3. Description of the deformation and fabric gradient
Spatially oriented samples were collected at closely-spaced intervals along a transect, starting at the contact between the Cerro de Costilla tonalite and the orthogneiss wall-rocks, and progressing 28.3 m into the tonalite until a point was reached where the rock showed no field evidence of deformation (Fig. 2b). Sample collection was most closely spaced at the edge of the tonalite, where the strongest fabric gradient and mineralogical changes were noted. Two foliation-perpendicular thin sections were prepared from each sample: one parallel to and the other perpendicular to the lineation. Below we describe the progression from the least to most deformed rocks.
Figure 2. Bedding and foliation data in the southern portion of the Cerro de Costilla complex.
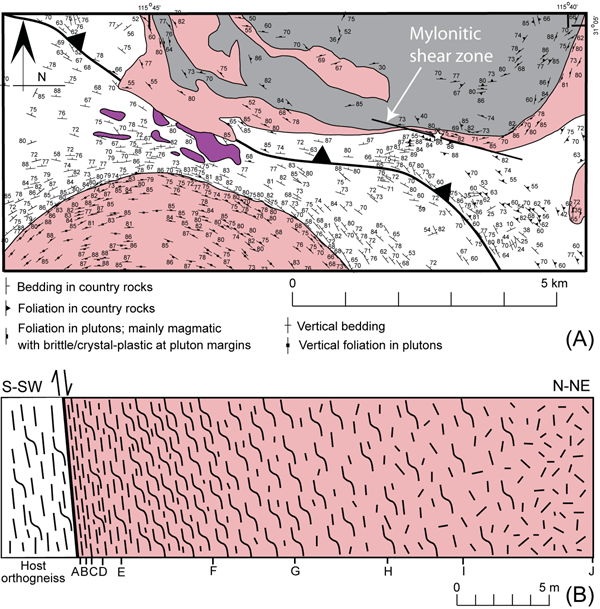
(A) Map showing bedding and foliation data in the southern portion of the Cerro de Costilla complex and surrounding area. See Fig. 1 for geology legend. Data from Johnson et al. (2004) and Melis (2006). The mylonitic shear zone examined here is shown at the southern edge of the complex, where it deformed the edge of the outer tonalite ring. Star marks the location where samples were collected. (B) Sketch of 28.3-m transect across the fabric gradient, from the contact with the host orthogneiss to slightly deformed tonalite. Letters A-J correspond to locations of oriented samples referred to in text.
3.1. Least deformed tonalite
The least deformed tonalite observed shows a weak magmatic foliation delineated mainly by biotite aggregates and aligned plagioclase grains. The plagioclase commonly has oscillatory zoning contained within core-to-rim compositional zoning, as discussed in Section 4. It also has well developed growth twinning and a few crystal faces, though most boundaries are irregular. Commonly it contains a few rounded quartz inclusions, as well as local rounded to euhedral and irregularly shaped inclusions of hornblende. Some plagioclase grains have been bent, producing local concentrations of lenticular deformation twins. Local evidence of minor strain-induced grain boundary migration is observed (Fig. 3). Interstitial quartz shows subgrains parallel to the c-axis, but has no chess-board subgrain patterns indicative of c-slip. It shows evidence of subgrain rotation recrystallization, as well as some grain boundary migration (Fig. 4). Brown-green hornblende occurs as irregularly shaped to subhedral grains and aggregates, some with inclusions of quartz and biotite, together with inclusions of plagioclase that vary from rounded to euhedral with rounded corners. Some of the hornblende has simple or multiple twinning, and some is optically zoned, with brown-green cores and blue-green rims.
Figure 3. Minor grain boundary migration in plagioclase.
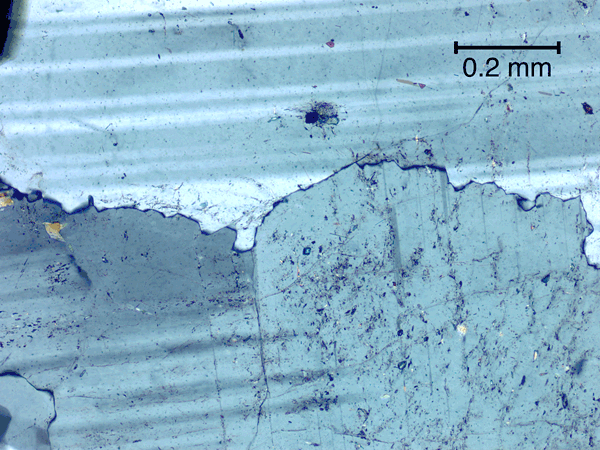
Evidence for minor strain-induced migration of grain boundaries in plagioclase. Crossed polars.
Figure 4. Typical quartz microstructures.
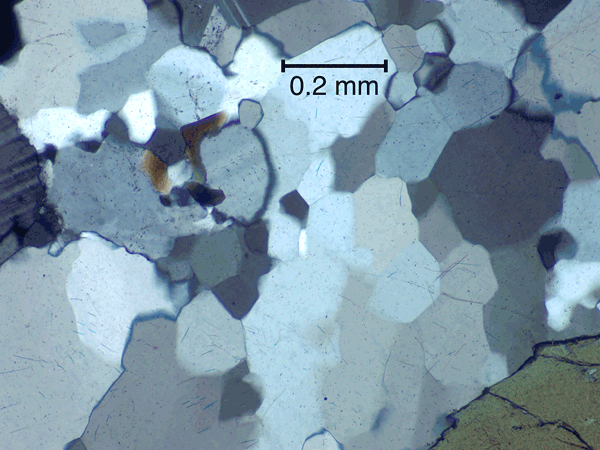
Quartz microstructures typically show evidence for a combination of subgrain rotation recrystallization and minor grain boundary migration. Variably developed foam texture and equilibrium grain boundary geometries indicate post deformational recrystallization. Crossed polars.
Biotite occurs as greenish brown, subhedral grains with {001} faces, as well as ‘decussate’ aggregates, a few of which locally pass into incipient foliae (Section 4), suggesting that some deformation and recrystallization occurred in the least deformed rocks. Locally biotite is intergrown with hornblende. Some biotite grains are bent, some have slightly misoriented, irregular to ragged ‘subgrains’ that appear to have been formed by fracturing (Fig. 5), and rare grains have been broken into fragments with ragged edges, the resulting spaces having been filled with quartz, fine-grained titanite and small rotated biotite fragments (Fig. 6). Locally biotite adjacent to plagioclase has been partly replaced by fine-grained, symplectic aggregates of titanite and plagioclase, the plagioclase being commonly in optical continuity with the plagioclase grain on which it nucleated (Fig. 7).
Figure 5. Biotite aggregate in the least deformed tonalite with granular titanite grains.
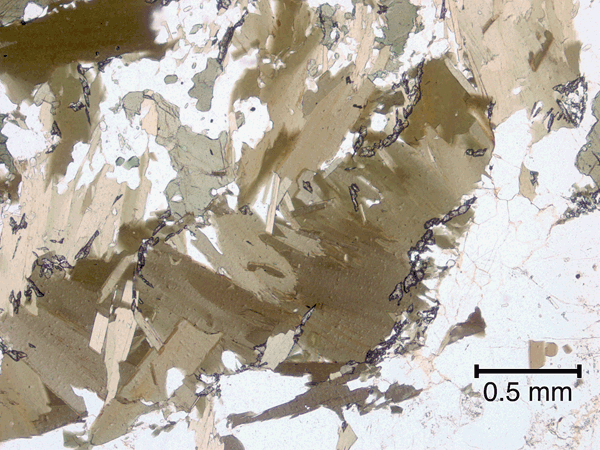
Biotite aggregate in the least deformed tonalite. The splintered grain contains irregular to ragged ‘subgrains’ with granular titanite concentrated around its edges. Plane-polarized light.
Figure 6. Bent, recrystallized biotite grain.
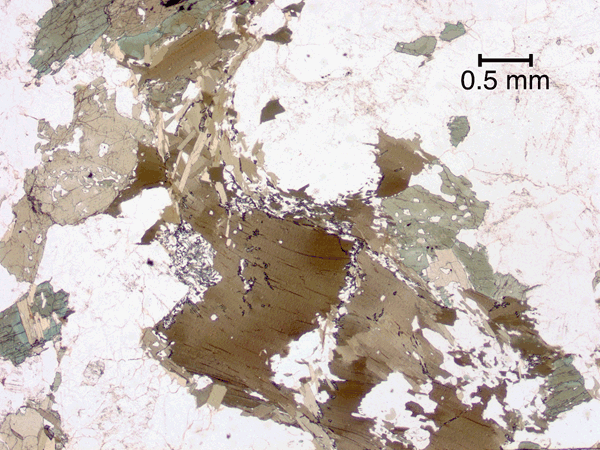
A large biotite grain that has been broken, separated (bottom-center) and bent into a folium and recrystallized (upper-center). The broken edges are ragged, and spaces between adjacent pieces of the original grain have been filled by quartz, fine-grained titanite and small biotite grains. Plane-polarized light.
Figure 7. Biotite partially replaced by symplectic plagioclase and titanite.
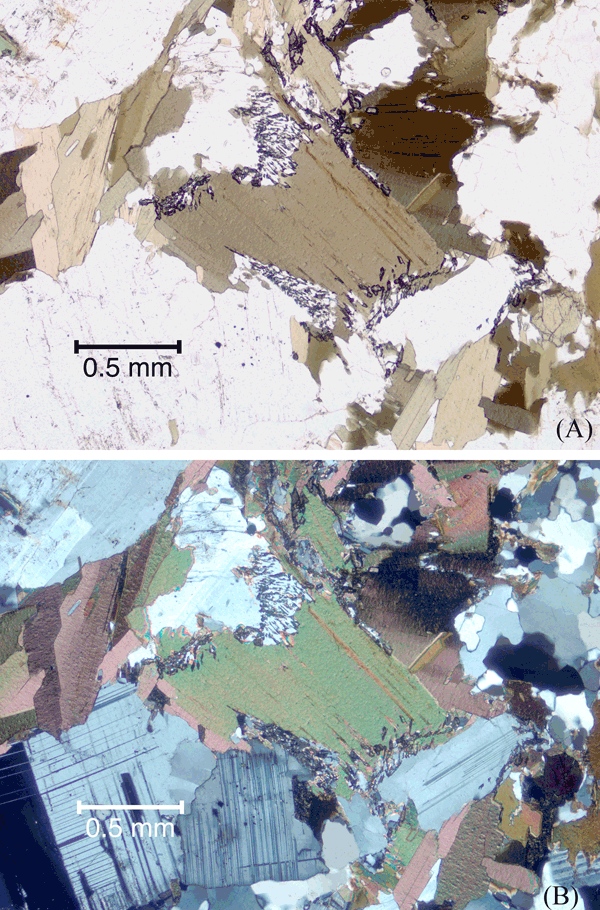
Lobes of symplectic plagioclase and titanite replacing the central biotite. (A) plane-polarized light; (B) crossed polars.
K-feldspar occurs as small interstitial grains marginally replaced by fine-grained myrmekite (Fig. 8), the formation of myrmekite probably being related to the deformation (e.g., Simpson, 1985; Simpson and Wintsch, 1989; Vernon, 1991).
Figure 8. Lobes of fine-grained myrmekite (x) replacing K-feldspar.
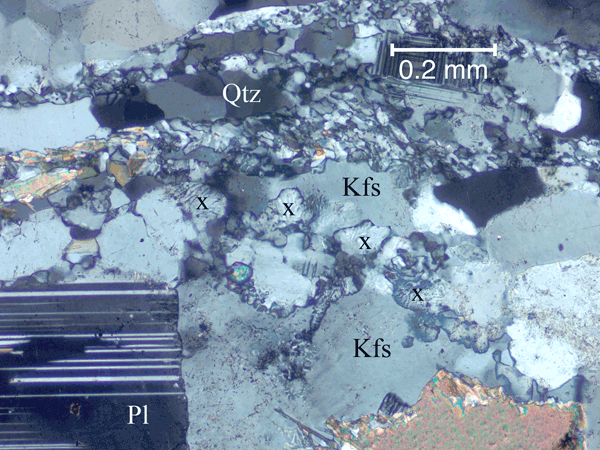
Interstitial K-feldspar (Kfs) extensively replaced by lobes of fine-grained myrmekite (x), which locally has recrystallized to form fine-grained, granular plagioclase-quartz aggregates initiating new foliae (upper half of image). Crossed polars.
Titanite occurs locally as scattered large grains, but mainly as small grains, aligned aggregates and veinlets, especially in biotite. Also present are rare pink to blue-black tourmaline, apatite and brown allanite.
3.2. Initiation of foliae
Quartz recrystallization increases with increasing fabric intensity, though the recrystallized grainsize remains relatively large. The recrystallized aggregates become elongated in more deformed rocks, but are highly elongated only in the most strongly deformed rocks, where they help to delineate the foliation. Quartz also forms incipient foliae in aggregates with biotite (Fig. 9), with or without fine-grained titanite or apatite.
Figure 9. Biotite, plagioclase and quartz forming incipient folium between grains of plagioclase.
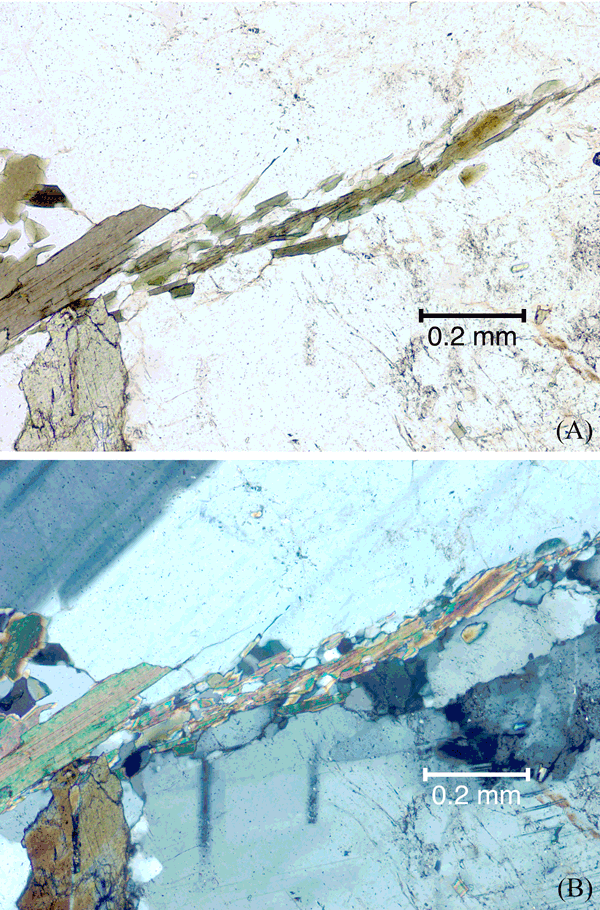
Incipient folium of biotite, plagioclase and quartz between grains of plagioclase that appear to have been either fragmented, recrystallized or both at the lower edge of the folium, which itself appears to have undergone recrystallization to form low-energy grain shapes. Some biotite grains appear to have penetrated along small fractures in plagioclase (centre of image). (A) plane-polarized light; (B) crossed polars.
Plagioclase locally shows small new marginal grains and aggregates of grains that, on the basis of optical evidence, could have been formed by either primary recrystallization or ‘sintering’ of small fragments. Though apparent subgrains occur locally, deformation temperatures were probably too low for subgrain rotation recrystallization to have played an important role (see sections 3.5 and 4.3). Therefore, local minute subgrains and new grains along internal fractures and grain boundaries (Fig. 10) probably originated mainly as fragments (e.g., Vernon et al., 2004; Johnson et al., 2004), although some genuine subgrains may be present (e.g., Fig. 10a). These fine-grained aggregates may contribute to foliae by providing pathways for linking biotite-rich foliae. Locally plagioclase grains have been fractured and pulled apart, some with fillings of undeformed quartz, others with fillings of recrystallized quartz, others with fillings of quartz and aligned biotite in a crude ‘beard’ structure, and still others with hornblende and plagioclase fragments that broke away from matrix grains as they flowed in to fill the gap (Fig. 11). No fillings involving feldspar were observed, confirming that melt was absent during the deformation (cf. Vernon et al., 2004).
Figure 10. Subgrains and new grains along grain boundaries and fractures in primary plagioclase.
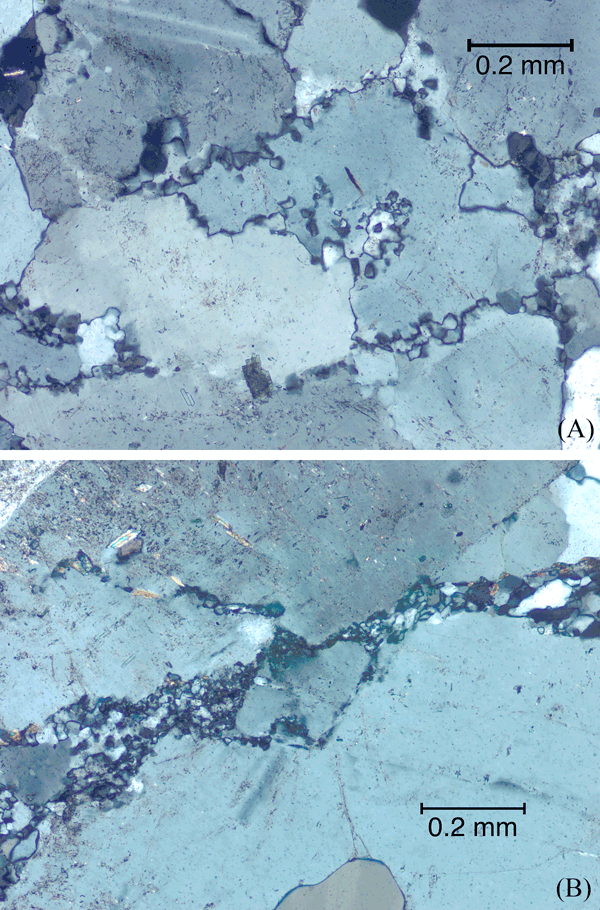
(A) Subgrains and new grains along grain boundaries and fractures in plagioclase. The subgrains and new grains in this example may possibly have formed by subgrain rotation recrystallization, or by fragmentation following by sintering. Crossed polars. (B) Very fine-grained aggregates along fractures in plagioclase, presumably formed by fragmentation. Crossed polars.
Figure 11. A single plagioclase grain broken into three pieces.
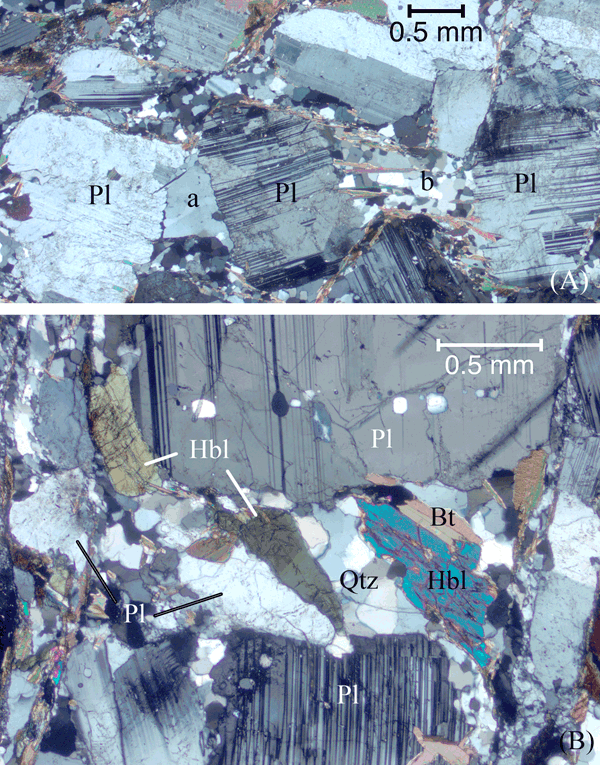
(A) A single plagioclase grain (Pl) broken into three pieces. The left gap contains a pool of only slightly deformed quartz (a) and the right gap contains a ‘beard’ of recrystallized quartz and aligned biotite. Crossed polars. (B) A plagioclase grain that has been pulled apart, the gap filled with recrystallized quartz, and fragments of hornblende and plagioclase. Lines indicate the matrix grains from which a hornblende (Hbl) and plagioclase (Pl) fragment originated. Crossed polars.
Biotite is the main contributor to foliation initiation, in the form of (1) small biotite grains that grow into fractures on the edges of primary plagioclase grains (Fig. 12a), and are eventually smeared out into long foliae as they separate the plagioclase into pieces (Fig. 12b); (2) aligned biotite aggregates cutting through hornblende grains, some following straight fractures, and others apparently partly replacing hornblende; (3) lenticular biotite grains and aggregates splintering during ‘necking down’ at their ends to form incipient foliae, owing to sliding of cleavage fragments on one another, followed in some instances by apparent recrystallization (Fig. 13); (4) development of sub-parallel splinters at bends in biotite grains; (5) deformed biotite grains bending into an alignment, accompanied by the development of subgrains and recrystallized aggregates (Fig. 14) (6) biotite-rich foliae leading away from hornblende that was partly replaced by biotite, the foliae commonly carrying small fragments of hornblende (Fig. 15), (7) thin veinlets of very fine-grained biotite and quartz, locally with titanite, epidote or apatite, mostly in primary grain boundaries, though locally cutting through grains, suggesting initiation by fracturing (Fig. 16), (8) ‘beards’ of biotite and biotite ± quartz on primary plagioclase grains (Figs 11a, 17), and (9) rare biotite splays or fans cut by foliae, to which they contribute (Fig. 18).
Figure 12. Biotite and quartz growing filling fractures in a primary plagioclase grain.
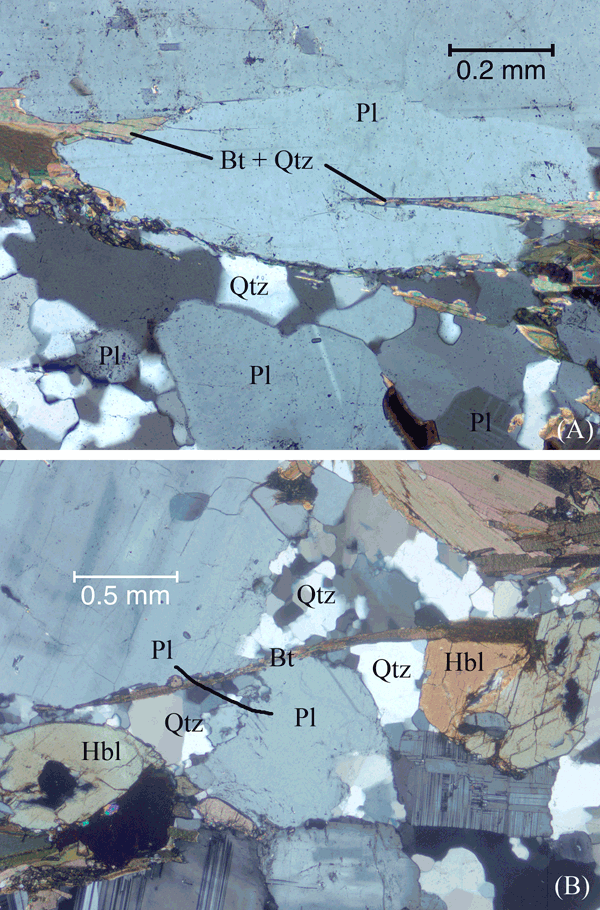
(A) Biotite and quartz growing into fractures on the lower edge of a primary plagioclase grain. Eventually, the lower part of the grain would calve off into the matrix. Fragments of plagioclase (Pl) in the matrix below may also have formed by this process. Crossed polars. (B) An example of what we infer to be a more advanced stage of the process in (A). A plagioclase fragment (lower-center) has been separated from its parent grain. A line shows the plagioclase grains that were once together. Pools of recrystallized quart fill spaces that have developed during the relative motion of strong plagioclase and hornblende (Hbl) grains. Crossed polars.
Figure 13. Thinning and internal deformation of a biotite grain to form a new folium.
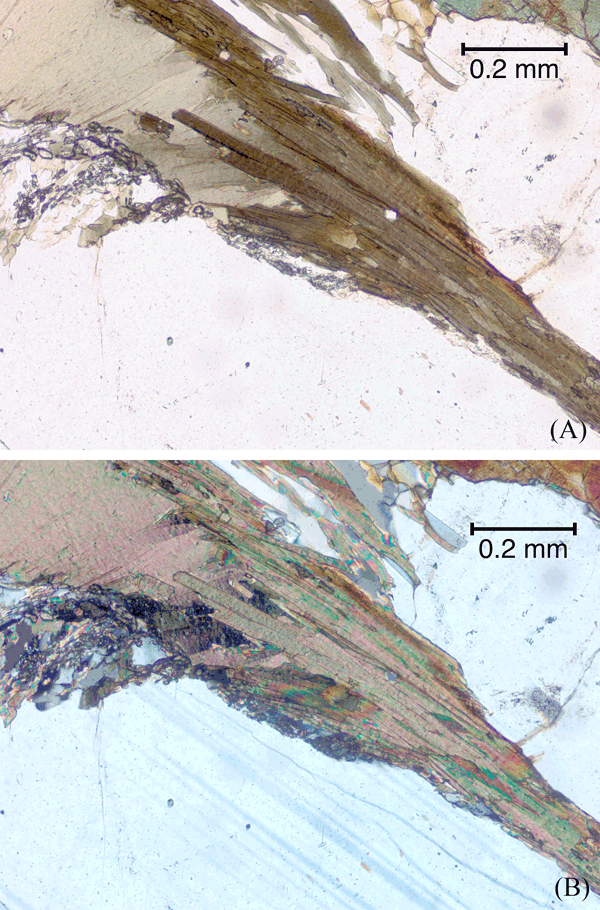
Internally deformed and thinned biotite grain forming an incipient folium between plagioclase grains. (A) Plane-polarized light, (B) Crossed polars.
Figure 14. Biotite grain bent, deformed and recrystallized into a developing foliation.
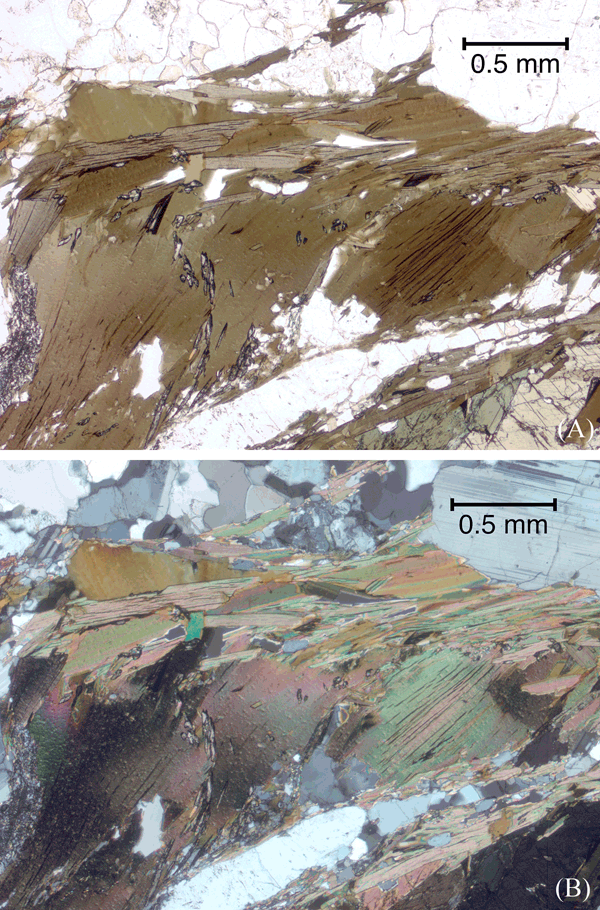
Biotite grain that had been bent towards alignment with a developing foliae, resulting in the development of fractures, subgrains and recrystallized aggregates. (A) Plane-polarized light, (B) Crossed polars.
Figure 15. Hornblende partially replaced by biotite and fragmented into a folium.
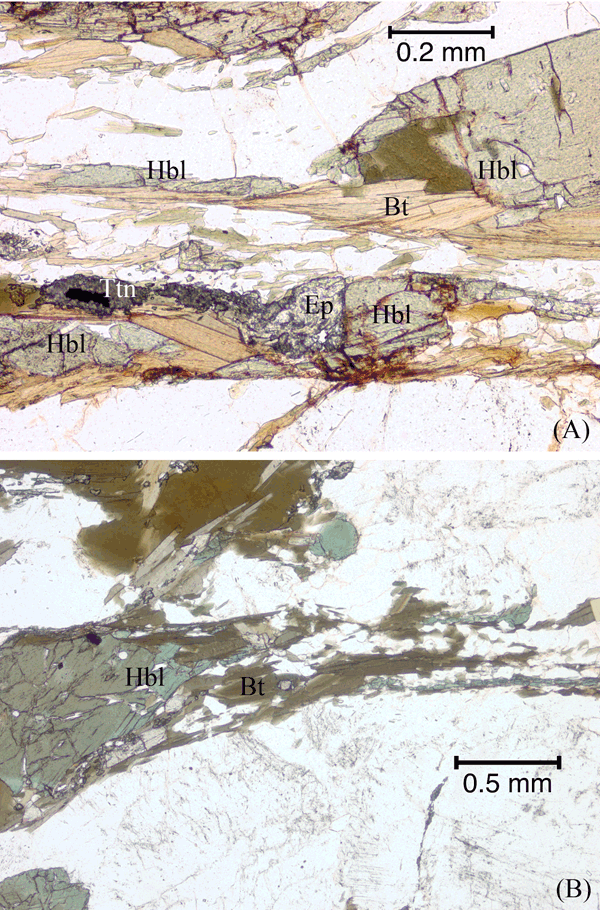
Two examples of extensive replacement of hornblende (Hbl) by biotite (Bt) and developing biotite-quartz folium with small hornblende fragments. (A) and (B) plane-polarized light.
Figure 16. Continuous foliae of biotite and quartz with small fragments of titanite, epidote and hornblende.
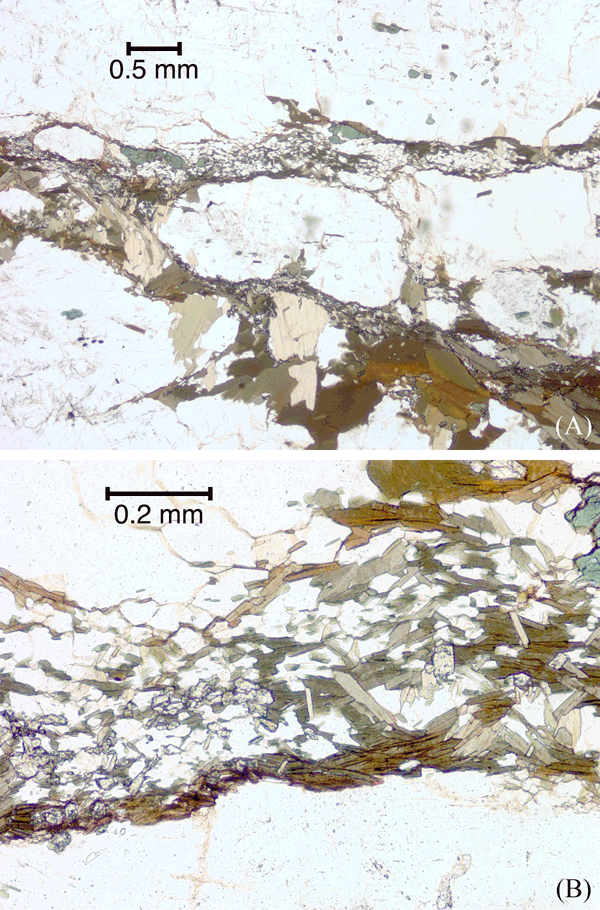
(A) Continuous foliae of biotite and quartz (both mainly recrystallized to form low-energy grain shapes), with small fragments of titanite, epidote and hornblende, anastomosing around large plagioclase grains. Plane-polarized light. (B) Magnified view of part of A, to show quartz and biotite grain shapes. The higher-relief grains are fragments of titanite and epidote. Plane-polarized light.
Figure 17. Biotite and quartz ‘beards’ on plagioclase
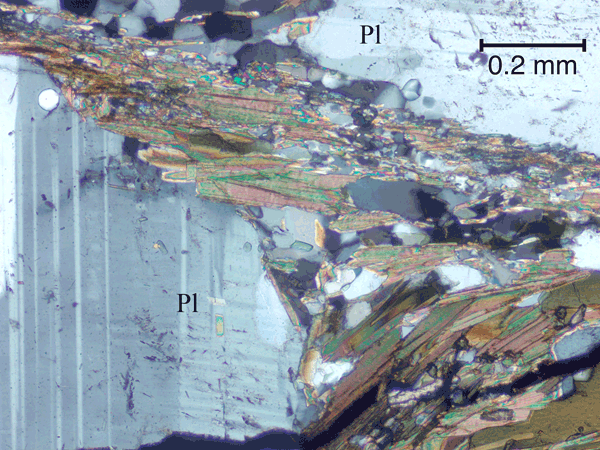
Biotite and quartz ‘beards’ on plagioclase lead away into foliae, the formation of the ‘beards’ involving partial replacement of the plagioclase. Crossed polars.
Figure 18. Biotite grain cut through by a foliae to which it contributes by recrystallizing into aligned new grains.
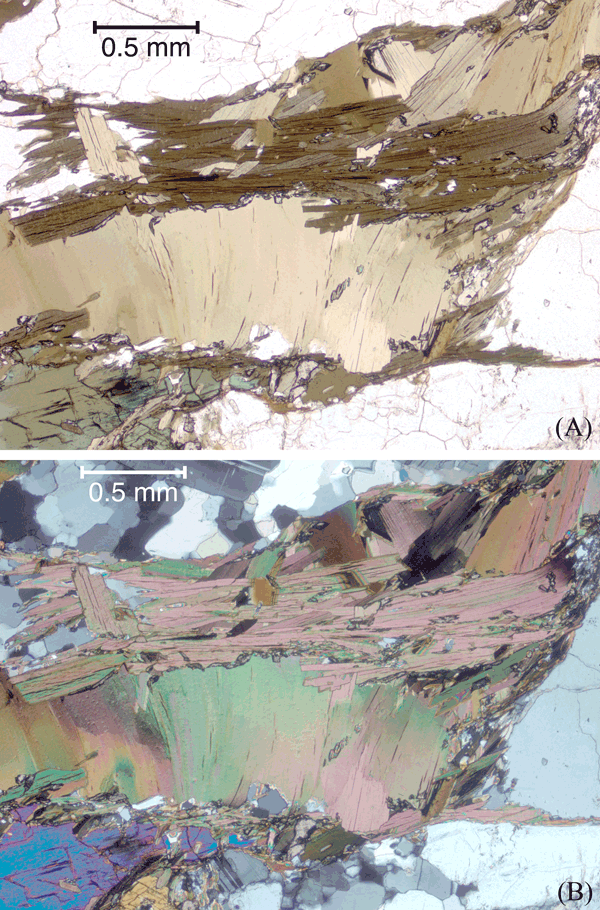
A fan-shaped grain of biotite, cut through by a foliae to which it contributes by recrystallizing into aligned new grains. Grain at lower left is hornblende. (A) plane-polarized light; (B) crossed polars.
Many of the new biotite-rich foliae follow grain boundaries of strong minerals, especially plagioclase and hornblende, constituting the beginnings of an S/C-type foliation pattern (Fig. 19). This is consistent with preferential fracturing along grain boundaries, rather than across grains, though some biotite-rich foliae, apparently occupying former fracture sites, do cut plagioclase and hornblende grains.
Figure 19. Development of an S/C-type fabric around plagioclase grains.
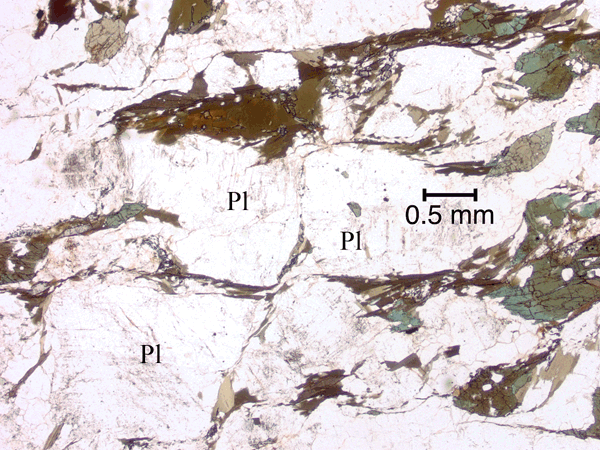
Biotite-rich foliae deflect around plagioclase grains in the beginnings of an S/C-type fabric. Plane-polarized light.
Hornblende shows rare internal deformation in the form of slightly misoriented ‘subgrains’ that probably formed by fracturing. In moderately deformed rocks, ‘beards’ of aligned blue-green hornblende (optically similar to the rims of zoned primary grains), with biotite and quartz, locally develop between drawn apart hornblende fragments (Fig. 20). Where hornblende is extensively replaced by biotite, the resulting hornblende relics can be incorporated into biotite-rich foliae (Fig. 15). Hornblende splinters have locally been smeared, with biotite, along some foliae. Uncommonly, hornblende grains neck down at their ends, releasing small fragments into incipient foliae (Fig. 21). In more strongly deformed rocks, some hornblende grains have been broken into cleavage fragments that have been separated and drawn out into crude foliae, linked by biotite and embedded in recrystallized quartz.
Figure 20. Blue-green hornblende, biotite and quartz form a ‘beard’ between pulled apart fragments of green-brown hornblende.
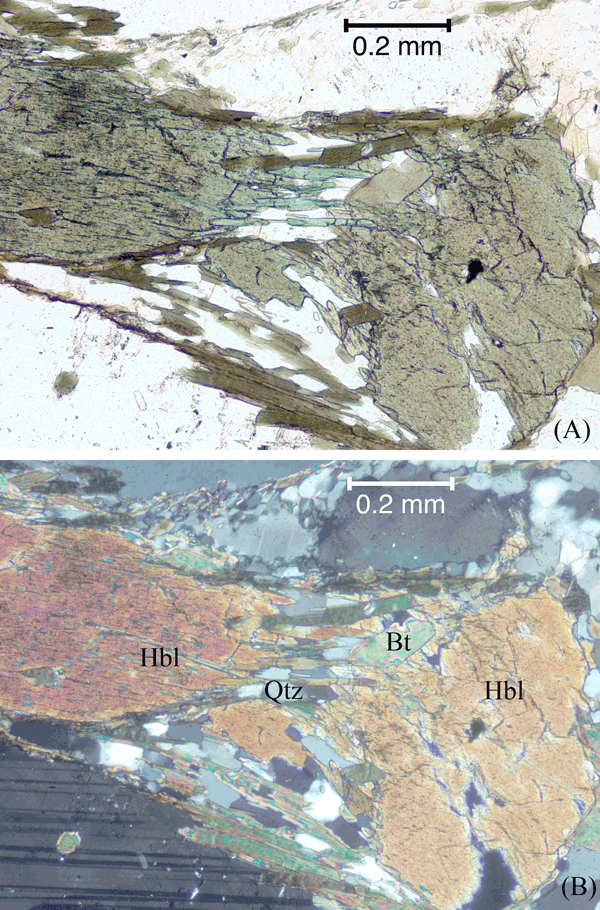
‘Beard’ of elongate blue-green hornblende, with biotite (Bt) and quartz (Qtz), between pulled apart fragments of green-brown hornblende (Hbl). The relatively low-energy grain shapes of quartz and biotite in the ‘beard’ suggest some recrystallization. (A) plane-polarized light; (B) crossed polars.
Figure 21. Hornblende grain necked down, fragmented and partly replaced by biotite.
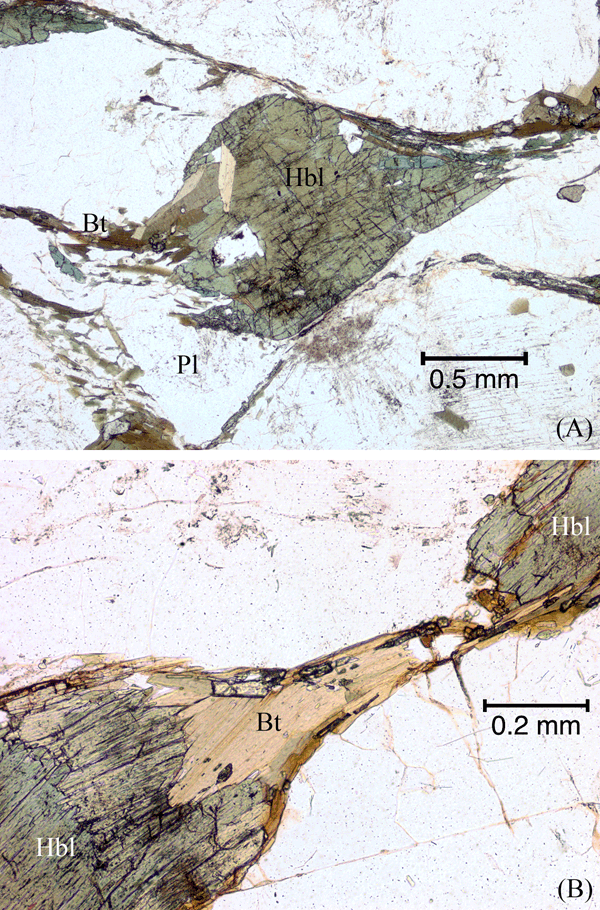
(A) Hornblende (Hbl) grain necking down and partly replaced by biotite (Bt), releasing small splintery hornblende fragments into the developing foliation. Also shown is a biotite-quartz ‘beard’ on a plagioclase (Pl) grain at bottom-left of image. Plane-polarized light. (B) Hornblende (Hbl) grain that has been boudinaged and pulled apart, and partly replaced by biotite (Bt) that crystallized in the boudin neck. Small fragments of hornblende are incorporated in the biotite. Plane-polarized light.
Titanite makes a minor contribution to the initiation of foliae, in the form of small lozenges and irregular grains in aligned biotite grain boundaries and rare foliae consisting mainly of fragments dislodged from originally large primary titanite grains (Fig. 22). Titanite-plagioclase symplectites replacing biotite (Fig. 7) form a fine-grained reaction product that may help to initiate foliation development, forming recrystallized, fine-grained aggregates (Fig. 23).
Figure 22. Foliae formed mainly of titanite fragments with some quartz and minor biotite.
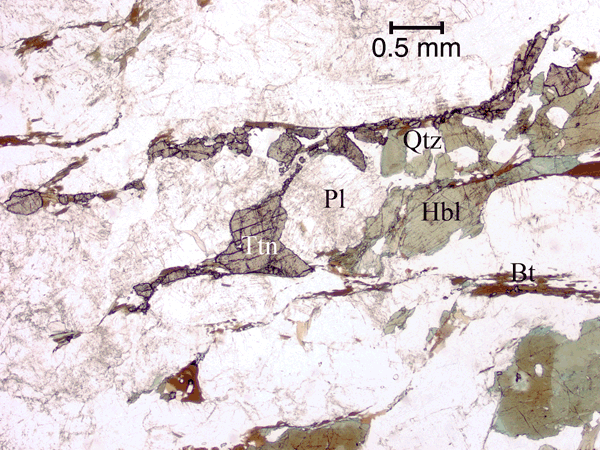
Foliae formed mainly of fragments of titanite (Ttn) with some quartz (Qtz) and minor biotite. Also shown are fragments of hornblende (Hbl) separated by coarse-grained quartz. Plane-polarized light.
Figure 23. Biotite cut by a folium containing titanite and disaggregated plagioclase-titanite symplectite.
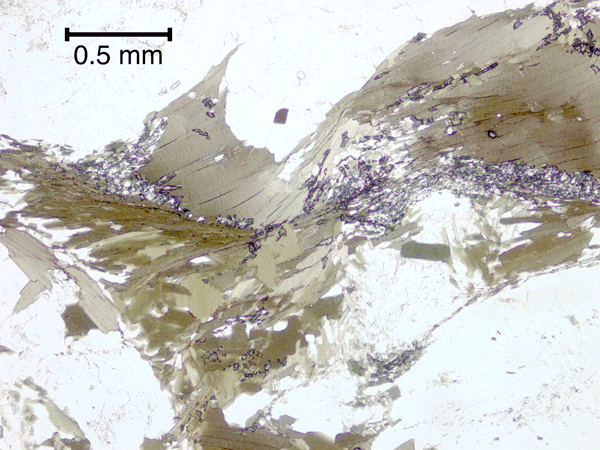
Folium consisting mainly of fine-grained, granular titanite and disaggregated plagioclase-titanite symplectite cutting deformed biotite. Plane-polarized light.
Myrmekite partly replacing the minor amount of interstitial K-feldspar (Fig. 8) can also contribute to the initiation of foliae, because the fine-grained quartz and plagioclase recrystallize to very fine-grained aggregates (Fig. 8) that promote grain-boundary deformation processes (e.g., Vernon et al., 1983; LaTour, 1987).
3.3. More continuous foliae
Many relatively continuous foliae consist of recrystallized biotite ± quartz, with or without irregularly distributed titanite, plagioclase, epidote and hornblende (Fig. 16). In some foliae the biotite is locally ‘decussate’ (Fig. 16), whereas in others it is well aligned (Fig. 15a). Some biotite-rich foliae splay into several foliae. Most foliae pass between primary grains and so tend to anastomose around large, strong grains of plagioclase and hornblende in an S/C-type relationship (Fig. 24).
Figure 24. Anastomosing foliae forming an S/C-type fabric around plagioclase grains.
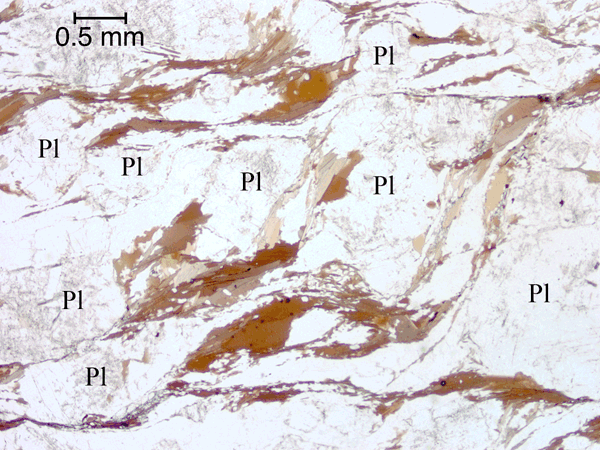
Biotite and biotite-quartz foliae anastomosing around plagioclase grains in an S/C-type relationship (C being parallel to the long edge of the image) in a strongly deformed rock (Sample C). Plane-polarized light.
Initially, the biotite-rich foliae are discontinuous, with insufficient linkages to make a through-going foliation (e.g., Johnson et al., 2004). Links or bridges consist mainly of fine-grained aggregates, with varying proportions of biotite, quartz, titanite and plagioclase (Fig. 25). Some are very fine-grained plagioclase-quartz aggregates representing recrystallized myrmekite (Fig. 8), whereas others appear to be small plagioclase grains, many of which may have originated as minute fragments along fractures (Fig. 10). After the links have been established, further strain can accumulate by preferential deformation of continuous, weak, biotite-rich foliae, producing mylonite, as discussed by Johnson et al. (2004).
Figure 25. Fine-grained biotite, plagioclase, quartz and titanite folium linking biotite foliae.
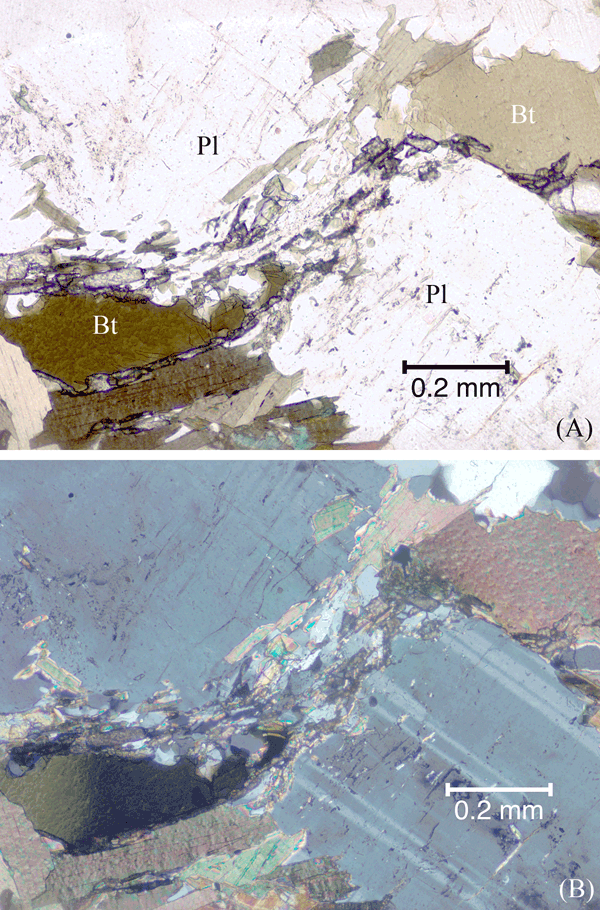
Linking (bridging) folium between biotite foliae, consisting of fine-grained biotite, plagioclase, quartz and titanite. (A) plane-polarized light, (B) crossed polars.
3.4. Strongly deformed rocks
Foliae in the most strongly deformed rocks consist of: (1) continuous aggregates rich in biotite, with varying proportions of quartz, minor ilmenite and rutile, and local fine-grained aggregates derived from the symplectites replacing biotite; (2) elongate aggregates of recrystallized quartz (Fig. 26); and (3) finer-grained aggregates rich in biotite and quartz forming ‘beards’ on and between plagioclase clasts, all anastomosing around the larger plagioclase grains (Fig. 27). Biotite and, less commonly, quartz appear to have replaced plagioclase at the edges of the ‘beards’ (Figs 17, 28), although in some instances these minerals may simply be growing into jagged embayments where fragments of the plagioclase have been detached. In many places, these detached plagioclase fragments of various sizes are incorporated into the ‘beards’ (Fig. 27). We infer that the internal foliation in the ‘beards’ delineates the stretching direction.
Figure 26. ‘Ribbons’ of recrystallized quartz
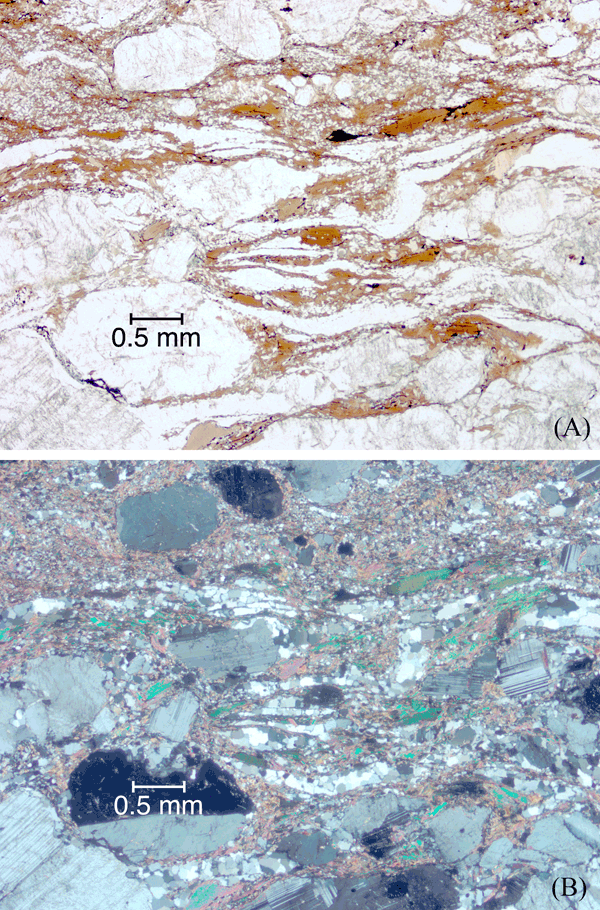
‘Ribbons’ of recrystallized quartz (representing former interstitial quartz grains) alternating with biotite foliae and biotite-quartz foliae in the most strongly deformed rock (Sample A). (A) plane-polarized light; (B) crossed polars.
Figure 27. Plagioclase clast with ‘beard’ of biotite and quartz.
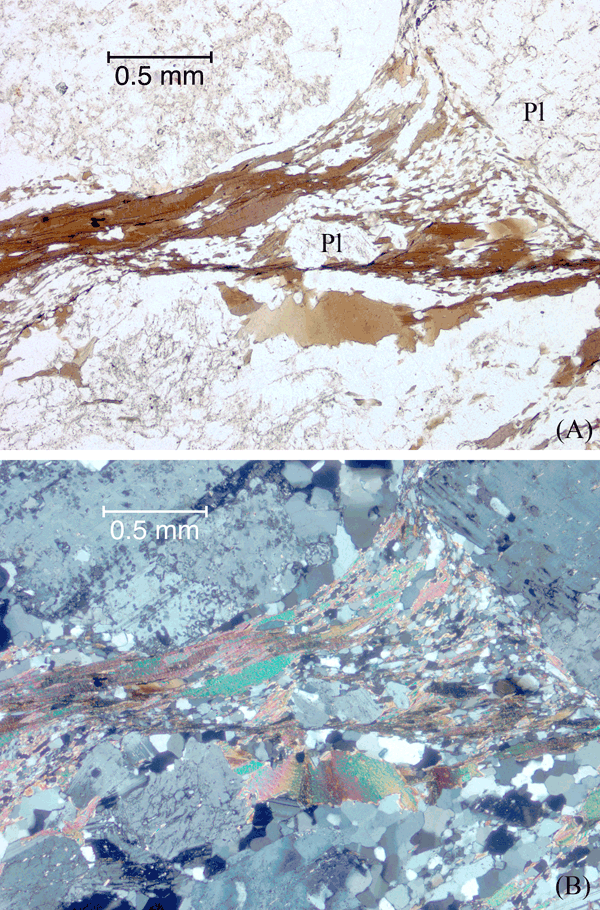
‘Beards’ of biotite and quartz at the end of a plagioclase clast in a strongly deformed rock (Sample C). (A) plane-polarized light; (B) crossed polars.
Figure 28. Plagioclase clast with ‘beard’ of biotite and quartz.
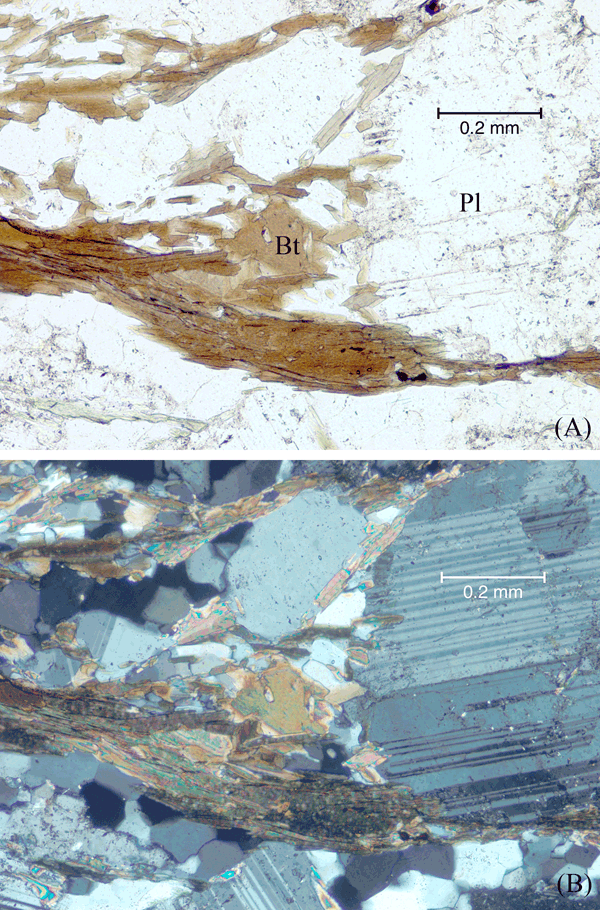
‘Beard’ of biotite and quartz at the end of a plagioclase clast in a strongly deformed rock (Sample C). The biotite, and possibly quartz, appear to have replaced the plagioclase. (A) plane-polarized light; (B) crossed polars.
As shortening normal to the foliation increases, the plagioclase clasts separate, with the result that the ‘beards’ become extended into fine-grained, foliated aggregates and continuous fine-grained foliae (Figs 27, 29), eventually constituting a foliated matrix between residual plagioclase clasts. At this stage of foliation development, the S/C-type character of the less deformed rocks is replaced locally by a more uniform, anastomosing foliation as the biotite and quartz foliae collapse into zones between separating plagioclase clasts. The plagioclase clasts are locally broken and can be extensively fragmented, the fragments being cemented by recrystallized quartz (Fig. 30).
Figure 29. Plagioclase clast with ‘beard’ of biotite and quartz extending into a folium.
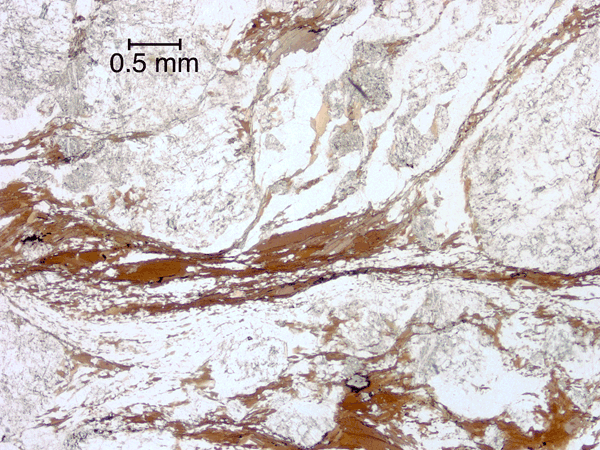
‘Beard’ extended into a folium in a strongly deformed rock (Sample C). Plane-polarized light.
Figure 30. Shattered plagioclase clast with infilling of quartz.
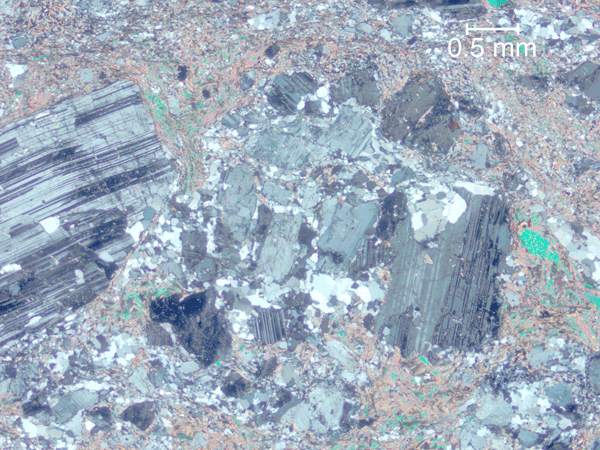
Plagioclase clast that has been shattered, the volumes between the fragments having been filled with quartz, now recrystallized, in the most strongly deformed rock (Sample A). Crossed polars.
In one layer (about 2 cm thick) of one of the more strongly deformed rocks, the plagioclase shows marginal replacement by lobes and irregular patches of symplectic, myrmekite-like aggregates (Fig. 31), some plagioclase grains having been extensively to completely replaced; locally the replacing plagioclase is devoid of quartz blebs (Fig. 31). The rocks show an abrupt change from layers without plagioclase replacement to the layer with extensive plagioclase replacement. We infer that this layer was preferentially infiltrated by fluid, in order to produce the symplectite (discussed below).
Figure 31. Plagioclase-quartz symplectite (x) replacing plagioclase.
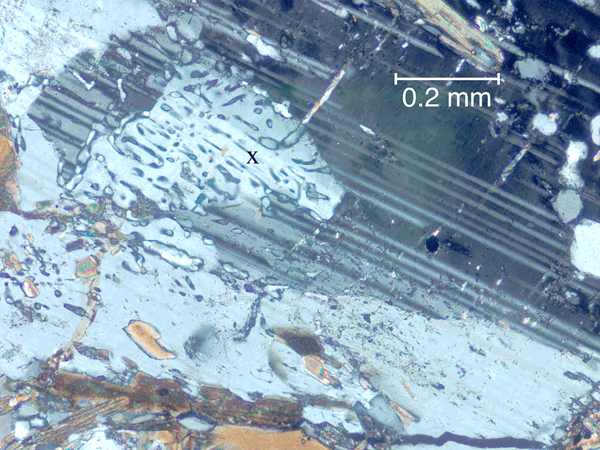
Lobe of plagioclase-quartz symplectite (x) replacing plagioclase in a layer in Sample C. See Fig. 37b for plagioclase compositional data. Crossed polars.
The most deformed rocks (sample A) contain quartz-tourmaline patches and veins, in which the tourmaline occurs as angular fragments dispersed in recrystallized quartz. Some of the tourmaline consists of close fragments that could be fitted back together, separated by single-crystal quartz fillings, suggesting deposition of quartz from solution.
In the mylonitic rock immediately at the edge of the pluton (sample A), the grainsize of the biotite-quartz aggregates is much smaller than in samples B and C, and the amount of matrix between the plagioclase clasts appears to have increased (Fig. 32). Extensive recrystallization of the matrix produced fine-grained decussate aggregates of biotite and aggregates of quartz and biotite with low-energy grain shapes.
Figure 32. Fine-grained, biotite-rich matrix in the most strongly deformed rock.
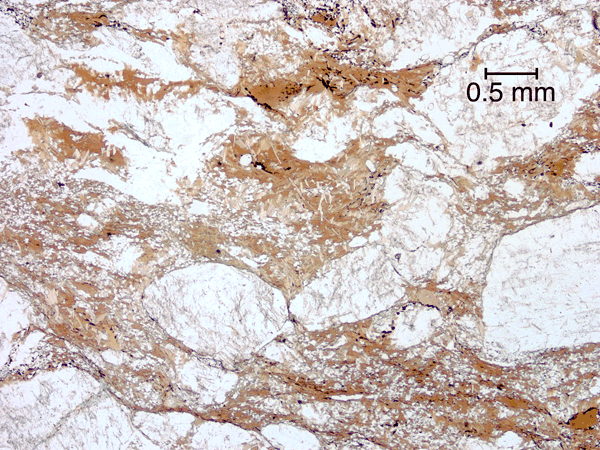
General view of the fine-grained, biotite-rich matrix in the most strongly deformed rock (Sample A). Plane-polarized light.
3.5. Microstructural evidence of deformation conditions
We infer from microstructural observations that the deformation temperatures for the Cerro de Costilla rocks were 400-550°C, because (1) quartz shows no evidence of c-slip, which is generally inferred to require temperatures above 600°C (e.g., Kruhl, 1996, 1998); (2) quartz shows widespread evidence of subgrain rotation recrystallization, and some evidence for grain-boundary migration recrystallization, suggesting a temperature range of 400-500°C (Hirth and Tullis, 1992; Tullis et al., 2000; Stipp et al., 2002a, b); and (3) plagioclase shows no clear evidence of dislocation creep or subgrain rotation recrystallization, which require temperatures above 500-550°C (Tullis et al., 2000; Rosenberg and Stünitz, 2003); instead it appears to have undergone minor grain-boundary migration and fracturing, though some recrystallization (‘sintering’) of fragmental aggregates may have occurred — a process that can occur at less than 400-500°C (Fitz Gerald and Stünitz, 1993). Given the local mobility of plagioclase grain boundaries, and that we cannot completely rule out very rare subgrain development through rotation recrystallization, we place an upper limit on temperature of 550°C. In Section 4.3 we present hornblende-plagioclase thermometry that suggests a deformation temperature less than approximately 540°C. In view of the microstructural evidence and thermometry results, we suggest that the deformation temperature was 475 ± 50°C.