The Pliocene-Pleistocene Magmatic events: Tuscan Magmatic Province
The Tuscan Region has been the site of bimodal igneous activity during the Pliocene and Pleistocene (Fig. 11). Crustal-derived magmas, formed by anatexis of lower to intermediate continental crust occurred to form granitic to granodioritic plutonic bodies (Fig. 11) and some hybrid products that have intermediate characteristics between mantle- and crustal-derived magmas have been also produced (Poli et al. 1984, 1989; Peccerillo et al., 1987; Pinarelli et al., 1989; Poli 1992, 1996; Westermann et al., 1993; Gagnevin et al., 2004, 2005a, 2005b; Poli et al., 2004). For the purpose of the present work we are not going to include here igneous rocks that are generated dominantly by magmas of continental crust derivation. They are usually treated separately from mantle derived ultrapotassic and related rocks.
Figure 11. Distribution of Plio-Pleistocene ultrapotassic igneous rocks and associated shoshonites and calc-alkaline rocks from Eastern Tyrrhenian Sea and Italian Peninsula (Tuscan, Roman and Lucanian Magmatic Provinces)
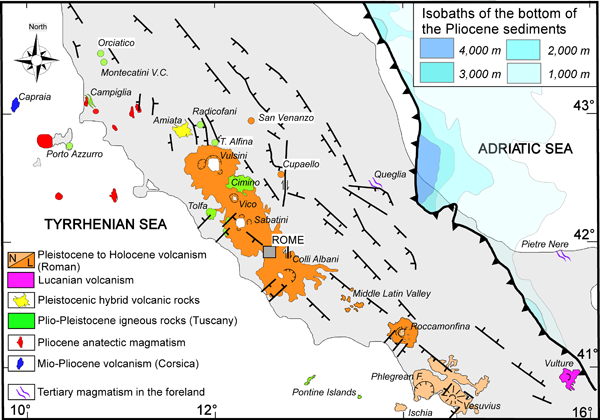
Ultrapotassic rocks are found distributed along the Tyrrhenian border of the Italian peninsula, and in some cases offshore within the eastern Tyrrhenian Sea and on the other hand in the intra-apennine setting. Tuscan, Roman and Lucanian magmatic provinces can be efficiently divided on the basis of mineralogy of the ultrapotassic rocks, timing of magmatism and location with respect to the Italian peninsula. Tuscan Magmatic rocks are Pliocenic in ages and ultrapotassic terms are always leucite-free; they are distributed in the westernmost portions of the region. Roman Magmatic rocks are Pleistocenic to holocenic in ages and ultrapotassic terms are always leucite-bearing; they are distributed in the central portions of the region. Lucanian Magmatic rocks are Pleistocenic in ages with leucite/haüyne-bearing ultrapotassic terms; they are distributed locally in the southeasternmost area of the the region. The only deviation from this rule is represented by the Monte Amiata volcano. See text for further explanation. Redrawn after Avanzinelli et al. (2009); Mattei et al. (2010).
The Pliocene mantle-derived ultrapotassic and related rocks are made up by high-potassium calc-alkaline, shoshonitic, and plagioclase- and leucite-free alkaline ultrapotassic rocks (i.e., lamproite) generated by magmas of ultimate mantle-origin (Conticelli et al., 2009a). They are found as scattered outcrops straddling the Tyrrhenian border of the Italian Peninsula (Fig. 11). Traditionally the Tuscan Magmatic Province has been considered to be confined a little beyond the administrative border of the Tuscany region (Peccerillo et al., 1987; Innocenti et al., 1992; Poli et al., 2004), but coeval leucite-free potassic to sub-alkaline magmatic rocks are found along the entire Tyrrhenian border of the peninsula (Avanzinelli et al., 2009). Therefore, in this chapter we have also included the descriptions of the coeval volcanic rocks that have been either intruded and erupted few kms north of Rome (i.e., Tolfa, Manziana, Ceriti; Fig. 11), and offshore at Ponza Island, within the Pontinian Archipelago (Fig. 11). Indeed these volcanic rocks have high-K calc-alkaline and shoshonitic affinities, although silicic terms prevail over mafic ones.
The oldest volcanic rocks of the Tuscan Magmatic Province are found at Elba Island, Tuscan Archipelago (Fig. 11) with an 40Ar-39Ar age of 5.8 Ma (Conticelli et al., 2001), followed by the emplacement in the mainland, Val d’Era area, of the hypabissal minette of Montecatini Val di Cecina, 4.2 Ma (K/Ar; Borsi et al., 1967), of the orendite of Orciatico (4.1 Ma, Capaldi G. Personal Communication in Conticelli et al., 1992) (Fig. 11), and of the olivine latitic dikes at Campiglia (Conticelli, 1989). Almost coevally are erupted the products of the Tolfa-Manziana-Ceriti dome complexes (Fig. 11). They are made up of trachytic to rhyodacitic domes, massive lava flows, and welded ignimbrites, but latitic to olivine latitic mafic enclaves are also found to testify the occurrence of mafic magmas in their genesis (Fazzini et al., 1972; Clausen & Holm, 1990; Pinarelli, 1991; Bertagnini et al., 1995). Several K/Ar dates are available for the Tolfa-Manziana-Ceriti volcanic rocks spread from 4.3 to 1.9 Ma, excluding a datum from a xenolith (Evernden & Curtis, 1965; Bigazzi et al., 1973; Lombardi et al., 1974; Villa et al., 1989). Villa et al. (1989) performed a new mineral separation on four rocks previously analysed by Lombardi et al. (1974), and obtained ages overlapping within error for Tolfa and Manziana samples, all around 3.5 Ma, either older (3) and younger (1) than previous data. Villa et al. (1989) explain the discrepancies observed among the old and new analyses with the use of mixed mineral phases by Lombardi et al. (1974), as testified by K-feldspars non-stoichiometric K contents. A fairly young age, 2.36 Ma, on a sanidine with a stoichiometric K% is reported also by Evernden & Curtis (1965). The data since now available do not allow to identify a precise time length for this volcanism.
The Pontine Islands are placed offshore of the Southern Latium coast (Fig. 11). Ponza and Ventotene Islands are placed at the same distance from the Apennine front of Tolfa-Manziana-Ceriti dome comeplexes, due to the arcuate morphology of the Northern Apennine chain. Only K/Ar data are available in literature for the Pontine Islands volcanic rocks. The recent paper by Cadeaux et al. (2005) gives an age interval from 4.2 to 3.7 Ma for the outcropping rhyolitic dykes and associated hyaloclastites of Ponza Island. Previous authors indicate older ages for the same products (5.1-4 Ma: Barberi et al., 1967; Savelli, 1983, 1988; Altaner et al., 2003). Cadeaux et al. (2005) recognise a second episode at about 3 Ma in the central-southern part of the island, and indicates an age around 1 Ma for the final subaerial activity of the Southern portion of the Island. Barberi et al. (1967) and Savelli (1988) report slightly older ages for the final activity of Ponza. Furthermore, K/Ar ages of illite-smectite of hydrotermally altered rocks constrain the alteration event at about 3.4 Ma (Altaner et al., 2003). At Palmarola Island K/Ar ages obtained from different laboratories are less discordant than in Ponza, and are comprised between 1.8-1.6 Ma (Barberi et al., 1967; Savelli, 1988) and 1.6-1.5 Ma (Cadeaux et al., 2005). The Ventotene Island is the subaerial remnant of post caldera volcanic rocks emplaced along an old calderic rim. The Ventotene stratovolcano is well below sea level. K/Ar ages on Ventotene oldest outcropping products disagree, ranging from 1.75 Ma (Barberi et al., 1967) to 0.92 Ma (Bellucci et al., 1999); the volcanic activity then continues till an undefined recent time (< 0.15 Ma for a pumice form the uppermost pyroclastic floe unit, Metrich et al., 1988). Santo Stefano Island appears as a lava dome, emplaced on a flank of Ventotene submerged sratovolcano, which is covered by pyroclastic products: K/Ar model ages are comprised between 1.2 and 0.6 Ma (Barberi et al., 1967; Fornaseri, 1985a; Metrich et al., 1988; Bellucci et al., 1999). A bit more southeast, in the Campanian Plain (Fig. 11), thick sequences of altered volcanites of calc-alkaline affinity were found in drillings (Parete 2 and Villa Literno 1 wells; Di Girolamo et al., 1976). K/Ar age of the deepest sample of Parete 2 well (2.0 ± 0.4 Ma, Barbieri et al., 1979) is devoid of analytical details. 40Ar-39Ar analyses of plagioclases separated from both wells failed to give reliable results (Brocchini, 1999). Hence, the age of this volcanism remains uncertain.
Figure 12. Classification and incompatible trace element characteristics of Tuscan Magmatic Province
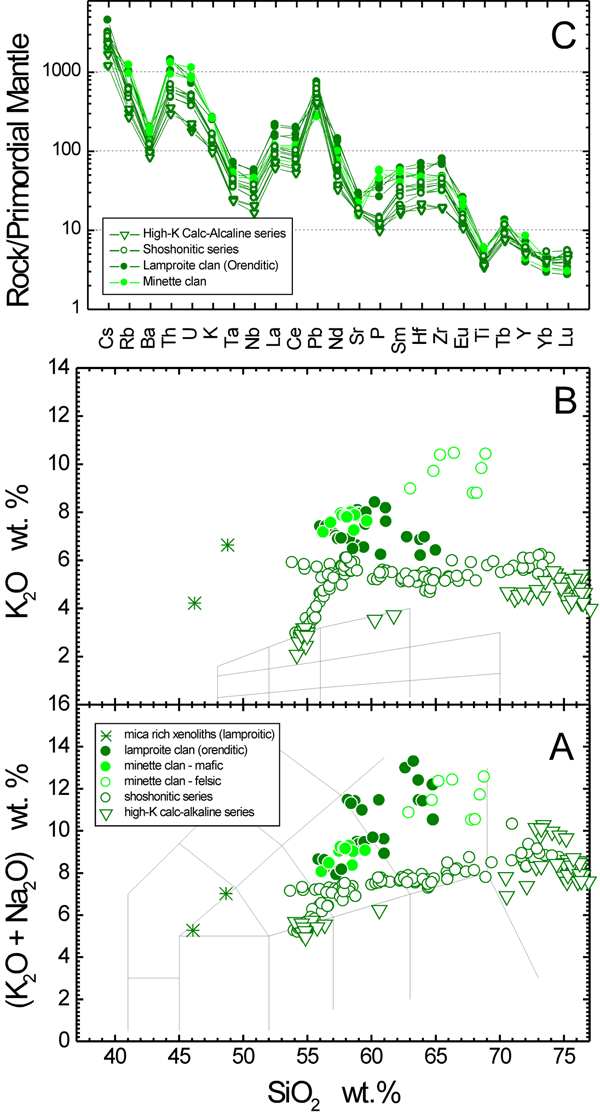
Classification and geochemical characteristics of Tuscan Plio-Pleistocene ultrapotassic (lamproites) and related rocks. A) Total Alkali-Silica (TAS) classification diagram (Le Maitre, 2002). B) K2O wt.% vs. SiO2 wt.% classification diagram with reported the grid for orogenic volcanic rock suites (Peccerillo & Taylor, 1976). C) Incompatible trace element patterns for mafic volcanic rocks normalised to the primordial mantle values of Sun & McDonough (1989). Data from Metrich et al. (1988); Peccerillo et al. (1988); Pinarelli (1991); Conticelli & Peccerillo (1992); Conticelli et al. (1992, 2002, 2007, 2009a, 2011b, 2011c); Conticelli (1998); D’Antonio et al. (1989a); Cadeaux et al. (2005); Prelevic et al. (2008).
The youngest Leucite-free ultrapotassic rocks of the Tuscan Magmatic Province are found a bit more east, erupted along the NNW-SSE extensional basin that will be occupied later, during the Pleistocene, by the Roman leucite-bearing volcanic rocks. The emplacement of the basaltic andesitic to shoshonitic lavas of Radicofani center (Fig. 11) is placed around 1.3 Ma: the 40Ar-39Ar age of one Radicofani lava flow and the K/Ar model ages of the neck agree within error (Pasquarè et al., 1983; D'Orazio et al., 1991). K/Ar model ages on the olivine latite with lamproitic affinity and latite to trachytes with shoshonitic affinity of Monte Cimino Volcanic Complex are comprised in the interval 1.43-0.97 Ma (Evernden & Curtis, 1965; Nicoletti et al., 1969; Puxeddu, 1971; Sollevanti, 1983), partially overlapping with Radicofani volcanic rocks. There are also two 40Ar-39Ar age spectra on a sanidine megacryst from the Faggeta quartz-latitic dome, without a clear age calculation (Villa, 1988: the paper was centered on Ar geochemistry). The flattish parts of the two spectra give a weighted average of about 1.1 and 1.16 Ma (new calculation from Table 1 of Villa, 1988). Only a K/Ar model age of 0.82 Ma is available for the olivine latite with lamproite affinity of Torre Alfina (Nicoletti et al., 1981a; Conticelli, 1998).
The rocks of this period range in composition from ultrapotassic (i.e., lamproite-like) to shoshonitic and high-K calc-alkalic rocks (Table 3; Fig. 12b). Shoshonitic rocks are represented by the overall spectrum of compositions, from trachybasalt to trachyte, as well as high-K calc-alkalic rocks with terms ranging from high-K basaltic andesites to rhyolites (Fig. 12a). Lamproite-like rocks are few and mostly mafic with phenocrysts of Al-poor clinopyroxene, and olivine with chromite inclusions (Fig. 3a-c), set in a groundmass made up of clinopyroxene, phlogopite, sanidine, K-richterite, apatite, picroilmentite and Ti-magnetite and accessory perrierite/chevkinite (Wagner & Velde, 1986; Conticelli et al., 1992; Cellai et al., 1993, 1994; Conticelli, 1998). The aluminium content in clinopyroxene increases from lamproite to shoshonite with contemporaneous appearance of plagioclase (Fig. 3d; Conticelli et al., 2010b). Two pyroxene high-K calc-alkaline rocks are strongly differentiated products, at Cimino, Tolfa, Manziana, and Ponza, which are associated at younger mafic lamproite-like shoshonitic rocks (Clausen & Holm, 1990; Pinarelli, 1991; Perini et al., 2003; Conte & Dolfi, 2002; Paone, 2004; Cadeaux et al., 2005; Conticelli et al., 2010d). Equilibrium clinopyroxene and orthopyroxene is the main petrographic characteristic of the most differentiated terms, which are associated to biotite, sanidine, plagioclase, apatite, zircon, ilmenite, Ti-magnetite.
The mafic Plio-Pleistocene magmatic rocks of the peri-tyrrhenian area positively correlate with K2O contents (see Fig. 8 in Conticelli et al., 2009a), showing a temporal decrease in the contents of this oxide. High-MgO volcanic rocks, irrespectively of their K2O content, display strong depletions in Ti, Ta, and Nb relative to Th and LILE (Fig. 12c). When compared to Corsican rocks, Plio-Pleistocenic volcanic rocks show higher peaks at Th, U, Pb, Zr and Hf, and deeper troughs of Ba, Ta, Nb, P, and Ti (Fig. 12c). These rocks still dispay normalized Ta/Nb and Nd/Sr lower than unity. Conticelli & Peccerillo (1992) argued that incompatible trace element concentrations and their distribution and fractionation are primary characteristics derived directly from their mantle source(s) due to sediment recycling within the upper mantle.