Chapter 4. Mechanical stratigraphy and style of deformation
The structural style of both compressional and extensional structures of the NA is strongly influenced by the mechanical anisotropy within the upper crust. In fact the sedimentary cover is composed by alternating competent and not-competent units, whilst the shallower part of the basement often correspond to a low-Vp horizon, interpreted as a mechanically weak layer (e.g. Brogi and Liotta, 2008; Mirabella et al., 2008).
In the AD, the Miocene-Quaternary compressional structures affect the Umbria-Marche Mesozoic-Tertiary succession, widely described in the literature (e.g., Centamore et al., 1986; Cresta et al., 1989, and references therein). From a mechanical point of view, this succession can be divided into four main litho-structural units. top to bottom: turbidites, carbonates, evaporites and basement s.l. (fig. 5). The upper part of the "basement" includes Permian-Middle Triassic clastic rocks (e.g. Verrucano Formation) and/or Late Palaeozoic meta-sedimentary rocks. The mechanical boundaries among these units correspond to the main décollements of the compressional structures, as well as to the best seismic markers, driving the interpretation of the seismic profiles.
Figure 5. Thrust trajectory and mechanical stratigraphy of the sedimentary cover in the Adriatic Domain.
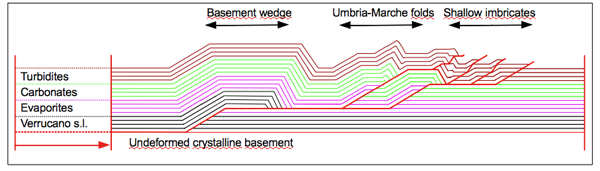
Schematic view of a thrust fault propagating through the Umbria-Marche succession, generating a set of progressively shallower structures, bottom to top: basement wedges (décolled within the upper part of the basement s.l.), Umbria Marche folds (décolled within the Late Triassic evaporites) and shallow imbricates (décolled at the top of the carbonates).
The stratigraphic and structural setting of the TD upper crust is more complex, consisting of a thick stack of embricated Liguride and Tuscan Units, successively dismembered by the Miocene-Quaternary extensional structures (e.g. Carmignani et al., 1994 and this volume; Molli et al., this volume). Beyond the structural complexity, this also results into a mechanical alternation of competent (e.g. Macigno, Tuscan carbonates and dolomites) and less competent (e.g. Ligurids, evaporites, phyllites) units.
Structural style of the compressional belt
Contrasting interpretations of the structural style of the Umbria-Marche fold and thrust belt have been proposed by different Authors, mainly by integrating surface geology data with seismic reflection profiles (e.g. Ghisetti et al., 1993 for a discussion). The proposed interpretations can be divided into two main groups. A first group of authors interpreted Umbria-Marche Apennines as a typical thin-skinned thrust belt, where thrusts developed in a regular, piggy-back sequence, over a main basal décollement, corresponding to the Triassic evaporites (Bally et al., 1986; Calamita and Deiana, 1986; Hill and Hayward, 1988). A second group recognized the involvement of the basement in the major thrust sheets (Lavecchia et al., 1987; Sage et al., 1991; Barchi, 1991; Barchi et al., 1998b), some of these invoking the reactivation of previous normal faults (tectonic inversion, e.g. Coward et al., 1999; Butler et al., 2006). Contrasting interpretations of the basement geometry and depth, and of its involvement in the major thrust sheets, have also been inferred from aeromagnetic data (Arisi Rota and Fichera, 1985; Cassano et al., 1998; 2001; Speranza and Chiappini, 2002).
The style of deformation beneath the main mountain ridge of the AD is effectively imaged by a portion of the CROP03 profile, where two prominent reflections can be recognised, corresponding to the Marne a Fucoidi Formation (close to the top of the carbonates) and to the top of the basement s.l. (fig. 6). Other reflectors (e.g. top evaporites, top carbonates multilayer, Messinian evaporites) can also be traced locally.
At depth, at least the shallower part of the basement is involved in the major thrust sheets, progressively deepening towards the east. Above these structures, the Marne a Fucoidi reflections describe a set of wide anticlines (involving the carbonates and the evaporites) whose geometry and wavelength resemble the typical Umbria-Marche folds, cropping out in the main mountain ridge. The shallower part of the section, above the Marne a Fucoidi seismic marker, is characterized by numerous reflections, imaging a complex pattern of short wavelength structures disharmonically overlying the carbonates.
Figure 6. Interpretation of a portion of the CROP03 profile across the main mountain ridge of the Apennines (modified after Barchi e al., 1998).
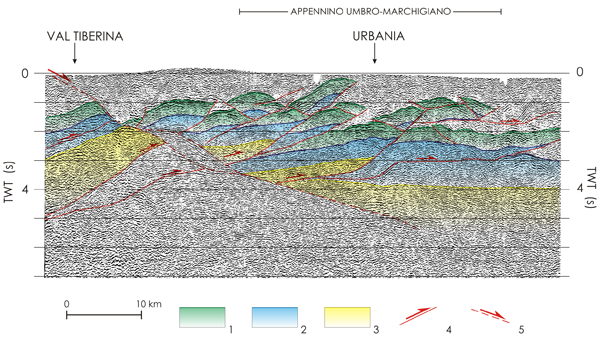
The seismic profile illustrates the style of deformation of the subsurface structures of the Umbria-Marche Apennines, in particular the relationships between the basement wedges and the Umbria-Marche folds.
This structural setting supports a style of deformation, characterized by a system of multiple décollements, where different sets of structures are generated at different structural levels, linked to each other and developed in a hierarchical mode. The size (i.e. wavelength and amplitude) of the structures depends on the depth of the décollement, which they sole to, so that the deeper the décollement, the larger the structures (fig. 5).
The most important classes of structures seismically and geologically detectable are:
shallow imbricates, detached on the top of the carbonates and involving the turbidites; along the section of figs. 6 and 7, these structures show a wavelength of few hundreds meters;
Umbria-Marche folds, detached at an intra-evaporites level and involving the carbonates, with structures that have a 5 to 10 km wavelength;
basement thrusts, corresponding to the major structural units of the region (having a 25–35 km wavelength) and that transfer the shortening from the upper part of the basement up to the sedimentary cover.
The structures generated at different structural levels may be connected along asymmetric major thrust systems, propagating across the whole upper crust, from lower towards upper crustal levels and branching into a progressively larger number of splays (fig. 5), so that shallower structures are more numerous and possess a shorter wavelength than the corresponding deeper structures.
The relationships between the Umbria-Marche folds and the shallow embricates are also illustrated in the geological section of fig. 7, crossing the culmination of a NE verging anticline (M. Petrano anticline) in the inner ridge of the Umbria-Marche Apennines. The M. Petrano anticline involves the entire carbonates succession and is décolled in the underlying evaporites. The major thrust propagates upward into a flat trajectory in the marly horizons at the top of the carbonates, splaying out into a set of small, narrow, shallow seated embricates.
Figure 7. Geological section throuh the inner ridge of the Umbria-Marche Apennines (modified after Massoli et al., 2006).
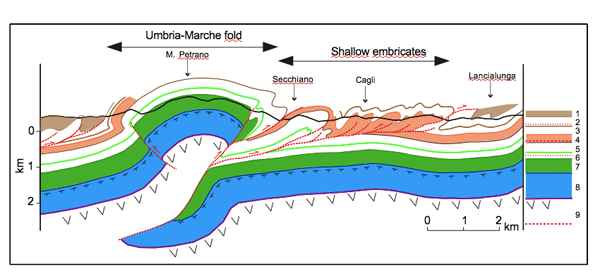
The geological section illustrates the style of deformation of the shallow structures of the Umbria-Marche Apennines, in particular the relationships between the Umbria-Marche folds and the shallow imbricates.
1- Marnoso-Arenacea; 2- Schlier; 3- Bisciaro; 4- Scaglia Cinerea and ScagliaVariegata; 5- Scaglia Rossa and Scaglia Bianca; 6- Marne a Fucoidi; 7- Jurassic multilayer; 8- Calcare Massiccio; 9- Anidriti di Burano. The red dotted lines mark the main décollements.
This style of deformation is also supported by many other studies, mostly published in the last 20 years and based on shallower commercial profiles, crossing both the mountain ridge and the adjacent deformed foredeep, from the Po Plain to the Marche-Adriatic region (e.g. De Donatis et al., 1998; Turrini et al., 2001; Massoli et al., 2006).
It is important to note that the wavelength of the embricated structures, detached above the Mesozoic-Paleogene carbonates, depends on the thickness of the syn-orogenic clastics. Where the Tertiary clastic succession is very thick (e.g. Po Plain, Pescara basin), the “shallow” structures show a much longer wavelength (4.5 to 8.2 km, Massoli et al., 2006) than in the inner part of the Umbria-Marche fold and thrust belt (e.g. fig. 7).
Structural style of the extensional belt
As the mechanical stratigraphy of the Umbria-Marche succession affects the flat-ramp-flat geometry of the compressional structures of the AD, the complex stratigraphy of the Tuscan upper crust also affects the geometry of the major east-dipping normal faults of the TD, which are characterised by a marked staircase trajectory, influencing the deformation of the hangingwall blocks and the evolution of the hinterland basins as well (fig. 8).
The presence of low-angle normal faults in the Tuscan mainland was initially hypothesised to explain anomalous stratigraphic relationships in the Mesozoic successions (i.e. “serie ridotta”; e.g. Lavecchia et al., 1984). Successively, in the geothermal areas of Southern Tuscany the availability of large data-sets of surface and subsurface data (seismic reflection profiles, calibrated by a dense network of boreholes) allowed many Authors to reconstruct in detail the down-dip geometry of the major extensional detachments, producing asymmetrical boudinage of the involved crustal volumes and the “lateral segmentation” of the competent units (e.g. Brogi and Liotta, 2008), with main flats within (or at the base of) the Liguride units and the Triassic evaporites (Calcare Cavernoso), and the ramps cutting trough the carbonates of the Tuscan Units. At a larger scale, low-angle detachment with staircase trajectory produce the boudinage of the entire upper crust (Brogi, 2004; Brogi et al., 2005).
A pronounced staircase trajectory also characterises the still active ATF, whose geometry has been reconstructed over an area of about 1800 km2 using a network of seismic reflection profiles (Barchi et al., 1999; Chiaraluce et al., 2007). In this case, the main décollements driving the curvi-planar geometry are located at the top of the carbonates and within the phyllitic basement. Since the ATF may be considered a seismogenic fault, its staircase geometry potentially produces down-dip segmentation of the fault, thus affecting the dimensions of the active fault segments and the maximum expected magnitude, to be considered for seismic hazard evaluation.
Figure 8. Normal faults with staircase trajectory and related hinterland basin in the Tyrrhenian Domain (modified after Brogi and Liotta, 2008).
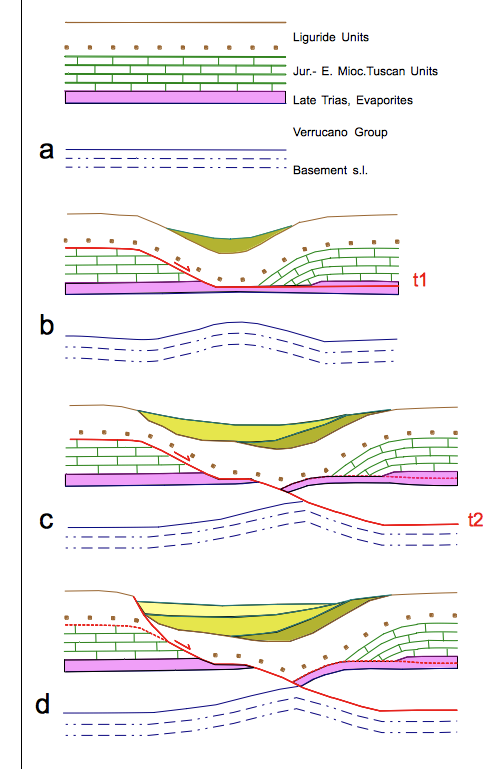
a- schematic stratigraphy of the TD; b- the movement along the t1 detachment produces lateral segmentation of the Tuscan carbonates and the onset of a bowl-shaped basin within a syncline ramp; c- the movement along the t2 detachment produces further extension and the enlargement of the basin; d- high-angle faults, splaying out from the main detachment propagate up to the surface: the basin evolved into a semi-graben, driven by a major east-dipping detachment.
The staircase trajectory of the major detachments can have significantly affected the onset and evolution of the hinterland basins, generated at their hangingwall (fig. 8). The initial bowl-shaped geometry of the hinterland basins has been used to support the hypothesis of a syn-compressional development of the hinterland basins (e.g. Bonini and Sani, 2002). However, this geometry can be also explained in an extensional context: in fact, bowl-shaped basins may be generated within ramp synclines at the hangingwall of curvi-planar normal faults, as hypotheised by Brogi and Liotta (2008) for the Radicondoli basin; alternatively, the upward propagation of normal faults can produce the dragging of the syntectonic strata along the basin flank (extensional fault propagation folds, e.g. Withjack et al., 1990), as illustrated by Sachpazi et al. (2003) for the faults bordering the Corinth Gulf basin.