma-Vesuvius volcanic rocks. A) Total Alkali-Silica (TAS) classification diagram (Le Maitre, 2002). B) K2O wt.% vs. SiO2 wt.% classification diagram with reported the grid for orogenic volcanic rock suites (Peccerillo & Taylor, 1976). C) Incompatible trace element patterns for mafic volcanic rocks normalised to the primordial mantle values of Sun & McDonough (1989). Data from Joron et al. (1987), Ayuso et al. (1998), Di Rienzo et al. (2007), Avanzinelli et al. (2008) Melluso’s unpublished data).
Incompatible normalized trace element patterns of the mafic volcanic rocks from Campi Flegrei and Procida have mild enrichment with smooth fractionation of large ion lithophile with respect to high field strength elements (Fig. 31c). Indeed Ta and Nb troughs are extremely smoother with respect to other Roman volcanoes. The Ba trough is absent, whereas the Hf trough is more pronounced (Fig. 31c). It is important to note, as in the rest Neapolitan district the relatively low U and Th abundances with respect to the other Italian ultrapotassic and related igneous rocks, with also peculiar U/Th ratios (Avanzinelli et al., 2008).
3. Somma-Vesuvius
The Somma-Vesuvius volcanic complex is actually formed by two main volcanic edifices separated by two calderas: the Monte Somma composite volcano, disrupted by several pyroclastic eruptions which formed at least two calderas in the last 0.022 Ma (the Somma caldera s.s. and the Piano delle Ginestre calderas), and the Vesuvius s.s., a cone mostly formed by juxtaposition (and disruption) of volcanic rocks erupted from the 79 A.D. (Pompei eruption) and the 1944 A.D. last eruption. Some eccentric cones and lava domes are recorded throughout the activity (e.g., Santacroce, 1987; Rosi et al., 1993; Principe et al., 2004; Di Renzo et al., 2007; Cioni et al., 2008; Santacroce et al., 2008). The oldest volcanic activity has been found as leucitite-bearing lavas in a drill at Trecase and dated at about 0.4 Ma (Brocchini et al., 2001).
The Vesuvius rocks lack of primitive magma compositions (Table 7), due to a more or less efficient stoping in several shallow depth reservoirs (e.g., Civetta et al., 1991b, 2004; Santacroce et al., 1993; Cioni et al., 1995; Del Moro et al., 2001; Fulignati et al., 2004; Gurioli et al., 2005; Morgan et al., 2006; Scaillet et al., 2008). There is clear evidence of shift of magmatic compositions with time (Joron et al., 1987; Cioni et al., 2008, and references therein). The bulk of the Somma volcanic rocks are less undersaturated in silica, and range in composition from leucite-bearing trachybasalts to leucite latites to trachytes, whereas the more recent volcanic rocks, in the form of lava flows and juvenile products of plinian eruptions, range in composition from leucite basanite and leucite tephrite to phonolite (Fig. 32a). All the Somma-Vesuvius mafic volcanic rocks are silica undersaturated, and belong to the ultrapotassic plagioclase-leucititic volcanic series (Fig. 32b), differently from the volcanic rocks of Campi Flegrei volcanic field and Ischia volcanic complex, indicating completely independent magma reservoirs and feeding systems (e.g., Cortini & Hermes, 1981; Joron et al., 1987; Caprarelli et al., 1993; Villemant et al., 1993; Ayuso et al., 1998; Paone, 2006; Cioni et al., 2008 and references therein). Recently, Iacono Marziano et al. (2007), resurrected the old hypothesis by Rittman (1933) of shallow level carbonate assimilation to explain the transition from leucite-free shoshonitic Somma compositions to ultrapotassic leucite-bearing recent activity.
Similarly to the other Neapolitan district volcanoes, incompatible normalized trace element patterns of Somma-Vesuvius mafic rocks still have a very marked fractionation of LILE with respect to HFSE (Fig. 32c), with troughs Ta, Nb, and Ti, no Ba anomaly and inversion of Th enrichment with occurrence of a very small trough (Fig. 32c). In terms of trace element distribution the volcanic rocks of the Neapolitan district display some similarities with the volcanic rocks of the Lucanian Magmatic Province (i.e., Monte Vulture, see below), but Peccerillo (2001, 2005a) and Peccerillo & Alagna (2010, this volume) suggested a geochemical connection with Stromboli volcanic rocks (Aeolian Islands), which are, however, characterized by distinctly lower silica undersaturation.
Lucanian Magmatic Province: the Mt. Vulture and Monticchio lakes volcanoes
The Lucanian Magmatic Province is made up by the Monte Vulture and Monticchio nested volcanoes, with few small eccentric explosive monogenetic centres along the Ofanto valley and at Ripacandida. The volcanism of this province is located east of the Apennine system, not far from the Apulian foreland (Fig. 11). The Monte Vulture volcano lies on a structural high made up by Meso-Cenozoic units of the substratum with Pliocene continental sediments on their top (Schiattarella & Beneduce, 2006; Giannandrea et al., 2006). The volcanic activity at Vulture-Monticchio nested volcanoes (Fig. 33) developed entirely within the Pleistocene starting at about 0.74 Ma (Villa & Buettner, 2009). The main stratovolcano represented by the Rionero and, mainly, by the Vulture-San Michele subsynthems (Principe & Giannandrea, 2006) was built up between ~0.67 and ~0.6 Ma (Brocchini et al., 1994; Villa & Buettner, 2009). At the end of this period volcanic activity produced lava flows from parasitic vents along the north and north-eastern side of the volcano, with the emission of the Melfi haüynophire (0.56 Ma, Bonadonna et al., 1998; 0.57 Ma, Villa & Buettner, 2009) and Piano di Croce lavas, and the beginning of the sector collapse along the curved faults of the Valle dei Grigi (Guest et al., 1988; Principe & Giannandrea, 2006). The volcanic and tectonic activity of the Vulture area has been sealed by a thick paleosoil (Schiattarella et al., 2005). Volcanic activity renewed later in small centres along fractures and it is characterised by small carbonatitic lava flows and explosion craters with formation of the Lago Grande and Lago Piccolo maars (Stoppa & Principe, 1997; Principe & Giannandrea, 2006). The magmatic fraction of a surge from the Laghi di Monticchio volcanic field gives an age of about 0.13 Ma (Brocchini et al., 1994). The Toppo del Lupo lavas are likely part of this monogenetic activity (D’Orazio et al., 2007, 2008). All reported ages for this magmatic Province are 40Ar-39Ar ages.
Figure 33. Geological sketch map of the Monte Vulture volcano (Lucanian Magmatic Province).
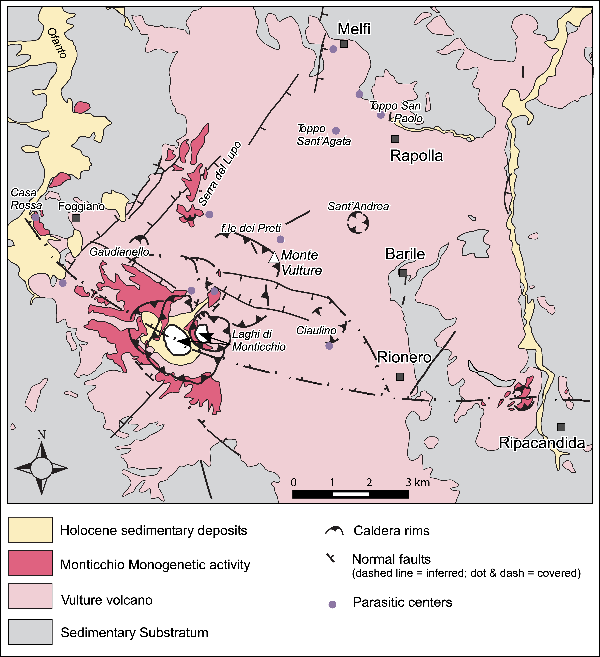
Geological sketch map of the Lucanian volcanic district redrawn after Giannandrea et al., 2006..
Figure 34. Classification and incompatible trace element characteristics of Pleistocenic rocks from Lucanian Magmatic Province.
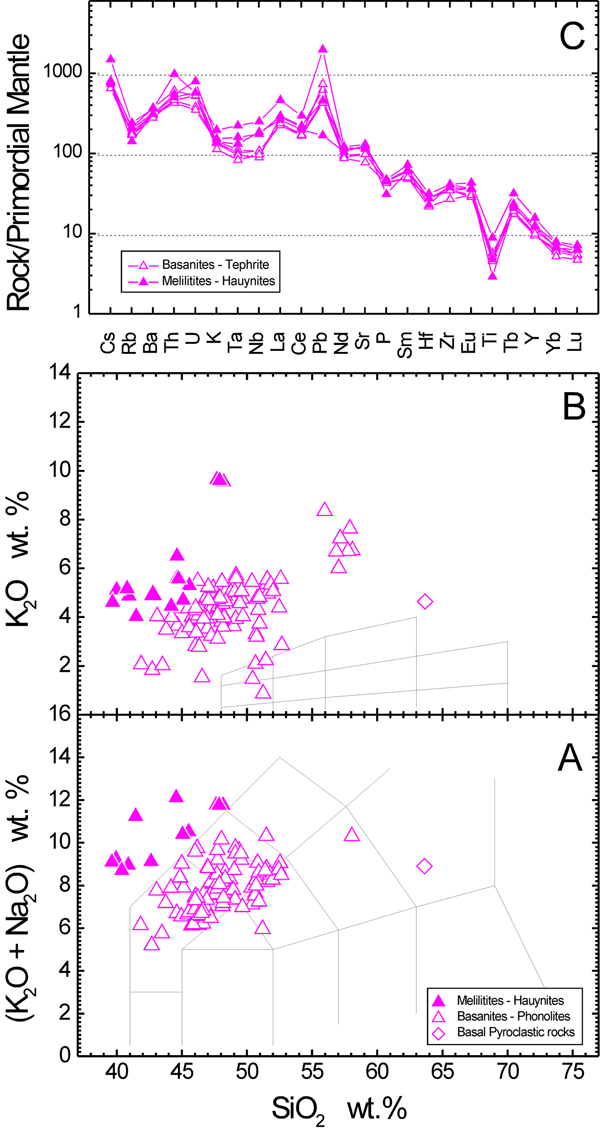
Classification and geochemical characteristics of the Lucanian volcanic rocks. A) Total Alkali-Silica (TAS) classification diagram (Le Maitre, 2002). B) K2O wt.% vs. SiO2 wt.% classification diagram with reported the grid for orogenic volcanic rock suites (Peccerillo & Taylor, 1976). C) Incompatible trace element patterns for mafic volcanic rocks normalised to the primordial mantle values of Sun & McDonough (1989). Data from Hieke Merlin (1967), De Fino et al. (1986), Melluso et al. (1996), Beccaluva et al. (2002), Downes et al. (2002), De Astis et al. (2006), D’Orazio et al. (2007, 2008), Stoppa et al. (2008), Avanzinelli et al. (2008, 2010).
The bulk of the erupted products mostly belong to a basanite-tephrite-phonolite series (Fig. 34a; Table 8), with mafic products (basanites and tephrites) dominating in both lavas and pyroclastic rocks (e.g., De Fino et al., 1986; Beccaluva et al., 2002). More silica undersaturated rocks, though minor in volume, are typical of this volcanic complex (Hieke Merlin, 1964, 1967). Melilitites, haüynites, haüyne-bearing leucitites are also found at the end of the Monte Vulture volcanic activity in the form of dikes, plugs and lava flows in the north-western flank of the volcano (Fig. 33). On the other hand, trachyphonolites are found as dykes in the neighbourings of the volcanic complex, and may be representatives of an earlier, and less silica undersaturated stage of activity (Melluso et al., 1996; Beccaluva et al., 2002). The typical phenocryst mineralogy of basanites and tephrites is composed by Fo-rich olivine (with chrome spinel inclusions), Ca-rich clinopyroxene, haüyne and leucite, to which Ca-rich plagioclase, amphibole, biotite and Fe-Ti oxides can be associated (Melluso et al., 1996; Caggianelli et al., 1990). Typical phonolites have phenocrysts of sanidine, haüyne, Fe-rich clinopyroxene, magnetite and garnet in a groundmass with additional nepheline. The melilite-bearing rocks are plagioclase-free rocks with clinopyroxene and haüyne phenocrysts; melilite is present as phenocryst or as groundmass phase, along with leucite, nepheline, Ti-rich garnet, magnetite, mica and perovskite (Melluso et al., 1996).
The Monticchio lakes volcanic activity was mainly characterized by pyroclastic deposits related to explosive and hydromagmatic activity. A small carbonatitic lava flow has been also found at Toppo del Lupo (e.g., Stoppa and Principe, 1997; D’Orazio et al., 2007, 2008; Stoppa et al., 2008). Within the hydromagmatic units round lapilli tuffs units with ejecta of intrusive carbonatites and mantle nodules are also found (e.g., Jones et al., 2000; Rosatelli et al., 2000; Downes et al., 2002). The carbonatic lava flows and pyroclastic rocks have olivine, monticellite, clinopyroxene, melilite, magnetite, amphibole and phlogopite, along with primary calcite laths (D’Orazio et al., 2007). K2O contents of the volcanic products decrease with time passing from Monte Vulture to Monticchio volcanoes. Sodic compositions are also found among lapilli tuffs of the Monticchio lake activity (Avanzinelli et al., 2008, 2010). In addition the Lucanian volcanic rocks have an increasing content of incompatible elements, coupled to a decreasing fractionation of HFSE with respect to LILE, with time passing from Monte Vulture to Monticchio products (Fig. 34c). This feature has been interpreted by De Astis et al. (2006) as an increasing contribution of asthenospheric foreland mantle in the genesis of Lucanian rocks from slab tears in the Adriatic plate subducted beneath the Italian Peninsula. A partial melting during adiabatic ascent of the upper mantle source has been evidenced for the final Monticchio lake rocks (Avanzinelli et al., 2008, 2010).
Volcanic structures of the Roman and Lucanian Magmatic Provinces
Monogenetic volcanism and dyking prevails in the Western Alps, Corsica and Tuscan Magmatic provinces, whereas volcanic edifices, ranging from flat ignimbrite shields to steep stratovolcanoes, prevail within the Roman and Lucanian magmatic provinces. This characteristic is a direct consequence of the volume of magma produced by the mantle source(s) but also of the tectonic regime acting during magma emplacement. There is a general consensus about the fact that Pleistocene volcanoes of the Roman and Lucanian Magmatic Provinces are favoured by the extensional post-orogenic tectonics. Indeed, locations and spacing on a crustal scale of the Roman volcanoes is ubiquitously related to intersection between NW-trending and NE-trending transverse extensional structures. This has been recorded in almost all volcanic districts of the Roman province: Vulsini (Barberi et al. 1984), Vico (Sollevanti 1983; Bear et al., 2009a,b), Sabatini (De Rita et al., 1983, 1996), Colli Albani (Giordano et al., 2006), Middle Latin Valley (Sani et al., 2006; Boari et al., 2009b), Roccamonfina (Giordano et al., 1995; De Rita & Giordano, 1996), Campi Flegrei (Scandone et al. 1991; Orsi et al., 1996a), and Somma-Vesuvius (Santacroce, 1987; Bianco et al., 1998) volcanoes. Furthermore, NE-trending faults are transfer structures of the regional NW-trending extensional faults, and seldom inherited from NE-trending transfer structure formed during the Apennine compression. For this reason NE-trending faults are thought to bear a more vertical dip than NW-trending faults which instead have been highlighted as low-angle normal faults in several seismic studies (Collettini et al., 2006). NE-trending fractures and faults may play therefore a determinant role to magmatic melts upwelling through the upper crust (Acocella & Funiciello, 2006).
Middle-Upper Pleistocene volcanoes of the Tyrrhenian margin, belonging to the Roman and Lucanian Magmatic provinces, show a variety of morphologies and eruption styles. Volumes involved are in the order of several hundreds of km3 for each volcanic complex and of several thousands of cubic kilometres for the whole volcanic belt (Table 9). All volcanoes, apart the Middle Latin Valley monogenetic ones, are associated with calderas, which are formed almost coevally, then suggesting a further structural and geodynamic control on volcanism. Very highly explosive volcanoes erupted several intermediate to large volume ignimbrites and are characterised by large, polyphased calderas and large ignimbritic plateaux. These complexes have been classified as caldera complexes and they are, from north to south: the Bolsena and Latera volcanoes in the Vulsinian district, the Bracciano and Sacrofano volcanoes in the Sabatian district, the Vulcano Laziale volcano in the Colli Albani district, the Campi Flegrei volcano in the Neapolitan district. On the other hand, several composite volcanoes characterized by a well formed stratocone are also present either as main edifice or as a secondary edifice during post caldera activity. At the former category belong the Vico, Roccamonfina, Ventotene, Ischia, Somma, and Vulture volcanoes, whereas to the latter one belong the Monte delle Faete, the intracaldera stratocone of Colli Albani, and the Vesuvius (Table 9).
Almost all the Roman and Lucanian volcanoes erupted intermediate volume ignimbrites, which brought to the formation of small summit collapse composite calderas (e.g., Vico, Roccamonfina, Ischia, Somma-Vesuvius and Vulture). Ischia volcanic complex hosts the only resurgent caldera (Orsi et al., 1991; Acocella & Funiciello 1999). Sector collapses associated with gravitational instability of the stratovolcanoes have also been described at Roccamonfina (De Rita & Giordano, 1996) and Vulture (Guest et al., 1988) volcanoes.
Latest stages of activity of many of the Roman and Lucanian volcanoes are associated to phreatomagmatism, which produced tuff rings and tuff cones, but maar volcanism has been also recorded (e.g., Albano lake and Monticchio lakes, Figs. 21 and 33).
The Mt. Amiata volcano, which is the northernmost Pleistocene volcanoes of this belt (Fig. 11), is somewhat intriguing. For long time it was thought to belong to the Tuscan Magmatic Province (e.g., Marinelli, 1961; Peccerillo et al., 1987; Innocenti et al., 1992; Peccerillo, 2005a), but a series of petrologic and geochemical evidence related the triggering of the Mt. Amiata volcanic activity to the arrival of leucite-bearing Roman magmas at shallow level (van Bergen et al., 1983; Conticelli et al., 2010c).
Figure 35. Nd-Sr isotopic variations for the Western Alps, Western Tyrrhenian Sea (Corsica) and Tuscan Magmatic provinces.
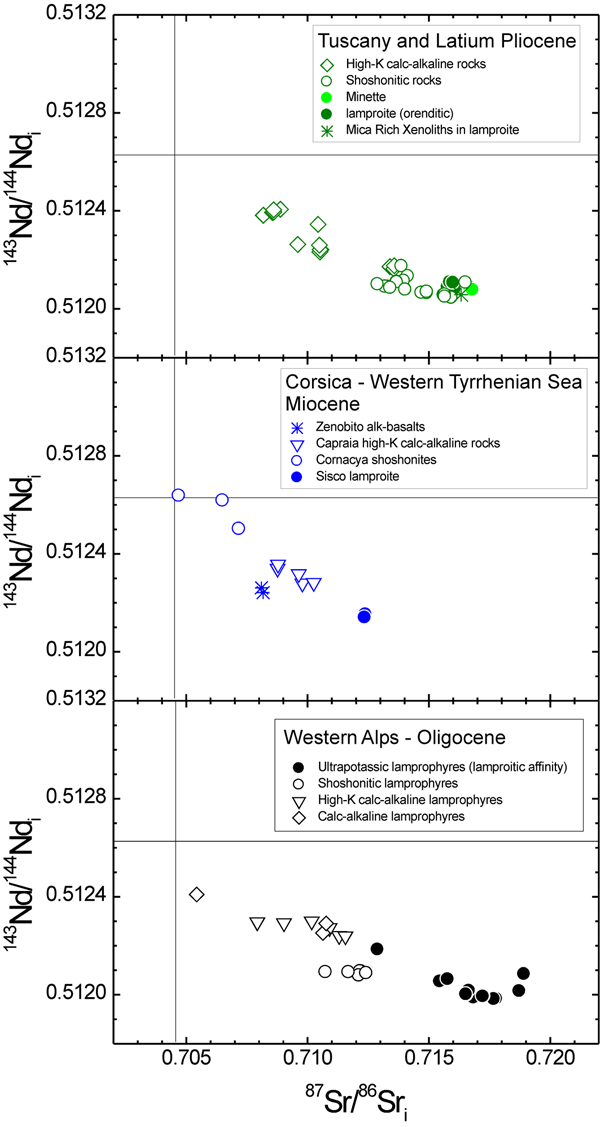
Data from Venturelli et al. (1984), Peccerillo et al. (1988), Pinarelli (1991), Conticelli et al. (1992, 2002, 2007, 2009a, 2010c, 2010d), Conticelli (1998), Cadeaux et al. (2005), Peccerillo & Martinotti (2006), Owen (2007), Prelevic et al. (2008), Avanzinelli et al. (2008).
Uprising silica-undersaturated magmas, potentially leucite-bearing, have been intercepted by long-living magma chamber filled by silica-saturated and extremely differentiated shoshonitic to calc-alkaline magmas of the Tuscan province. The interaction between the two magmas brought the extrusion of highly viscous crystal-rich Tuscan magmas together with mingled Roman magmas in which crystallization of leucite was suppressed by the interaction process with the high silica magmas (Conticelli et al., 2010c). This explains the very recent time of extrusion, which is clearly associated with the Roman Magmatic Province volcanism, but also the high aspect ratios of lava flows and the exogenous dome formation (Ferrari et al., 1996). A characteristic that has been never recorded in the volcanoes of the Roman Province, but recalling the Pliocene magmatism related with the Tuscan magmas such as the Tolfa-Manziana-Ceriti dome complexes and the Monte Cimino Volcanic complex.