Geochemistry of the Mafic Complex
Most of the numerous papers discussing the geochemistry of the Mafic Complex are based on samples collected along the Sesia and Mastallone Valleys, which cross-cut the upper Mafic Complex and paragneiss bearing belt. Rivalenti et al. (1975, 1981, 1984) demonstrated that whole-rock and mineral major-element data indicate open-system crystallization of the Mafic Complex. Across the basal, intermediate and upper zones, Mg/Mg+Fe decreases with height in the section from 0.78 to 0.35 although numerous stratigraphic oscillations occur that are similar in scale to those in ophiolitic gabbros. Mg/Mg+Fe ratios in the main gabbro are more restricted (0.58 to 0.35), suggesting that crystallization occurred under approximately steady-state conditions.
Voshage et al. (1990) first showed that igneous rocks within the paragneiss-bearing belt (their basal and intermediate zones) have initial Nd° and Sr° isotopic ratios that display considerable variation, as opposite to a relatively more homogeneous isotopic composition of the upper Mafic Complex (their Main gabbro and “Diorites”). Within the paragneiss-bearing belt, Voshage et al. (1990) report a few gabbros with isotopic compositions compatible with uncontaminated, mantle-derived melts (Sr° 0.702 to 0.704, εNd 6.2 to 7.2), which show MORB-like, depleted REE patterns. Sinigoi et al. (1996) report an additional sample with these MORB-like characteristics within the paragneiss-bearing belt and close to the mantle peridotite.
Although some of these uncontaminated samples could be interpreted as older mafic granulites unrelated to the Mafic Complex, one sample, TS4 (Mayer et al., 2000) is an undeformed gabbroic dike that intrudes the Balmuccia peridotite border zone from outside, cross-cutting the intense spinel foliation and boudinaged Cr-diopside and Al-augite dykes and bands. The TS4 dike clearly post-dates the emplacement of the mantle tectonite in the deep crust and appears to have preserved its primary composition, with Sr°=0.7021 (Voshage et al., 1988) and depleted REE patterns (Fig. 12) as a consequence of intruding peridotite rather that isotopically evolved paragneiss. Dike TS4 provided an internal Sm-Nd isochron of 274 Ma (Mayer et al., 2000) consistent with slow cooling after emplacement of the entire Mafic Complex at about 288±4 Ma (Peressini et al. 2007). Thus its intrusion is bracketed within the emplacement of the mantle tectonite and the cooling of the Mafic Complex, an age difficult to be unrelated to the main Permian event. These considerations support the interpretation of Voshage et al., (1990) that the first mantle melts intruded into the IVZ deep crust quenched too rapidly for their compositions to be influenced significantly by crustal contaminationSubsequently, after repeated intrusions heated the country rocks above their solidi, intruding mantle melts were contaminated by isotopically evolved crust.
Figure 12. REE patterns
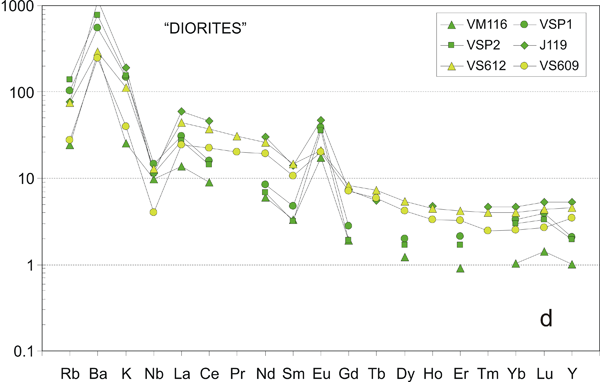
REE patterns of samples collected along Val Sesia. 12 a: uncontaminated gabbros in the Paragneiss bearing belt (Basal Zone of Rivalenti et al.,1984). 12 b to d: gabbroic cumulates at the bottom of upper Mafic Complex, Main gabbro and “Diorites.”
Excluding the few uncontaminated gabbros discussed above, the isotopic and trace-element compositions of most igneous rocks of the Mafic Complex indicate high degrees of crustal contamination. Voshage et al. (1990) showed that the εNd of the upper Mafic Complex (their Upper Zone, Main Gabbro and “Diorites” according to the stratigraphy of Rivalenti et al., 1984) was relatively uniform and concluded that these rocks had crystallized from a magma chamber that had achieved a thermal balance between magma input, anatexis, assimilation and crystallization. Sinigoi et al. (1994) extended the analysis to include Sr and O isotopic compositions and trace element abundances. Most of the Mafic Complex is characterized by Sri > 0.706, δ18O > 7.5 enrichment in LREE and an enrichment in Ba relative to K and Rb (Figs. 13 and 14). The absence of this characteristics in rocks with Sri < 0.704 indicates that they are an effect of crustal contamination and that the contaminant was enriched in Ba relative to K and Rb. Mazzucchelli et al. (1992) found positive Eu anomalies in plagioclase, clinopyroxene and garnet at deep levels of the upper Mafic Complex (their upper zone), which they interpreted to indicate that the melt that crystallized these phases had acquired a positive Eu by crustal contamination. Ba and Eu anomalies are in general positively correlated, indicating contamination by a biotite- and feldspar-enriched source that was previously depleted in Rb and K by dehydration melting and separation of anatectic melt. Positive Ba and Eu anomalies are also characteristics of the charnokites present as dikes or bands in the paragneiss bearing belt, which are interpreted as anatectic melts delivered from the septa. Similar Eu-enriched charnockites are described in other parts of the Ivrea-Verbano Zone (Schnetger, 1988) and in other granulite terranes (e.g., Pride and Muecke, 1982; Barbey et al., 1990; Harris et al.,1986). Sinigoi et al. (1995) concluded that the septa must have been stripped in K and Rb by fractional melting before being peeled off from the KF and incorporated into the Mafic Complex, according to the process driven by evolving density contrast previously described.
Figure 13. Ba, δO and Sr° vs. stratigraphy
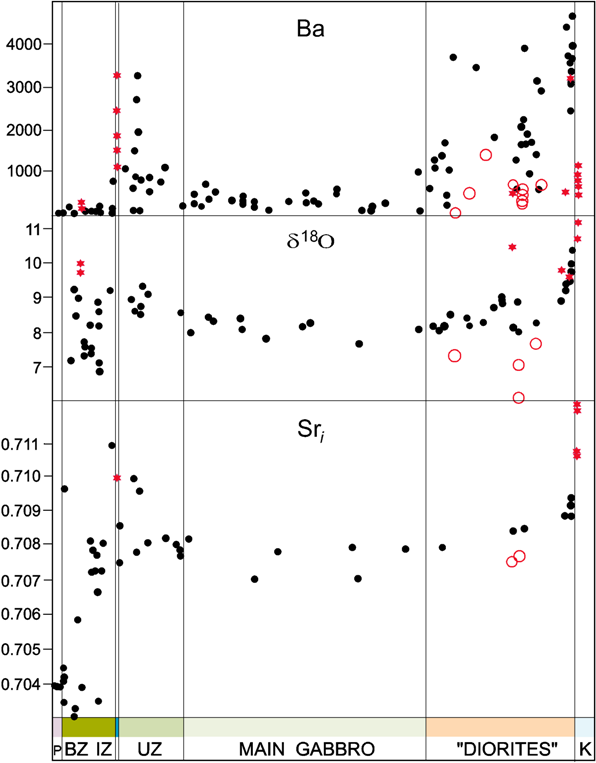
Ba contents (ppm) and isotopic composition of O and Sri (87Sr/86Sr calculated at 270 Ma) plotted against the stratigraphy of the Mafic Complex in the Sesia Valley. Subdivisions of the Mafic Complex are the same as in Figure 12. Solid circles, gabbro, norite and diorite; solid red stars, charnockite or leucosomes; open red circles, mafic enclaves in dioritic rocks. (From Sinigoi et al., 1994).
Figure 14. Normalized incompatible elements
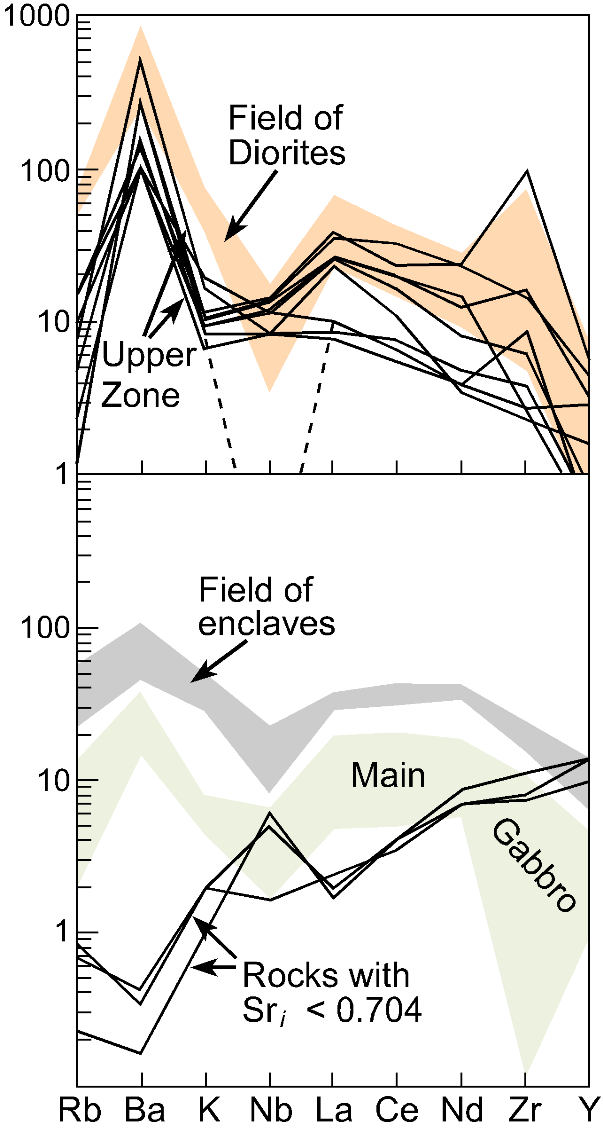
Abundances of incompatible elements in mafic and intermediate rocks of the Mafic Complex normalized to primitive mantle abundances (Hofmann, 1988).
Following incorporation of the septa by the Mafic Complex, advanced anatexis of residual biotite and especially K-feldspar, enriched in Ba and Eu, would provide the observed compositions. Ba and Eu are both compatible in K-feldspar, while only Eu is compatible only in plagioclase. In the Mafic Complex, the Ba an Eu anomalies are in general correlated positively, consistent with advanced melting of residual K-feldspar in the crustal source. A decoupling from this behavior may be caused by fractionation of plagioclase, with the result of increase Ba but decrease Eu up to cause a minor negative Eu anomaly, as observed in some norite from the paragneiss bearing belt (Fig. 15).
The highest Ba and Eu anomalies are found in cumulates above the paragneiss bearing belt, at the base of the upper Mafic Complex (Upper Zone of Rivalenti et al., 1984), an in the “Diorites,” while they are minor in the Main gabbro and in the mafic enclaves intruded in the “Diorites” (Figs. 13 and 15). This suggests that the “Diorites” are not differentiated melts (they should be lower in Eu, after fractionation of plagioclase-rich cumulates) but rather cumulates, as suggested also by the petrography (Fig. 16). If so, the “magma chamber” at the core of the arcuate structure was actually a cumulitic crystal mush within which swarms of mafic enclaves intruded as amoeboidal bodies.
Figure 16. “Diorites”
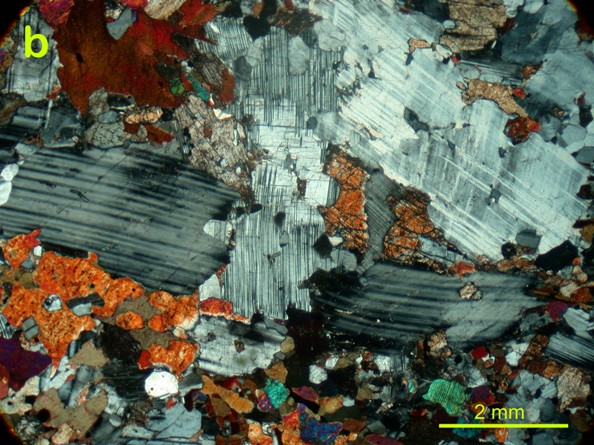
Although recrystallized, the texture of “Diorites” suggests they are cumulates of predominant plagioclase.