Mid-intensity eruptions at Italian volcanoes: case histories
Mount Somma-Vesuvius
About 18 ka ago the mainly effusive activity which built Mt Somma changed to largely explosive. Since then, the Somma-Vesuvius volcanic complex (Fig. 2a) has shown many different styles of volcanic activity ranging from high-magnitude Plinian eruptions (four in the time interval between 18 ka ago and 79 AD) to low-intensity Strombolian and effusive activity.
On the whole, the magnitude of the eruptions has been roughly decreasing with time, while, after the Plinian event of Avellino (3.9 ka BP), the frequency increased.
After the Avellino eruption, the occurrence of repeated mid-intensity explosive events is recorded by thick sequences of laminated to stratified ash deposits interlayered with minor massive lapilli beds. Periods dominated by prolonged ash emission activity alternated with Violent Strombolian eruptions characterized the volcano activity between higher intensity, Plinian and sub-Plinian events (i.e. the Plinian eruptions of Avellino and Pompeii, the AD 472 and 1631 sub-Plinian eruptions). Several explosive-effusive, polyphased eruptions - classified as Violent Strombolian from the style of their paroxysmal phase - also punctuated the last 300 years of activity of Vesuvius (1638 -1944).
In the following paragraphs we present the eruptive period between the Avellino and Pompeii eruptions focusing on AP3 eruption (according to Andronico and Cioni, 2002). The Violent Strombolian eruption occurred in April 1906 is taken as representative of this class of events.
Figure 2b-g. Summarizing table for the AP3 eruption at Vesuvius
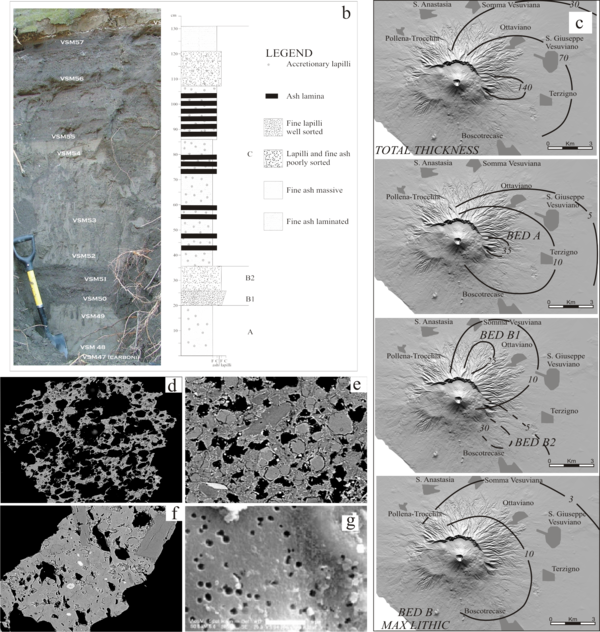
b) stratigraphic succession and schematic section at S. Pietro Quarry outcrop, located at about 3 Km eastward from the eruption vent; c) isopach maps (cm) of the deposits (modified from Andronico and Cioni, 2002), showing the total thickness (top), the Bed B1 and B2 (middle), and maximum lithic from Bed B areal distributions (bottom), used for the Mass Discharge Rate calculations; d, e, f) SEM Backscattered (BSE) images of different types of clasts: d) light, glassy, vesicular pumice; e) dense, highly crystallized, poorly vesicular scoria; f) poorly vesicular, crystal-poor, glassy fragments; d) pitting on the external surface of a clast.
1. Eruptive period between Avellino and Pompeii Plinian eruptions
Between the deposition of the Avellino Pumice (3500 year BP) and Pompeii Pumice (79 AD) Plinian eruptions, a complex sequences of fallout, massive to thinly stratified, scoria-bearing lapilli layers and ash events were deposited, reaching a maximum thickness in proximal area of about 800 cm. Thin paleosols, humified layers, and primary erosive unconformities generally separate the deposits of different eruptions. At least 6 main events were recognized (identified using the abbreviation "AP") followed by a progressive number (Andronico and Cioni, 2002). The first two events (AP1-AP2) were vent eruptions located 3 km west of the present crater of Vesuvius, in correspondence of the Piano delle Ginestre area as in the earlier Avellino eruption. Their stratigraphic features and clast characteristics suggest they were generated by a sub-Plinian to phreato-Plinian activity. Following these two eruptions, the stratigraphic succession records the deposition of four main eruptions (AP3–AP6 members) and at least two minor events (AP3 α and AP4 α members) totalling more than 4 m in thickness on the south-eastern slopes of the volcano. The succession consists of a monotonous sequence of fallout deposits largely represented by accretionary lapilli, massive to laminated ash, alternated with only minor lapilli beds. The AP3-AP6 succession was interpreted as mixed, violent Strombolian to Vulcanian events (Andronico and Cioni, 2002). The dispersal of these deposits indicates a shift in the position of the eruptive vent from Piano delle Ginestre to the area of the present crater. Along the eruptive sequence, the mean composition of the ejected material changes from tephritic phonolites to phonolites (AP1-AP2) to tephri-phonolite (AP3), with a progressive and continuous shift toward less evolved terms with time. This compositional variation shows a strong correlation with vent position and eruption style, reflecting in a lowering of melt viscosity, which can favour the lower intensity observed for the AP3–AP6 eruptions (Andronico and Cioni, 2002).
AP3 eruption
AP3 deposit consists of a fallout sequence mainly formed by ash beds and minor scoria layers. It has been subdivided into three main beds, based on colours changes and grain size of the products (Fig. 2b). The base is represented by an accretionary lapilli-rich, normally graded, vesiculated ash layer (Bed A). It is formed by massive, light-colored, fine ash and minor coarse ash laminations. In a few outcrops, this bed directly rests on a discontinuous, humified layer containing small, charred, vegetal remains. The transition toward the overlying Bed B is marked by a change in colour, corresponding to an abrupt change in the microscopic texture of juvenile shards. Bed B is an ubiquitous, coarse-grained fall deposit. Two main units are recognized. Unit B1 consists of a massive layer formed by light green-coloured, sometimes banded, scoria. Unit B2 is formed by an alternation of thin layers of dark, poorly vesicular, ash-coated lapilli and cohesive fine ash. At the top, a complex sequence of fine-grained, faintly laminated, accretionary lapilli-bearing, ash layers is present (Bed C). It represents the thickest bed (up to 1 m) of the AP3 member, possibly deposited by repeated eruptive pulses. It is very difficult to correlate the different layers forming Bed C because of their variable main direction of dispersal. In distal outcrops, up to about 14 Km from the vent, the deposit consists of three layers, correlated with A, B, C beds of the proximal section. At the base, bed A consists of 5 cm thick, massive layer, formed by light ash. Bed B is a 4 cm thick, well sorted, lapilli-bearing layer. At top, bed C consists of a 15 cm thick, accretionary lapilli-bearing layer, at the top of which are 6 cm of lapilli deposit. The total volume estimation of the whole AP3 deposit resulted 1.5 x 108 m3. Andronico and Cioni (2002) estimated the peak magma discharge rates (MDR) from isopleth maps of the maximum lithics in AP3 Bed (Fig. 2c). They found that the measured clast dimensions were close to the lower range of applicability for the method. The inferred peak MDR was of 3×106 kg s-1 (Wilson and Walker, 1987), corresponding to column heights of about 10 km. MDR values are one order of magnitude lower than those of most known Plinian and sub-Plinian events of Vesuvius (Carey and Sigurdsson, 1987; Rolandi et al. 1993a, 1993b; Rosi et al. 1993). Using the method proposed by Carey and Sparks (1986), Andronico and Cioni (2002) also calculated values of maximum column height of around 12 km, and a tropopause wind speed of ~15 m s-1. These values correspond to MDR about two times larger than those calculated with the previous method. No MDR estimation was possible using these classical methods on the ash-rich beds always present in the different members.
The products of the AP3 eruption are mostly made of juvenile materials (pumice, scoria, accretionary lapilli and loose crystals) and in minor part, of lithic fragments which consist of altered leucite and pyroxene-bearing lavas and very rare skarn material. Juvenile fragments are largely heterogeneous showing a variability of the physical, textural, and, in some cases, compositional features. As a general rule ash fragments vary between two end members: a) light, glassy, vesicular pumice (Fig. 2d) and b) dense, highly crystallized, poorly vesicular scoria (Fig. 2e). All the intermediate terms between these types exist. In particular, poorly vesicular, crystal-poor, glassy fragments are well represented (Fig. 2f). The large amount of coalescing vesicles relative to the total vesicularity of fragments suggests an important role of permeability in magma degassing. In some cases the pumice-like portion of the magma includes or envelops the dense, dark portion. Juvenile material is characterized by a porfiritic index of 1-5%, with a mineralogical assemblage represented by rare, mm-sized phenocrysts of leucite, pyroxene and biotite set in a groundmass of leucite, plagioclase and pyroxene with variable glass.
The stratigraphic observation and clasts characterization allow identification in AP3 eruption two contrasting styles of activity, each repeated during the eruption at least two times: 1) emplacement of a ash-rich layers (this activity dominated both the first phase of the eruption and the central part of the eruption); and, 2) deposition of well sorted lapilli-rich layer, present in the lower part and at the top of the sequence.
1) Ash-rich layers - Deposits of this phase of the eruption consist of massive, centimeter thick, fine ash-rich layers and are characterized by features such as accretionary lapilli and vesiculated tuff, typical of deposition from a “wet” system. Juvenile clasts have blocky and platy external shapes with diffuse superficial pitting (Fig.2g) and adhering of small particles, together with hydration and contraction cracks. Although different types of particles coexist within the same stratigraphic layer, the majority of the fragments (67% of Bed A, and 46% of Bed C) show a low sphericity external shape and smooth, not ragged external surface, while very angular fragments with irregular outlines are scarcely represented (5% in Bed A, and 20 % in Bed C). In addition, groundmass characterization of the ash evidenced the presence of poorly vesicular, poorly crystalline glassy fragments, together with dense, microlite-rich fragments in samples. These features have been associated with phreatomagmatic explosions in which the explosive vaporization of external water results in the fragmentation and quenching of the magma (Wholetz, 1986; Büttner et al. 1999, Morrissey et al. 2000; Andronico and Cioni, 2002; Taddeucci et al. 2002). The ubiquitous and large occurrence of moderately vesicular, glassy fragments, on the other hand, suggests that external water only acted to increase the intensity of a fragmentation process which was primarily driven by the gas bubbles explosions. According to this hypothesis, interaction of external water with the magma caused an increase of mixture pressure favoring the fragmentation of the poorly vesicular portions of the magma.
2) Lapilli-rich layers - Bed B, representative of violent Strombolian episodes consists of abundant scoria with large spherical to ovoid vesicles, separated by thick septa. The external surface of the clasts shows minor pitting and very minor occurrence of small adhering particles. The study of particle outlines revealed an increase of very angular fragments with bubble-wall-controlled contours (33 % in Bed B and 26 % in Bed C top). Groundmass analyses highlighted that moderately vesicular, glassy fragments represent the most abundant (more than 50%) type of ash emitted during these phases of activity, associated with dense, crystal-rich and moderately vesicular, crystal-rich fragments. These features are indicative of efficient magma degassing at fragmentation in fully magmatic Strombolian activity (Heiken and Wohletz, 1985). Moderately vesicular clasts with an almost completely crystalline groundmass are already present in these beds suggesting an extreme pressurization caused by microlite growth (Sparks, 1997; Gardner and et al. 1998). The last of these possibly represents the shattering of magma lining the external portions of the conduit, crystallized for the dual effects of degassing and cooling.
From crystal size distribution analysis of plagioclase microlite growth in the groundmass of the juvenile clasts it is possible to estimate the duration of the AP3 eruption (Cashman, 1988; Marsh 1988; 1998; Higgins 2000). Assuming that plagioclase grew at depth in the magma chamber/conduit and had a growth rate of 10−7-10−8 mm s−1 (Fenn, 1977; Hammer et al. 1999; Cashman and Blundy, 2000), the residence time for magma (i.e. the duration of the eruption) result of 10-20 days (D’Oriano, PhD thesis, 2008).
2. Violent Strombolian eruptions
This kind of eruption is thought to be the most likely type in the next, short-term reactivation of the volcano (Marzocchi et al. 2004; Neri et al. 2008).
Eruptive dynamics, products and impact of violent Strombolian eruptions occurred in the last three centuries; in particular, the two last events (1906 and 1944) are well described in numerous contemporary accounts and scientific papers.
The paroxysmal phase of these eruptions (the violent strombolian phase) has a purely magmatic character and consists in up to some kilometers-high, quasi-steady lava fountains. Several episodes of lava fountaining, each lasting from tens of minutes to hours, can occur, and the total duration of this phase ranges from hours to days. Coarse grained scoria beds (up to 1 meter on the volcano slopes) are deposited. Heavy ballistic showers can reach towns at the foot of the volcano. This phase is often preceded by overflow from the crater or lava emission(s) from lateral vents. Intense lava effusions from lateral vents can also occur during the violent Strombolian phase and affect towns located on the volcano slopes.
The final part of the eruption lasts from hours to days. Quasi-steady eruptive columns several kilometers high can develop. Repeated explosions generate dense ash-plumes dispersed by low level winds. This activity has a phreatomagmatic character, possibly related to the involvement of fluids from shallow hydrothermal systems (Bertagnini et al. 1991; Cioni et al. 1992; Mastrolorenzo et al. 1993; Fulignati et al. 1996; Andronico et al. 1996). The waning stages are dominated by the emission of wet fine-ash.
Magmas feeding these eruptions range from tephrites to phonolitic tephrites. Volatile content in melt inclusions hosted in high-Mg olivines is high, exceeding 5 wt%. and points to the involvement of a deep-seated, volatile-rich magma, possibly residing in an mush column located at a depth of ≥ 8 km (Marianelli et al. 2005).
The 1906 eruption
The 1906 eruption lasted 18 days and was observed by many contemporary scientists, but the most exhaustive descriptions of the eruptive phenomena, nature, volume and impact of emitted products are due to Mercalli (1906), Perret (1924), Johnston Lavis (1909) and Sabatini (1906).
According to Perret (1924), the beginning of the eruption can be set at 5:30 am on 4 April with the opening of a radial fracture on the southern slope of the Vesuvius cone and the activation of an effusive vent at an elevation of about 1200 m. In the following three days, new vents opened both on the same radial fracture and farther east at elevations of about 600 and 800 m. The lava emission was limited to a narrow sector 30° wide (Sabatini, 1906). From the morning of 4 April the explosive activity at the crater progressively increased and ash-laden convective clouds rose up to 2-3 km. In the morning of 7 April a moderate decrease of the explosions was observed. After 6 April a maximum lowering of the sea level of 50 cm indicated the swelling of the volcano (Mercalli, 1906).
In the early afternoon of 7 April, the magma level quickly rose and a strong increase of the explosive activity began. In the evening, 1-2 km high incandescent jets closely followed one each other as to form a true persistent fire fountain (Mercalli, 1906). A strong resumption of the effusive activity occurred shortly after and lava flows reached the villages of Boscotrecase and Torre Annunziata. Around 11 pm the intensity of the explosions further increased up to a maximum between 1-3:30 am (Mercalli, 1906). In this time interval a heavy fallout of lapilli, bombs and blocks affected Ottaviano and other villages at the NE foot of the volcano. According to Mercalli, the explosive climax ended at 3:30 am when the upper portion of the Vesuvius opened “like the falling of the petals of a flower” (Perret, 1924). The unfolding of the cone marked a change in the eruptive style and the beginning of the “intermediate gas phase” of Perret and the “vulcanian phase” of Mercalli. The descriptions of the two volcanologists of the activity are somewhat different, possibly because they observed the eruptive phenomena from two different points of view (Mercalli from Naples and Perret from the Vesuvius Observatory, at 1.5 km from the crater). Perret describes a quasi-steady column, as a huge persistent geyser which, with recurring “crescendo-diminuendo” effects, attained a height of 13 km in the early afternoon of 8 April (Fig. 3a). According to Mercalli, during all the day of the 8th, the volcano was enveloped in an impenetrable cloud of reddish ash while 4-5 km high, cauliflower dark grey clouds rose at short intervals. Towards the evening the violence of the explosions began to decrease; however, not until 10 pm or later when the lapilli shower stopped at Ottaviano (Johnston-Lavis, 1909).
In the night between 8 and 9 April the explosive activity progressively weakened and the ejected material became finer and finer (Johnston-Lavis, 1909). During the whole day ash-laden clouds were drifted by wind towards SW. As a result, the whole area was plunged into a total darkness, while a reddish-grey pisolitic ash was falling (Mercalli, 1906).
In the following days the ash fallout continued with a general intensity decrease. By 13 April the ash had a reddish color then turned light grey, giving to the Vesuvius cone a peculiar snow-covered effect (Johnston-Lavis, 1909). On 21 April the eruption was effectively over, but in the following weeks several episodes of lahar generation extensively damaged the N and W sectors.
Figure 3. Summarizing table for the 1906 eruption at Vesuvius
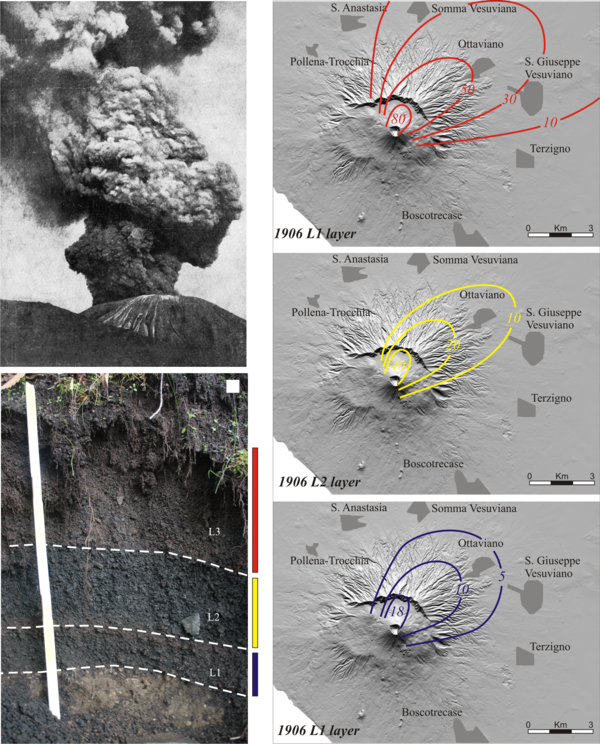
a) 8 April eruption plume (photo of Perret, 1924); b) stratigraphic succession of the proximal deposit with indications of the different layers; c) isopach maps (cm) of the different layers (modified form Arrighi et al. 2001).
The fallout of coarse material chiefly affected the NE sector (Fig. 3b). In the village of Ottaviano, located at 6 km from the crater along the dispersal axis, the deposit attained an average thickness of 80 cm and was formed by two main beds: the lower layer (∼20 cm) was mainly constituted by black vesicular scoria lapilli further subdivided in two layers by the presence of a reddish ash coating on the scoria clast (L1 and L2 in Fig.3c) while the upper one had a more abundant lithic component and a characteristic reddish-ash coating of the clasts (the cocoa-coloured lapilli of Johnston-Lavis, L3 in Fig. 3c).
The ash dispersal, strongly controlled by the dominant winds, was rather variable. Up to 20 cm of red ash was measured in Torre del Greco (6 km SW from the cone) and 15-20 cm near Ottaviano (Mercalli, 1906). Light ash fallout was reported in a ENE direction (1 cm in Apulia about 200 km distant) up to Montenegro, at about 400 km.
In the villages of Ottaviano and nearby San Giuseppe Vesuviano 216 people were killed and 112 injured by roof collapses. A further 11 people died and 30 were injured in the collapse of a shed in a market in Naples and around 34,000 people became refugees (Chester and Duncan, 2007).
A minimum volume, varying from 5.7 x 106 m3 (Sabatini, 1906) to 20 x 106 m3 (Mercalli, 1906) was estimated for the lava flows. According to Sabatini (1906) the coarse fallout deposit had a volume of about 12.1 x 107 m3 and the distal ash a minimum volume of 2.8 x 107 m3. Mass discharge rates of 0.34-5.38 x 106 kg/s was calculated by Arrighi et al. (2001) on the basis of durations deduced by historical chronicles and field data.
Phlegrean Fields
The Phlegrean Fields is a 13 kilometres-wide caldera situated to the west of Naples (Italy) that resulted from two big collapses which occurred 35,000 and 12,000 years ago. Since the beginning of their activity (not precisely known, but around 60,000 years ago) they were characterized essentially by explosive eruptions and subordinately effusive activities (Orsi et al. 1996; Pappalardo et al. 1999), the products of which range in composition from latite to phonotrachyte. After the second caldera collapse the following eruptions were generated by vents located either inside the caldera or along its structural boundary. About 72 eruptions occurred in three epochs of volcanic activity between 12 and 9.5 ka, between 8.6 and 8.2 ka and between 4.8 and 3.8 ka (Di Vito et al. 1999). The volcanic activity was mostly explosive with phreatomagmatic phases generating tuff rings and tuff cones and subordinately effusive. The most recent eruption formed the Monte Nuovo tuff cone (Fig. 4a) in 1538 AD after a quiescence of about 3,000 years.
Figure 4b-f. Summarizing table for the AP3 eruption at Vesuvius
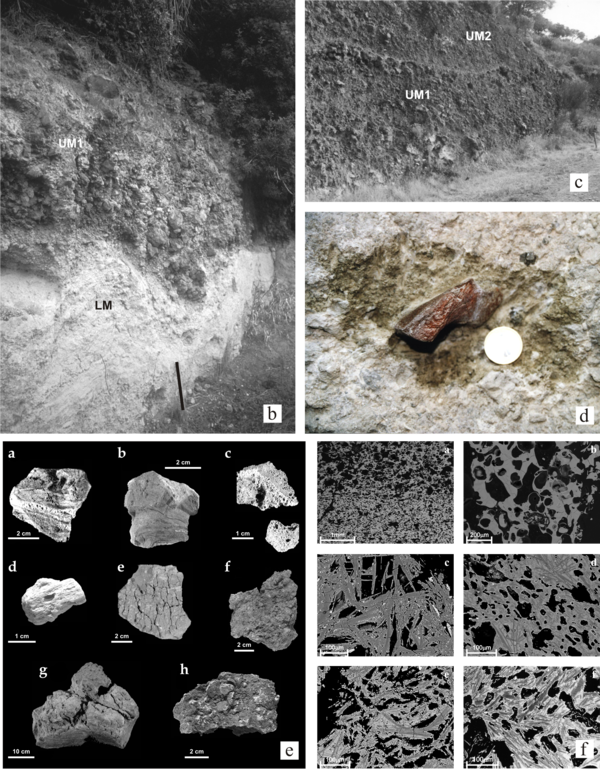
b) field photographs of MtN proximal deposits (from D’Oriano et al., 2005). Note the wavy, erosive contact between the Lower and Upper Members. Bar is 40 cm; c) Upper Member outcrop. The contact between UM1 and UM2 is evidenced by a change in the grain-size. Thickness of UM1 is about 2.50 m; d) fragment of manufactured product within the LM deposit; e) juvenile products of Lower Member (a, b, c, d) and Upper Member (e, f, g, h); a - banded pumice; b - banded pumice with obsidian-like texture; c - coarsely vesicular pumices; d - tube pumice; e - dense clast with superficial fractures; f - scoria-like clast; g - cauliflower bomb; h - agglutinated Clast (from D’Oriano et al., 2005); f) SEM-BSE images showing groundmass textures of juvenile products from Lower (a, b) and Upper Member (c, d, e, f): a - microvesicular and coarsely vesicular bands in banded pumice clast; b - coarsely vesicular pumice clast; c - dense holocrystalline clast; d - dense glassy clast, e - scoria-like fragment and f - glassy scoria (from D’Oriano et al., 2005).
The AD 1538 Monte Nuovo eruption
The AD 1538 Monte Nuovo (MtN) eruption is the most recent volcanic event of the Phlegrean Fields and the only one that occurred in historical times. It took place after 3.5 Ka of quiescence in the NW sector of the Phlegrean Fields caldera. Several contemporary eyewitness accounts (Del Nero, 1538; Delli Falconi, 1538; Marchesino, 1538; Porzio, 1551; Da Toledo, 1538) provide detailed information on precursors, timing and description of the eruptive phenomena. The MtN eruption was characterized by two main phases with contrasting eruptive styles. The deposits forming the body of the MtN cone consist of ash and pumice tephra; they are overlain by thin, dark-coloured, coarse-grained block and lapilli deposits. A wavy erosive surface separates these two units, called the Lower and Upper Member, respectively (Fig. 4b,c) (D’Oriano et al. 2005). The historical accounts of the event were thoroughly summarized by Parascandola (1946) and analysed in comparison with deposits by Rosi and Santacroce (1984) and Lirer et al. (1987).
The eruption started on Sunday 29 September around 8 pm from a vent near the village of Tripergole - 3 km west of Pozzuoli. During the first 2 days the activity was vigorous and consisted of quasi-continuous emission of ash-laden, black clouds which generated concurrent radially spreading pyroclastic density currents and a vertically rising convective column. During this phase the substantial involvement of groundwater in the eruption is suggested by the description of vapour-charged, white clouds and by the downwind fallout of muddy ash on the towns of Pozzuoli (~30 cm) and Naples (~2 cm), with fine ash dispersed to Calabria (~200 km SE). Wet deposition of the ash-rich deposits is evidenced by their incipient consolidation, occurrence of accretionary lapilli and mud coatings. After 2 days of activity the pyroclastic cone was almost entirely formed and an up 40 cm thick pumice raft was reported in the Bay of Pozzuoli. Activity had to be intense in the first 12 hours of the eruption because in this time span the cone was already largely formed (Del Nero 1538). The long-range ash dispersal reported by the chronicles (ash fallout up to a distance greater than 200 km) suggests strong convective power of the associated eruptive plume indicating a relatively high mass discharge rate. The first phase of the eruption corresponds to the first 2 days of activity (from 8 p.m. of 29 September to early afternoon of 1 October). The minimum volume of deposits emplaced in this phase is 3-6x107 m3 based only on the volume of the cone. Considering that the construction of the cone was largely completed after 12 h of activity (Del Nero, 1538), the minimum value for the mass discharge rate results of about 106 kg s-1 (D’Oriano et al. 2005).
On the morning of Tuesday 1 October the activity declined and then stopped completely. At that time, Da Toledo climbed the cone up to the crater rim observing some “boiling” in the crater bottom accompanied by moderate launch of stones. Around 4 p.m. on Thursday 3 October a new strong explosion generated dark globular clouds which overran the sea for a few miles, along with a scattered shower of blocks. After two more days of pause a new explosion occurred in the afternoon of Sunday the 6th, killing 24 people who were attempting to climb the new cone. In addition to thick burial around the vent, devastation around the cone was substantial. Da Toledo (1538) reports of trees being uprooted and covered by ash (pyroclastic density current activity?) up to Grotte di Lucullo, which, according to Parascandola (1946), was near Miseno, about 5 km south of Monte Nuovo, in the area of Capo Miseno. Marchesino (1538) who visited Pozzuoli in the afternoon of October 4, reported that 90% of the edifices were found destroyed or severely damaged as a result of the combined effect of ash load and seismic shaking. The minimum volume of the Upper Member is between 1 and 4x106 m3. The similar sedimentological features of UM2 and UM1 deposits suggest they were emplaced with similar dynamics during two independent events (D’Oriano et al. 2005).
Despite the identical K-phonolitic bulk rock composition of all MtN products (both LM and UM units), textures and microphenocryst compositions exhibit important differences. The juvenile material of LM member consists mostly of variable vesicular pumiceous lapilli and very subordinated cm-sized platy obsidian fragments. Foreign lithics account for less than 5 wt% of the deposit and are represented by fragments of the Neapolitan Yellow Tuff (Orsi et al. 1992). Chips of buildings and pottery from the pre-existing village of Tripergole as well as marine shells were also occasionally observed in the deposits (Fig. 4d). In LM, density is largely variable in each layer ranging from 400 to 2,000 kg m-3 throughout the sequence. This wide range reflects the large variability of clast morphological features. D’Oriano et al. (2005) recognized different clast types (Fig.4e, f): banded (42 vol%), coarsely vesicular (42 vol%), tube (8 vol%), and microvesicular pumices (8 vol%). Tube pumices have the lowest density while microvesicular have the widest density range. Banded pumices typically display the alternation of yellow and grey bands differing in their vesicularity. The latter are identical to microvesicular pumices while the former have textures very similar to coarsely vesicular pumices. Dense, platy obsidian fragments of up to 1–2 cm are scattered throughout the upper half of the sequence, probably resulting from the shattering of obsidian-bearing banded pumices. Bombs and blocks are represented by coarsely vesicular pumices and banded pumices within the lower 2/3 of the deposit and by microvesicular pumices at the top of the sequence.
The analysed samples of UM1 show a narrower density range than LM, from 700–2,100 kg m-3. The juvenile fragments are dark in colour, have heterogeneous textures and can be grouped into four main clast types: dense, scoria-like, transitional and glassy scoria. The first three clast types constitute UM1a and b (average frequency of 40, 37, 23%, respectively, of the analysed samples), while UM1c is only made up by glassy scoria. Transitional clasts include all fragments with morphological and textural characteristics between dense and scoria-like clasts. Most of them are agglutinated clasts formed by the welding of cm-sized, dense, blocky fragments and variably vesicular, irregularly shaped, scoria-like material. Some of the largest (m-sized) clasts have sets of parallel dm-long open cracks.
The large variability in density and clast and vesicle shapes of the LM juvenile fragments sheds some light into processes occurring in the volcanic conduit during the first phase of the eruption. Large density variations in juvenile material have been described both in purely magmatic eruptions (Gardner et al. 1998; Polacci et al. 2001; Rosi et al. 2004) and in deposits originated by magma/water interaction (Houghton and Wilson, 1989; Morrissey et al. 2000). The sedimentological and textural features of the LM deposits and the chronicle's accounts clearly indicate a prominent role of magma/water interaction during this phase of the eruption. Several lines of evidence, however, suggest that fragmentation was dominated by purely magmatic processes (mainly degassing and gas expansion). The contemporary occurrence of coarsely vesicular pumices, low-density, tube pumices and banded pumices reflects the disruption of a magma column characterized by a horizontal zonation of textural features. Banded pumices may originate in an outer collar-like region of the conduit, owing to mixing between melts with different viscosity (Freundt and Tait, 1986). The homogeneous magma composition suggests that differences in rheological properties were not produced by compositional variations but they should reflect a horizontal temperature gradient, with the cooler external region occupied by obsidian-bearing material which was physically mixed within the more fluidal rising magma. According to this conceptual model, coarsely vesicular pumices represent the central portion of the conduit.
Textures of the UM1 juvenile products suggest that they resulted from the disruption of a variably crystallized magma plug. Due to the short time (2 days) separating the two UM explosions from the preceding activity, we can assume quasi-closed system conditions for the degassing and crystallization history of the magma plug. Plug explosions occur as the response of a pressurization induced by a disequilibrium between volatile loss through permeable conduit walls and continuous gas accumulation in the inner crystal-rich part of the plug. When overpressure exceeded the tensile strength of the rigid, topmost part of the plug, blasting of this cap occurred, and gas hosted within the plug eventually expanded to lower pressure accelerating the fragments (Alidibirov 1994; Alidibirov and Dingwell 2000). The whole process can be envisaged as a layer-by-layer mechanical fragmentation due to an inward propagating decompressional wave. The process progressively dampened when the fragmentation front reached less viscous magma at the bottom of the plug. Crystallization due to massive cooling or to decompressional degassing enhances the volatile oversaturation, forcing gas exsolution and generating internal overpressure (Tait et al. 1989; Sparks, 1997). The sudden decompression of the partially crystallised and degassed magma body result in the emission of related to a Vulcanian style of activity (Morrissey and Mastin, 2000). The radial distribution of the pyroclastic flow deposits suggests they derive from the boiling-over of a dense cloud carrying a coarse mixture of plug fragments.
The shift from LM to UM activity can be attributed to a continuous decrease of the mass flux, triggering a feedback that contributed to decrease the frequency of the pulsatory activity. The decrease in the mass flux is a realistic hypothesis when considering that more than 90% of the total erupted volume was discharged during the first phase, implying an important decrease of the magma chamber overpressure (Folch and Marti, 1998). The shift between these two phases was also accomplished by a drastic change in the process of fragmentation. Fragmentation of magma during LM was dominated by conditions of rapid degassing during high magma ascent rate which promoted vesiculation and impeded crystal nucleation (Cashman and Blundy, 2000). On the other hand, the fragmentation of UM magma occurred under quasi-static conditions with the development of a highly crystalline, pressurized plug. The switch between the frequent explosions at the end of LM phase and the two distinct explosive events of UM may be related to non-linear variations of magma rheology. Viscosity of crystal-rich magmas shows a dramatic increase around a crystal content of 30–40% by volume (Lejeune and Richet, 1995). Overcoming of a crystal content threshold at the transition between LM and UM could have induced an important decrease of the magma ascent velocity favouring condition of magma crystallization along the conduit.
Mt. Etna
Mt Etna is mainly known for its persistent activity from its summit or from effusive eruptions originating from fissures on the flanks of the volcano. Tephra layers cropping extensively on the volcano flanks and adventives cones punctuating all the sectors of the volcano edifice witness an explosive activity of mid large intensity related to the recent activity of this volcano. The recent analysis of historical records of Branca and Del Carlo (2004) indicates that in the last four centuries at least 12 subplinan eruptions took place from that central crater while at least seven long-lasting, ash plume-forming explosive eruptions occurred from volcano flanks. Subplinan eruptions are generally impulsive, can last hours and produce several km-high eruptive columns and tephra fallout also of lapilli size at distances of several tens of kilometers. Explosive flank eruptions (Class B of Branca and Del Carlo, 2004) produce significant ash-plumes a few km-high from which a fallout of lapilli and ash extending for several tens of km from the volcano results. Though no casualties have been associated to this activity, these eruptions have had (in the past and also more recently) a significant impact on human activity and on the economy. We summarize here the main features of 2001 and 2002-3 eruptions, which are the most recent expressions of this flank-explosive activity. Such events have been and studied with modern methods and monitored with a large multidisplinary effort that include field survey, seismic, ground deformation, gas geochemistry, petrology, gravimetric an magnetic measurements and show large similarities.
The 2001 eruption
After eight years, and heralded by seismic swarms and geodetic, magnetic, and gravimetric anomalies, Mt. Etna resumed flank activity from 17 July - 9 August 2001. A complex system of eruptive fissures cut the NE and the S flanks of the volcano from 2900 to 2100 m elevation, producing extensive lava flows that buried infrastructure (mainly touristic) and forests down to 1035 m above sea level. Explosive activities of variable character took place from 18 July - 7 August in the form of hydromagmatic explosions, fire fountaining, Strombolian explosions and nearly sustained or pulsating ash explosions. Explosive vents were clustered in the southern reaches of the fissure system (at 2550 and 2100 m). Most of the tephra were erupted from a vent area at 2550 m elevation, forming a ≍100 m high scoria cone (Fig. 5b). Explosive activity at this vent area started with discrete, frequent hydromagmatic explosions and formed explosion craters. On July 24, activity shifted to Strombolian explosions and fire fountains forming, at first, two ill-defined and then one large scoria cone. In the final phase of the activity, from 1 - 5 August, the cone emitted pulsating tephra columns - initially continuous and gradually shifting to discrete explosions of decreasing frequency (order of minutes) and intensity (Fig. 5b). This activity deposited a thin blanket of ash that covered the cone and its surroundings, together with dense, non vesicular blocks. Possible small collapses of the ash column were also documented. During the entire eruption abundant ash was dispersed towards the S and E quadrant of the mountain by local winds causing the international airports of Catania and Reggio Calabria to be closed for some days. Lava flow from three emission points accompanied the explosive activity at 2550 vent in the period 25 July - 1 August (Fig. 5c).
Figure 5. 2001 and 2002 eruptions at Etna
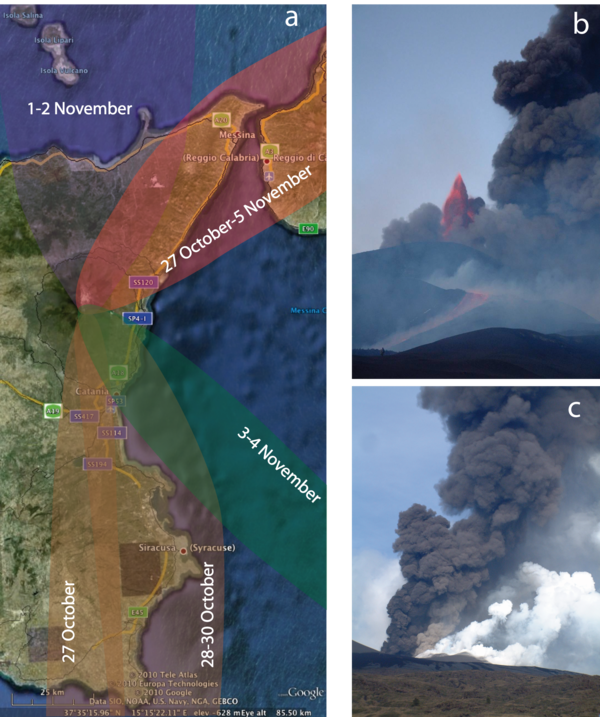
a) Distribution of the ash plume during the early days of 2002-3 Etna eruption. (redrawn from Andronico et al. 2005 and 2009). b) Fire fountains and ash emission from 2800 a.s.l. cone on S fissures, during mid November 2002. Compare the cone height with images from the video recorded on 27 October 2002 from the same position showing the very beginning of the activity on this fissure system; c) Contemporaneous emission of ash and vapour from 2800 a.s.l. cone on S fissures, at the end of November 2002. Picture taken from SW flank.
Figure 6. Summarizing table for the 2001 and 2002 eruptions at Etna
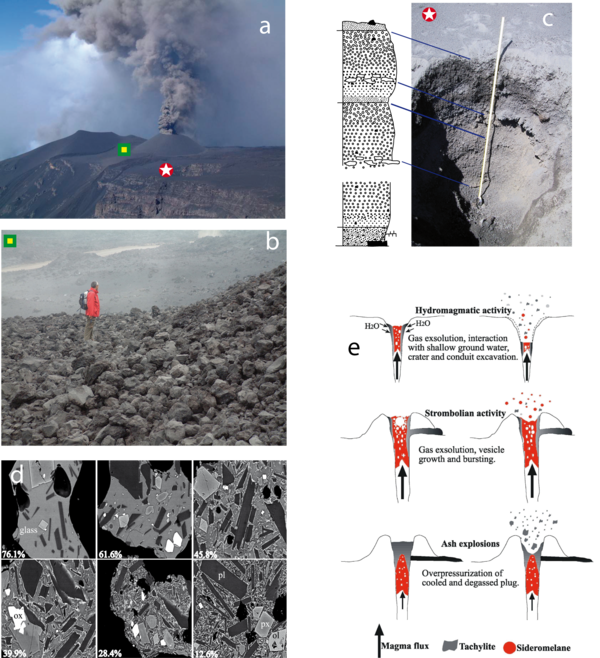
a) Aerial photo of Laghetto cone taken from NE with vigorous ash production on 1 August 2001. Lava flows poured out at the foot of the cone are also evident: Symbol report location of outcrops reported in b) and c); b) Dense blocks deposits at the foot of the Laghetto cone. These blocke are related to the last phase of the activity; c) Ash fall proximal deposit related to the 2001 eruption from Laghetto cone. Succession includes from the bottom: 1) lithic rich crudely layered (10 cm thick) of mm to a few cm-sized ash; 2) Two well sorted graded fall layers (220 and 70 cm thick) of cm-sized scorias and dm-sized bombs; 3) 20-cm thick layer mostly made of a few mm to mm-sized ash. Bar is 2 m long.(Redrawn from Taddeucci et al., 2003); d) SEM-BSE images of ash showing a large range of glass abundance in percentage (white digits) (redrawn from Taddeucci et al 2004); e) Conduit processes during the different stages of 2001 eruption as inferred by textural and compositional study of Taddeucci el al 2004.
The variability of explosive activity and the intense monitoring of the summer 2001 Etna eruption provided an exhaustive data record, making this eruption a favourable case study for the transitions between different types of explosive basaltic activity. In particular, tephra were largely investigated under several point of view, including deposit composition, texture grain size, plume dispersal and transport, volume, ground distribution, etc. (Taddeucci et al. 2002; Taddeucci et al. 2004a, 2004b; Scollo et al. 2007). Monitoring of ash componentry and related physical and chemical properties of the magma emitted during eruption were also employed to interpret transitions between eruptive styles. In ash samples, the following components have been recognized (Taddeucci et al. 2002): i) gray-black, opaque tachylite clasts, not or poorly vesiculated, equant and often blocky in shape, appearing micro- to crypto-crystalline in thin section; ii) transparent, light to dark brown sideromelane glass, non-vesicular to highly vesicular, that exhibit variable shape and smoothed surface; iii) felsic (plagioclase) crystals and mafic (pyroxene and olivine) crystals; v) altered volcanic and sedimentary lithic clasts. Fine ash aggregates were also found in samples erupted on 19-21 July. Microlite content increases gradually from sideromelane to tachylite, and the two components often grade into one another (Fig. 6b).
Both proximal stratigraphy (studied on field after the eruption) and the daily distal ash records show that eruption products varied in time accordingly with the three activities observed at the vent. In stratigraphic columns (Fig. 5d) from bottom to top we observe: a basal deposit enriched in oxidized (mainly volcanic) lithics and blocky ash with occasional hydration cracks and ash aggregates; a thick middle zone dominated by vesicular, glassy pyroclasts, both scoria and ash; a thin top layer of poorly vesicular to unvesicular, microlite-rich, dense and blocky juvenile pyroclasts. Dense blocks are mostly found at the slope break at the base of the cone where they form a continuous belt likely created by rolling blocks that landed on the cone flanks. This deposit architecture indicates that dense blocks were erupted during the last phase when the cone had already formed. The juvenile origin of the blocks is clearly shown by the presence within them of sedimentary inclusions that mark other products of this eruption (Corsaro et al. 2007).
The observed features and stratigraphy of the eruption deposit, and the temporal trend of the activity, led Taddeucci et al. (2002) to the following inferences on the explosive processes during the three periods identified. First period: blocky shape of the ash particles, abundance of lithics, and the occurrence of aggregates and hydration cracks are typical of explosions caused by magma-water interaction (e.g. Büttner et al. 1999; Doubik and Hill, 1999), as well as the jets observed at the vent. When the eruption started, magma reached local water tables causing hydromagmatic explosions that widened the conduit and opened the new vents. Second period: vesicular sideromelane and fuse-like bombs that formed during Strombolian and continuous fire-fountaining activity are indicative of efficient magma degassing at fragmentation in fully magmatic activity (e.g., Heiken and Wohletz, 1985). As magma flux and pressure increased, water was cut off from the conduit and the activity becomes fully driven by magma degassing. Third period: the presence in the deposit of dense blocks and ash rich in poorly vesicular, microlite-rich tachylite implies fragmentation of a relatively cooled and degassed magma. The final part of the eruption involved the top of a largely degassed melt column, whose fragmentation was controlled by the concomitant increase of pore pressure and yield-strength of the cooling and crystallizing magma in a kind of small-scale, vulcanian-like eruptive mechanism (Nairn and Self, 1978; Self et al. 1979). Common aspects of the third phase with Vulcanian eruptions are the observed activity style, with continuous to discrete and cannon-like ash explosions (especially in the last days of activity) and the occasional occurrence of collapses of the denser parts of the ash column. Also the deposit shares features with Vulcanian ones - such as the thin ash fall layer together with large ballistic, dense blocks.
Crystal size distributions and textural investigations showed that sideromelane implies a simple crystallization history, probably due to single (or shorter) nucleation and microlite growth event, tachylite represents a subsequent, more complex crystallization stage of the magma in respect to sideromelane. During the 2001 flank eruption of Mt. Etna, the tachylite crystallization took place in increasing disequilibrium conditions, and, possibly, after a ~40°C temperature decrease.
Compositional, CSD, and vesiculation features of the ash particles indicate that tachylite-forming magma experienced a longer residence time in the upper conduit in respect to the sideromelane-forming one. Residence time is controlled by ascent velocity. The evolution of the explosive activity suggests that sideromelane formed by fragmentation of the central, microlite-poor, still vesicular, and hotter zone of magma flow where rising magma slugs transported magma at a relatively high velocity. Conversely, tachylite formed by fragmentation of the microlite-rich, denser, and cooler magma formed by vesicle collapse in the peripheral zone of the magma flux. The relative abundance of sideromelane and tachylite ash particles in the eruption deposit records the evolution of velocity gradients in the rising magma flux. Both of these ash particle types erupted simultaneously (over a 24 hours time window) during the Strombolian activity, but sideromelane content in the ash gradually decreased as the eruption evolved until the final, pulsing ash explosions erupted only tachylite ash and dense blocks. In this view, the transition from Strombolian to small-scale, vulcanian-like pulsing ash explosions may result from the progressive development of the peripheral zone until it formed a rigid, permeable, fractured plug of degassed and crystallized magma at the top of the magma column (Fig. 5f). Fragmentation of the plug also produced dense blocks with irregular, sometimes altered surfaces, likely remnants of the permeable network of fractures left by cooling and vesicle escape and collapse. The increased development of the peripheral zone is a consequence of a decrease in the magma emission rate and the two processes jointly lead to the closure of the vent and the end of the eruption.
From the point of view of the hazard related to the ash, though with different eruptive mechanisms, strong ash production is related to the opening and closing stages of the eruption and are strictly related to the physical properties of the magma that increase the efficiency of the brittle fragmentation of the magma.
The 2002 eruption
On 26 October 2002 at 20:12 GMT, an earthquake swarm (Patanè, 2002; Acocella et al. 2003) preceded and accompanied the formation of eruptive fissures over the S and NE flanks of the volcano, marking the onset of the 2002-03 Etna eruption. A north-south, 1 km-long eruptive fissure opened on the upper southern flank of the volcano (2850-2600 m a.s.l.), between Torre del Filosofo and the old cable-car station partially destroyed during the 1983 eruption. Another NE oriented 4 km long fissure developed on N flank, from northern base of NEC (3010-2920 m a.s.l) down to 1900 m. Detailed eruption chronology together with compositional and gas geochemical data are reported in Andronico et al. (2005). North fissure system was active until 5 november producing fire fountains, Strombolian activity and lava flows. The southern fissure produced 100-300 m high fire fountains that soon evolved into an ash column up to about 5 km a.s.l. (see movie) that was blown south by the wind spreading on the Catania and other south eastern Sicily towns. Fine ash reached the Northern Africa and Greek islands (Dellino and Kyriakopulos, 2003). Eruptive activity at the S fissure lasted until 28 January 2003, showing several changes in the eruptive style and a two week pause in lava effusion. This kind of activity (tephra dispersal, nature and volume of the deposits) were reported in detail and evaluated by several papers (Andronico et al. 2008, Andronico et al. 2005; Andronico et al. 2009).
Early days of the eruption were characterized by an alternation of lava fountains and phreatomagmatic explosions forming dense ash columns reaching an height of 4.2 km on the vents with mass eruption rates varying from 2.2 to 4.9 104 kgs-1 (Andronico et al. 2008). After that, ash-rich eruptions columns alternated to Strombolian explosions. The latter became dominant after end of December 2002. Ash fallout covered all sectors of the volcano with the exception of the western flank and extended southward up to Siracusa (80 km) and Ragusa (100 km) or northward up to Reggio Calabria (Fig. 5a). Ash fall concentration at the ground attained during the early days 18 kg/m2 at Rifugio Sapienza (S flank, 1900 m.a.s.l.) several kilos (up to 6 kg/m2 in Zafferana) in the mid-lower flanks and about 1 kg/m2 at Catania. Ash plume and tephra strongly affected commercial flights (airports of Catania, Reggio Calabria and Sigonella), main roads, highways and cultivated areas.
As in the 2001 eruption, ash juvenile components (mostly tachylite and sideromelane, Andronico et al. 2009) show that the relative abundance of tachylite and sideromelane and the morphology of the particles show large variations. And in particular, sideromelane abundance increases with episodes of Strombolian activity.
With regard to eruptive mechanisms (also for the 2002 eruption), crystallization occurring in the topmost part of the conduit seems to have a strong control on the efficiency and the style of magma fragmentation, hindering gas segregation and leading toward a brittle regime that increases ash production, and contributing to determining the eruptive style at the vent.
Stromboli Island
The typical activity of Stromboli, for which the volcano is famous, consists of mild intermittent explosions, ejecting incandescent scoriae, magma lumps, ash and blocks to heights of a few tens to hundreds of meters. Most of the coarse ejecta falls back into the craters although some are expelled up to 200 m from the source vent.
Figure 6a-b. 5 April 2003 paroxysm at Stromboli
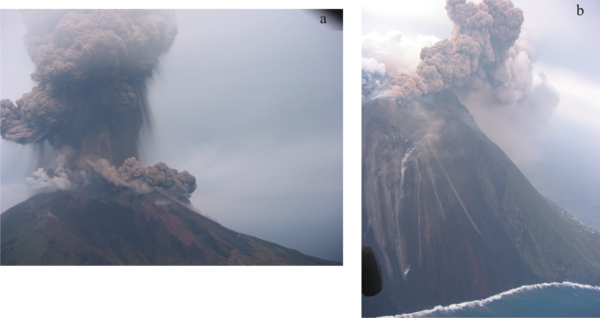
a) Convective column generated during Phase 2 and co-ignimbritic clouds related to pyroclastic flow formation during Phase 3; b) ash plumes emitted during the late Phase 4 (pictures courtesy of S. Calvari).
Every 10-20 years during the past two centuries, lava effusions occurred either from the summit craters or from vents opened inside the Sciara del Fuoco - a collapse scar on the NW flank. Lava flowed onto the Sciara slope eventually reaching the sea and without causing concern to the population. Both mild Strombolian explosions and lava flows are fed by a highly porphyritic (HP), degassed HK-shoshonitic basalt residing in the shallow part of the feeding system.
The most violent and hazardous eruptive manifestations of the volcano are represented by the Strombolian paroxysms (Mercalli, 1907). They usually consist of sequences of explosive events lasting from a few minutes to days. Ballistic bombs and blocks are ejected up to a distance of a few kilometres from the craters, sometimes affecting the two villages on the coast. The impulsive emission of jets of gas and pyroclasts evolves into short-lived convective columns depositing ash, pumice lapilli and bombs on the volcano slopes up to the sea. Significant morphological modifications of the crater area and the formation of deep craters are also commonly observed (Bertagnini et al. 2008). The distinctive feature of paroxysms is the emission of a low porphyritic (LP), volatile-rich HK-basalt as highly inflated, light colored pumice. Pumice are variably mingled with the HP residing magma (Fig. 6 g, h) and unmingled HP scoriae are present as well. In the last 8 years the volcano has produced two lava effusions (December 2002 - July 2003 and February - April 2007, respectively) and two paroxysms (on 5 April 2003 and 15 March 2007, respectively) while the effusive eruptions were in progress.
Figure 6c-h. Summarizing table for the 5 April 2003 paroxysm at Stromboli
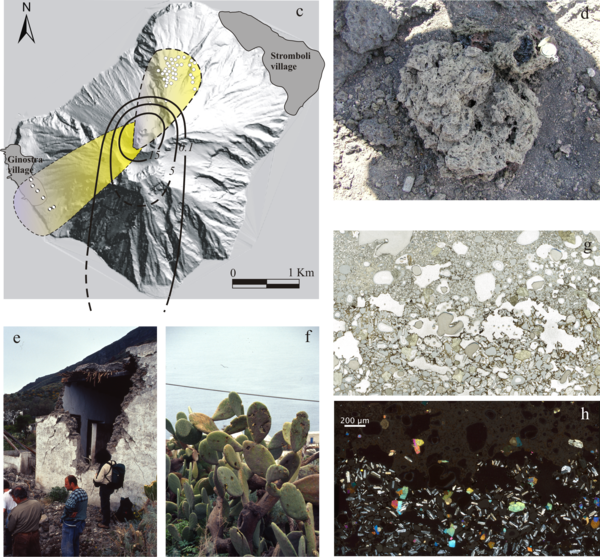
c) Mapped impact craters from larger ballistic blocks (yellow-colored areas indicate the higher concentrations of blocks) and isomass lines (values are in kg/m2) of fallout deposit related to Phase 2 (modified from Pistolesi et al, 2008); d) Highly vesicular LP pumice bomb sampled at about 500 m from the crater area (picture courtesy of N. Métrich); e) house in Ginostra village damaged by a m-sized block ejected during Phase 2; f) shards of a block fragmented after impact and jabbed in prickly pears near Ginostra village; g) and h) optical microscope pictures (open-nicol and cross-nicol, respectively) of a mingled clast, highlighting the difference between the highly vesicular and nearly aphyric LP and crystal-rich HP magmas.
Strombolian paroxysms: the case of the 5 April 2003 eruption
The 5 April 2003 paroxysm was observed by several researchers present on the island and a detailed event chronology was reconstructed through data from thermal camera, digital photos and a thermo-acoustic-seismic array (Calvari et al. 2006; Ripepe and Harris, 2008; Harris et al. 2008). The integration of these data with field and laboratory analyses (Rosi et al. 2006; Pistolesi et al. 2008) provided a quantitative picture of the explosive dynamics, while geochemical, mineralogical and melt inclusions studies characterized nature, deep of provenance and ascent dynamics of the emitted magmas (Métrich et al. 2005; Francalanci et al. 2008). The paroxysm of 5 April 2003, the most violent of the past 50 years, had a total duration of about 373 s and progressed through four phases:
1) Eruption onset (~30 s). The eruption began with a weak emission of red ash to a height of a few tens of meters, from the NE and central craters. After 17 s the emission became more intense and cauliflower grey clouds rose from the NE crater.
2) Climactic explosion (~38 s). The second phase began with the emission of a rapidly expanding, dark-colored ash plume that was, seconds later, over taken by a second plume composed of multiple jets, comprising leading blocks with ashy contrails (Fig. 6a). The explosions involved simultaneously both SW and NE craters and fed an up to 4 km-high convective column. Meter-sized ballistic blocks were launched up to 1400 m above the craters and fell on the volcano flanks and on the village of Ginostra, at about 2 km from the vent (Fig. 6c, e, f). The areal distribution was strongly asymmetrical, in two narrow sectors oriented to NE and WSW respectively, such as in the large paroxysm of 11 September 1930.
The convective plume drifted southwards and the resulting fallout deposit consisted of variably expanded and mingled LP pumice clasts, HP scoriae and minor lithic fragments. Across the summit area, a moderately sorted, incipiently welded spatter deposit was emplaced, hot avalanche deposits limited to the crater area, resulted from sliding of fallout deposit accumulated on steep slopes.
The most of the pyroclastic material was emitted during this phase. From the isomass map (Fig. 6c) a total erupted mass of 1.1 – 1.4 x 108 kg was calculated, with erupted magma accounting for 0.8 – 1.1 x 108 kg. The average mass discharge rate was calculated in 2.8–3.6 × 106 kg/s, with a possible peak at 1.0-1.2 × 107 kg/s in the 10 s-long initial gas thrust. During this phase gas velocities peaked at 324 m/s when large blocks were ejected at velocities of up to 185 m/s. The overpressure needed to accelerate gas and blocks to this value was calculated at 3.8 MPa, one order of magnitude higher than the gas overpressure (~0.2 MPa) estimated for the mild explosions of the persistent Strombolian activity (Vergniolle and Brandeis, 1996).
3) Pyroclastic flow and smaller explosions (~75 s). This phase was dominated by the formation of a scoria flow and the concurrent rise of co-ignimbrite clouds (Fig. 6b), possibly accompanied by a series of explosions from the head of the dyke which was feeding the lava flow. The mass of the scoria flow deposit was estimated at about 1.0-1.3 x 107 kg. A 175 s long hiatus followed, characterized by low intensity pulses from the SW crater.
4) Terminating ash explosions (~87 s). Three main discrete explosions at SW crater emitted red colored, ash-laden plumes with maximum height of ~600 m.
Pumice (Fig. 6d) emitted during the paroxysm has a HK basaltic composition as their analogs erupted by the earlier paroxysms and similar mineralogical and textural features. It carries up to 15-20 vol% of crystals in a glassy groundmass. As verified in all previously studied eruptions, most of them are inherited from the shallow crystal-rich magma during syn-eruptive mingling or show resorption features indicating reactions between LP melts and cumulative body/crystal mush.
A few olivine crystals (Fo86-87) host melt inclusions with a volatile content of 3.1 wt% (H2O, CO2, S, Cl), for which the total fluid pressure is evaluated between at about 200 MPa. It suggests that the paroxysm was initiated by the uprising of a small magma blob from a depth of about 8 km. The same depth has been estimated for the 15 March 2007 paroxysm (Métrich et al. 2010).
The H2O and CO2 evolution in melt inclusions in these recent paroxysms and earlier events indicate that they are related to the ascent of volatile-rich magma batches, under prevalently closed-system decompression conditions, from the storage zone lying at ~7-10 km depth. The low microlite content of the pumice suggests that decompression and ascent are fast enough to prevent crystal nucleation and growth (Bertagnini et al. 2003; Métrich et al. 2010).