Discussion
Evidence for generation in a Slow-spreading center
It is now defined that the physical configuration of the crust–mantle sequence is related to differences in the spreading rate of an oceanic basin, which, to a certain extent, is related to the amount of magma extruded on the surface (Searle, 1992; Niu and Hekinian, 1997; Klingelhofer et al. 2000; Graciano and Yumul, 2003). Slow-spreading centers, which would include the Mid-Atlantic Ridge, Southwest Indian Ridge, and the West Philippine Sea, are characterized by a spreading rate of less than 5 cm/year and fluctuating magma supply with mechanical extension resulting primarily from faulting. Fast-spreading centers, with 10 cm/year full spreading rates, are characterized by robust magmatism with sheet flows dominating over pillow lavas. Sediments intercalated within volcanic rocks indicate gaps in volcanism and are indicative of generation along intermediate spreading-rate centers (Karson, 1998).
Slow-spreading centers contain tectonic features that are not readily apparent in intermediate- to fast-spreading centers (Tucholke et al. 1998; Fujioka et al. 1999; Allerton et al. 2000, Graciano and Yumul, 2003 and Khalatbari-Jafari et al., 2004). Submersible dives and dredging data supported by geophysical information show that slow-spreading centers are characterized by (a) small volume of gabbros, (b) general absence of dike complex, (c) deep-water sediments or volcanic rocks directly overlying peridotites, (d) large-scale extensional faulting, (e) discontinuous axial magma chambers, (f) localized hydrothermal deposits, and (g) large-scale tilting of the crust (Cannat and Casey, 1995; Lagabrielle et al. 1998). As already mentioned, due to the paucity of magmatism and the low degree of partial melting, the crust has time to solidify and strengthen because the main cause of mechanical extension is faulting (Ishiwatari, 1985; Karson, 1998). Volcanism is also characterized by point source volcanism with the lava flows dominated by pillow structures (Searle, 1992). With the robust magmatism in fast-spreading centers, volcanism is fissure-type with the dominance of sheet flows with respect to pillow lavas. The typical magmatic activities of fast-spreading centers ensure the existence of long-lived magma chambers (MacDonald, 1998).
Comparing the SCO with the above geological attributes gives an idea of the responsibility of the kind of spreading center for the generation of this ancient sequence. The layered- and massive-gabbro suggest the presence of a local magma chamber (Figure 3). Slow-spreading centers have no well developed magma chamber and are dominated by gabbro pods within the peridotite indicating episodic intrusion or a low magma budget.
The SCO is intensely faulted - brought about by mechanical extension as is the case for slow-spreading centers. The presence of a sediment layer within the volcanic suite may indicate that a gap in volcanism was encountered. This supports the possibility of the SCO being formed in a slow-spreading center.
The paucity of well-developed layered-gabbro indicates that a magma chamber does not exist and supports the formation of the SCO in a slow-spreading center. Although the rate of spreading may change through time or even along the ridge axis (Lagabrielle and Lemoine, 1997), there is no compelling evidence to conclude that the SCO is formed in a fast-spreading environment.
The presence of widespread pillow lava with respect to sheet lavas in the studied area (ratio 90 to 10) indicates that the rate of the spreading center is slow. Based on the diagram of spreading rate versus pillow lava/sheet lava (Juteau and Maury, 1999), the rate calculated for this ophiolite is less than 5 cm/year.
In conclusion, based on the observed field characteristics of the SCO, it is believed that this ophiolite complex has characteristics of slow-spreading centers and was formed in a slow-spreading center.
Harzburgite ophiolite type or lherzolite ophiolite type?
The crust–mantle sequences from slow-spreading centers correspond to the lherzolite ophiolite type (LOT) while those from fast-spreading centers are characterized by the harzburgite ophiolite type (HOT) (Nicolas and Al Azri, 1991). As presented in the works of Boudier and Nicolas (1986), the HOT is characterized by (a) a metamorphic sole made up of metamorphosed oceanic crust, (b) a thick cumulate gabbro layer, (c) an intrusive complex dominated by dikes, (d) volcanic rocks having tholeiitic characteristics and the mantle section composed of harzburgite and dunite, and (e) the presence of chromite pods. The LOT is defined as having (a) a metamorphosed continental or oceanic crust as the metamorphic sole, (b) thin to poorly developed mafic cumulate layer, (c) the preponderance of sills over dikes, (d) the presence of tholeiitic to alkaline volcanic rocks with the mantle section characterized by plagioclase lherzolite, and (e) rare to absent chromite pods. Considering at these varying parameters, it can be readily seen that the HOT has undergone greater degrees of cumulative partial melting in comparison with the LOT (Ishiwatari, 1991). This is because of the dissimilarity between fast- and slow-spreading centers. Fast-spreading centers, characterized by robust magmatism, have mantle source regions that have undergone more stages and greater degrees of partial melting resulting in the formation of harzburgite as the residual peridotite (Niu and Hekinian, 1997). On the other hand, slow-spreading centers are characterized by episodic magmatism, which is exposed to lower degrees of partial melting comparing with fast-spreading centers (Ishiwatari, 1985). As a result, clinopyroxene-bearing harzburgite or lherzolite, in general, characterizes the mantle peridotite section of slow-spreading centers.
Based on the field characteristics, the SCO contains pillow lavas and rare sheet flows with a rare associated hypabyssal dike complex and a small mafic cumulate sequence. Furthermore, no pods of chromite are present. It seems that the appropriate condition for the formation of chromite mineralization (e.g. high PH2O; high fO2; extensive mantle–melt interaction) was not achieved. Unfortunately, the mantle parts of SCO are not outcropped and we could not find any lherzolite or harzburgite sample in the whole study area. Although there is no occurrence of lherzolite because of dense rain forest, the absence of orthopyroxene in the samples of ultramafic sequences can be evidence for non-formation of harzburgite in this region. Now, based on the absence of harzburgite or lherzolite the important question that arises is, "is the SCO sequence a lherzolite ophiolite or a harzburgite ophiolite?"
After opening of the older ocean (Paleo-Tethys(II) (Eftekharnejad et al., 1993) or Meso-Tethyse (Spakman, 1986 and Golonka, 2004)) in Northern Iran (Figure 5a), the Southern Caspian margin developed by seafloor spreading. Subduction began beneath the Central Iran Block, after the collision of this microcontinent with Eurasia (Figure 5b). This subduction produce Alborz arc volcanism during Cretaceous to Cenozoic (Figure 5c). The last oceanic lithosphere was produced during Upper Cretaceous in a closing oceanic basin and in a slow-spreading center (Figure 5d). Probably this oceanic lithosphere was formed above the arc volcanism zone and was possibly metasomatised by fluids coming from the subducted slab and may explain the observed island arc geochemical signature (Figure 5e). This oceanic lithosphere was never subducted and remained unmetamorphosed, creating the Upper Cretaceous ophiolite complex of the Southern Caspian Sea (Figure 5f).
Figure 5. Geodynamic evolution of the SCO.
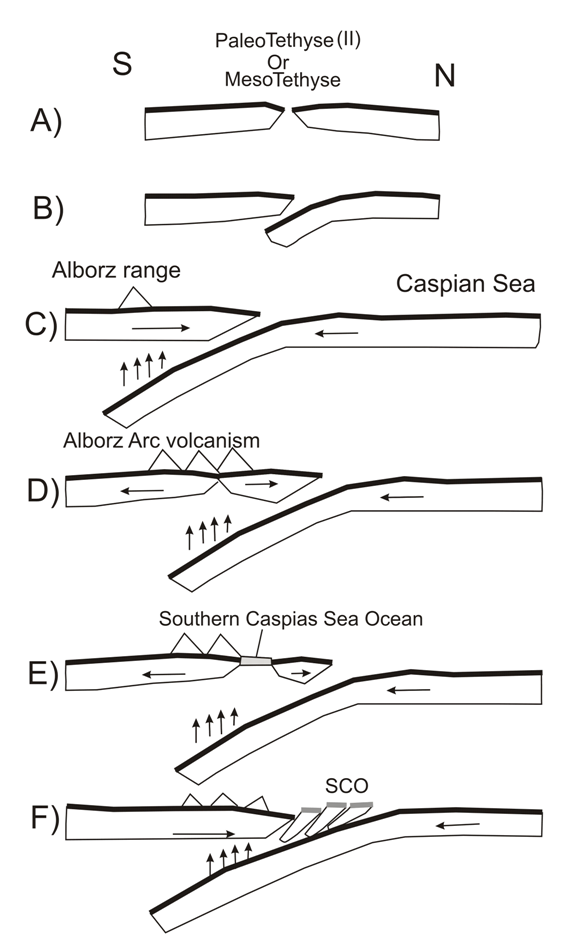
Proposed scenario for the geodynamic evolution of the Southern Caspian Sea Ophiolite. See text for discussion.
Lastly (Lower Paleocene), due to a change in the stress field from a compressional to a local extensional regime due to a local or regional tectonic event, the Southern margin of the basin began to be thrust beneath the Upper Cretaceous oceanic lithosphere. Very local extension in this time produced the alkali rocks that intruded in all parts of SCO ophiolite. Just before collision, the ophiolite of Southern Caspian Sea was obducted over the older rocks.