The Geodynamic framework
The present-day structure of the Central Mediterranean region derives from the convergence of Africa and Eurasia, which over the Tertiary occurred at about 1-2 cm/yr on average, with a total value estimated in about 400-500 km (Dewey et al., 1989). During such time, the Ionian-Adriatic lithosphere continuously subducted toward west and northwest underneath the Eurasia plate. This process led to the progressive closure of the intervening Mesozoic oceanic basins of the Tethyan domain, with the formation of a complex arcuate orogenic belt (Apennine- Maghrebide belt) and extensional back-arc basins (Ligure-Provençal and Tyrrhenian basin) (Dewey et al., 1989; Horvath & Berckheimer, 1982). The subducting slab is still recognizible beneath the Calabrian arc, where deep seismicity is detected along a narrow (~ 200 km) and steep (70°) Benioff plane dipping north-westward down to about 500 km. Continental collision is probably still active in the central-northern Apennines, where intermediate earthquakes occur down to 90 km (Selvaggi & Amato, 1992; Carminati et al., 2005).
The Africa-Eurasia convergence and the consequent subduction of the Ionian-Adriatic lithosphere, resulted in the building of the Alps-Apennine belts. The main geological evidence of such process within the Apennine chain are the deposition of thick siliciclastic deposits in the foredeep basins and the HP/LT metamorphism, in the internal part of the orogenic wedge (Jolivet et al., 1998). In the Apennines, the migration of the orogenic front is marked by the onset of siliciclastic deposits, which are progressively younger toward the Adriatic-Ionian foreland. The onset of siliciclastic deposition occurred in northern Apennines during the Late Cretaceous in the Ligurian oceanic domain, which was deformed during Late Cretaceous to Early Eocene time, and formed a double vergent accretionary wedge, now outcropping from Corsica to the Italian peninsula (Treves, 1984; Carmignani et al., 1994). Starting from the Oligocene onwards, foredeep basins migrated eastward and formed on the top of continental crust, pertaining to the passive margin of Apulia. Their incorporation into the Apenninic orogenic wedge marked the subduction of Adriatic continental lithosphere underneath Europe. Afterwards, during the Neogene, the foredeep basins further migrated toward the Apulia foreland ahead of the eastward migrating orogenic front. Foredeep basins formed on top of progressively more external units, with an eastward migration and during the Quaternary reached the configuration in the Adriatic foreland, where the foredeep deposition ceased (Cipollari & Cosentino, 1995, and references therein). During the Quaternary the southeastward rollback of the subducting Ionian plate was expressed in the southern Apennines by the progressive southeastward shifting (parallel to the longitudinal axis of the chain) of the Bradanic foredeep basin (Tropeano et al., 2002, and references therein) and of the Apenninic outer thrust front, which is presently located off-shore, in the Ionian Sea (Doglioni et al., 1999 and references therein).
In the central-western Mediterranean, differential trench retreat of the Ionian-Adriatic slab caused the formation of the Liguro-Provençal and Tyrrhenian back arc basins (Malinverno & Ryan, 1986; Lonergan & White, 1997; Faccenna et al., 2001; 2004). The Liguro-Provençal spreading took place simultaneously with the eastward drift of the Corsica-Sardinia block, which rotated counterclockwise about a pole located north of Corsica (e.g., Van der Voo, 1993; Speranza et al., 2002 and references therein). Rifting and drifting processes in the Corsica-Sardinia were related to the southeastward retreat of the subducting Ionian slab, and were accompanied by arc-related volcanism, which appeared first in Sardinia (~32My) and in Provençe and continued until ~14 My in southwestern Sardinia (i.e., Beccaluva et al., 1985; Lustrino et al., 2004).
After the end of the Corsica-Sardinia drifting, back-arc extension continued in the southern Tyrrhenian Sea. In this basin, oceanic crust formed diachronously in two sub-basins: between ~4.3 and 2.6 Ma the Vavilov basin was formed, whereas the Marsili basin developed after ~2 Ma (Marani & Trua, 2002; Nicolosi et al., 2006). Seismic, structural and stratigraphic data on the on-shore western Calabria-Peloritani terrane (Mascle et al., 1988; Sartori, 1990; Mattei et al., 2002) suggest that rifting started along the western margin of the southern Tyrrhenian Sea (Sardinian margin) during Serravallian, and progressively migrated south-eastward in the Vavilov (late Messinian-Early Pliocene) and Marsili (Late Pliocene-Early Pleistocene) basin. In the northern Tyrrhenian Sea lithospheric extension caused the formation of Neogene sedimentary basins. N-S to NW-SE oriented extensional basins developed on the previously thickened Alpine crust in the hinterland, contemporary with flexural basins in the foreland (e.g., Kastens et al., 1988) which get younger eastward as well documented by the age of the infilling sedimentary sequences. In the westernmost Tyrrhenian sea these sedimentary sequences are Lower Miocene in age and are characterized by N-S trending east-dipping normal faults (Bartole, 1995) while they are Pleistocene in age in the Umbrian region, where extensional tectonics is presently active and most of the normal faults strike NW-SE and dip westward (Jolivet et al., 1998; Collettini et al., 2006).
Present day tectonics and kinematics
Space geodesy investigations, the distribution of seismicity, geological, structural and paleomagnetic data provide a framework of the recent tectonic evolution of the Italian geodynamics, which is substantially different from that active during the Neogene and Pleistocene (until about 1 Ma), where the processes of subduction and opening of backarc basins do not appear active anymore (D'Agostino & Selvaggi, 2004; Goes et al., 2004; Mattei et al., 2007). The GPS data show that in Italy the current convergence between Eurasia and Nubia (the African plate with the exception of the region east of the East African Rift) is about 5-6 mm / year and oriented NW (Fig. 4) (Sella et al., 2002; D'Agostino & Selvaggi, 2004). The study of earthquakes and tectonics, however, has recently suggested that the manner in which this convergence is absorbed along the margin between the two plates is extremely complex, given the coexistence of compressive and extensional deformation along a wide area stretching from north Africa to Greece, and affecting also the entire Italian peninsula (McKenzie, 1972; Pondrelli et al., 1995; Goes et al., 2004). The existence of areas with low or no seismicity has suggested the possible presence of kinematically independent microplates between Eurasia and Africa. In particular, the distribution of earthquakes (Anderson & Jackson, 1987) and recent geodetic data (Ward, 1994; D'Agostino and Selvaggi, 2004; Goes et al., 2004) shows that the Adriatic region currently moves to NE respect to Eurasia, and has therefore an independent motion relative to both the Nubia and European plates (Fig. 4).
Figure 4. GPS velocities relative to Eurasia of continuous stations in Italy.
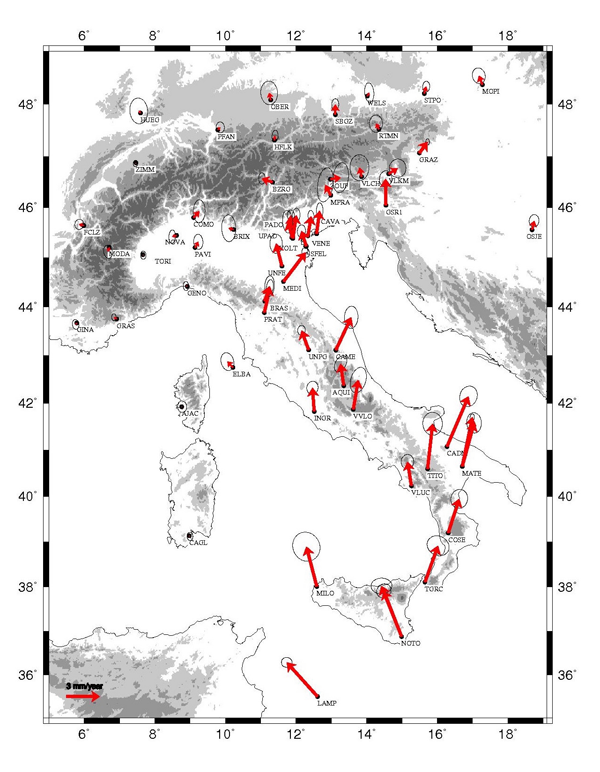
Velocities relative to Eurasia defined from continuous stations in Italy of Global Positioning Systems. Error ellipse represents the 95% confidence interval (drawn after D’Agostino & Selvaggi, 2004 and Mattei et al., 2008).
These results are consistent with several geological and paleomagnetic data that show a gradual deactivation of the compressional outer fronts of the Apennines and Sicily, the gradual decrease of the subduction processes in the Italian region and the end of the curvature processes of the Northern Apennines and the Calabrian Arc (Mattei et al., 2004; Cifelli et al., 2007; Mattei et al., 2007). These data suggest that the convergence between Africa and Eurasia is currently absorbed by the motion of the Adriatic microplate, rather than by subduction of Adriatic-Ionian lithosphere. At the same time geodetic data show that the Adriatic area is kinematically independent from the Apennine area, which shows velocity vectors oriented NNW respect to Eurasia, confirming the existence of a band of active deformation in the axis of the chain already highlighted by the study of seismicity and geological analysis (Valensise & Pantosti, 2001 and references cited). In this same area studies of deformation based on geodetic triangulation shows that the region is currently subject to an extension rate of about 3-5 mm/year NE-SW oriented (Hunstad et al., 2003), which is consistent with the focal mechanisms and the distribution of the major historical and instrumental earthquakes which occurred in the axial zone of the Apennine chain.
D’Agostino et al. (2008), on the base of GPS observations and earthquake slip vectors, suggest that the kinematics of the Adriatic region is controlled by the presence of two distinct microplates: Adria (corresponding with the northern Adriatic region) and Apulia (corresponding with the southern Adriatic, Ionian and Hyblean regions), whose relative motion controls the active deformation in the central Adriatic Sea. The opposite rotations (counterclockwise for Adria and clockwise for Apulia) of these two microplates respect to Europe are able to explain the present-day deformation pattern in the central Adriatic region and represent the way in which the relative motion between Nubia and Eurasia is presently accommodated in the Central Mediterranean region.
Structural and geological features of the extensional Tyrrhenian margin
In the westernmost northern Tyrrhenian Sea, where the Western Tyrrhenian (Corsican) Magmatic Province occur (Fig. 1), the sedimentary sequences that infill the extensional sedimentary basins are Lower Miocene in age and they are bounded by N-S trending east-dipping normal faults (Bartole, 1995), whilst in the Apennine region they are Pleistocene in age, where extensional tectonics is presently active and most of the normal faults strike NW-SE.
In the central Tyrrhenian margin, the onset of extensional tectonics can be dated at the Late Miocene because syn-rift sedimentary sequences have been recognized in extensional basins located between the Tolfa-Cerite-Manziana and Roccamonfina volcanoes (Fig. 1). In the Southern Tyrrhenian margin, south of the Roccamonfina area, extensional tectonics started more recently than in the northern and central Tyrrhenian margin, with similar eastward temporal migration. In this region the onset of the extension is marked by Late Pliocene syn-rift sedimentary marine deposits that crop out along the Tyrrhenian coast of the Campania region. Extension later progressed toward the axis of the Apennine chain, where sedimentary basins are filled by lower-middle Pleistocene continental sequences (Cinque et al., 1993), and the largest (M > 6.7) historical and instrumental seismicity ever recorded occurred.
In Northern Apennines extensional tectonics is mostly controlled by NW-SE oriented, east-dipping low-angle normal faults and associated high-angle east-dipping normal faults. Low-angle normal faults gently dipping toward the east, show evidence of present-day activity along the axial part of the Apennine chain, whereas their old equivalents are now outcropping along the western part of the margin. Here exhumed normal faults have been widely recognized in the Alpine metamorphic units in the Elba and Giglio Island, offshore Tuscany (Jolivet et al., 1998; Collettini et al., 2006). Toward the south, the existence of low-angle normal faults is less evident and active extension is mainly given by high-angle normal faults, NW-SE oriented. In Central Apennines high-angle active normal faults are mostly dipping toward the west. Such faults show a long term tectonic activity, which is responsible for the formation of large intermontane extensional basins, infilled by Late Pliocene-Quaternary continental sedimentary sequences (D’Agostino et al., 2001; Cavinato et al., 2002). High-angle NW-SE oriented, west dipping, normal faults are especially evident along the axis of the Apennine chain. Conversely, toward the west normal faults are covered by Quaternary volcanic deposits and their existence has been recognized by means of geophysical investigations and deep boreholes drilled for geothermal research (Barberi et al., 1994).
In Southern Apennine, active normal faults are at high-angle and NW-SE oriented and they are either dipping toward the east or toward the west. In particular, field observations and seismological data show that the fault responsible of the Irpinia 1981 M = 6.5 earthquake occurred along a NW-SE oriented east-dipping normal fault (Westaway & Jackson, 1984; Pantosti et al., 1993), whereas, some of the most important intermontane Quaternary extensional basins are bounded by NW-SE west dipping oriented normal faults.
All along the extensional Tyrrhenian margin an important role is exerted by transverse tectonic structures, NE-SW oriented, which either bound some of the major extensional sedimentary basins in the area, or represent the tectonic elements along which the main segments of the NW-SE oriented normal faults reverse their dip directions (Faccenna et al. 1994a; Acocella & Funiciello 2006; Barchi et al., 2007). In some cases NE-SW oriented faults also bound major NE-SW oriented extensional sedimentary basins (i.e., Baccinello-Cana, Cerite, Ardea, Garigliano), which formed parallel to the main, NE-SW oriented, stretching direction and play a major role to transfer extension to the different segments of the NW-SE oriented normal faults. The NE-SW normal faults system is particularly important along the western side of the extensional Tyrrhenian margin, whereas assume a minor importance within the axis of the Apennine chain, where extensional basins are NW-SE oriented. In particular, NE-SW tectonic lineaments represent a major factor to control the location of Quaternary volcanoes all along the western Italian peninsula. Most of the Quaternary volcanoes formed where NW-SE normal faults intersect transverse tectonic lineaments, which represent a preferential structure for magma upwelling and fluids emissions (Funiciello & Parotto 1978; Acocella & Funiciello 2006).
Extensional tectonics along the Tyrrhenian margin of the Italian peninsula has produced significant crustal thinning, high thermal flow, mantle fluids, and a characteristic distribution of seismic activity along the Italian Tyrrhenian margin. Crustal thickness has been recently defined in detail along a transect from Northern Corsica to the Adriatic Sea in the framework of the CROP 03 project (Pialli et al. 1998; Collettini et al. 2006) and using receiver functions from teleseismic data (Piana Agostinetti et al., 2002; Di Bona et al., 2008). Results converge to show the upwelling of Moho in the Tyrrhenian area, where Moho depth is about 22 km, and a progressive westward thickening of the continental crust as far as the Apennine chain, with a maximum crustal thickness of about 35-38 km observed toward the Adriatic foreland. To the west, data indicate a partial overlapping between the deep Adriatic Moho and the shallower Tuscan Moho, with a mantle slice embedded between two crustal slices.
Thermal flow is generally characterized by very high values all along the Tyrrhenian border of the central-northern peninsula, from Tuscany to Campania, but discontinuously distributed. Southern Tuscany and northern Latium have high heat flow (>100 mW/m2), with localised peaks (>600 mW/m2), which produce several geothermal fields, with the Larderello one being the most famous worldwide (Mongelli et al., 1991) (Fig. 5). Toward the south-east, thermal flow values are drastically reduced within the Middle Latin Valley, the area comprised between the Colli Albani and the Roccamonfina volcanoes, as a consequence of the presence of thick carbonatic sedimentary sequences hosting huge karstic reservoirs, which buffer heat flow to values lower than standard (30 mW/m2). It is also noteworthy that the presence of convective support to topography and strong attenuation of seismic waves consistently suggest that a positive thermal structure of the crust-mantle boundary is characteristic of a large region including the Apennine chain and its Tyrrhenian border from southern Tuscany to the Vulture area (Mele et al., 1997; D’Agostino & McKenzie, 1999).
Figure 5. Heat flow and seismicity in Central Italy.
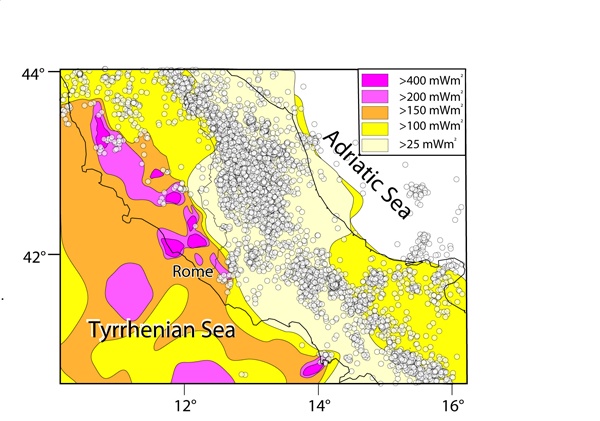
Heat flow and seismicity in Central Italy. Heat flow values are generally very high along the Central-Northern Tyrrhenian margin of the Italian peninsula (Mongelli & Zito, 1991). High values of heat flow correspond to a decrease in seismic activity, which is concentrated along very small areas corresponding to recent or active volcanoes or geothermal fields.
The extensional Tyrrhenian margin of the Apennine chain is also affected by extensive CO2 degassing, mostly derived from a mantle source and representing a significant amount of the estimated global CO2 emitted from sub aerial volcanoes (Collettini & Barchi, 2002; Chiodini et al., 2004; Miller et al., 2004). The anomalous flux of CO2 decreases toward the axis of the Apennine chain. Here fluid overpressures, documented at depth by deep boreholes, suggest that CO2-rich fluids can be trapped by stratigraphic or structural seals and released to shallow reservoirs at hydrostatic fluid pressure, triggering the main seismic events in the Apenninic chain (Miller et al., 2004). Strong historical and instrumental earthquakes have occurred along the axis of the Apennine chain, and represent the most important effect of active deformation in the Italian peninsula. In this region the extensional active stress field is NE-SW oriented, with extension rate of 2.5-3.0 mm/yr. Here, M>6.5 earthquakes nucleate in the upper crust at depth between 5 and 12 km with extensional focal mechanisms, in agreement with geodetic data (Hunstad et al., 2003) and borehole breakouts results (Montone et al., 2004). Toward the Tyrrhenian margin seismicity decreases dramatically in correspondence with the abrupt increase of positive heat flow anomalies. In this region seismic activity generally nucleates at very shallow level and is mostly concentrated along the active volcanoes and geothermal fields of the area (Fig 5).