FAQ-5. What observations and data are relevant to the relationship between fine-grained pyrite and the economic ores?
Painter et al. (1999) report observations and extensive sulphur isotope data on the fine-grained pyrite from the northern end of the lead-zinc system and extending for more than 11km northwards, as well as from south of the mine. Some of these observations were regarded as supporting the interpretations of Perkins (1998) and some seemed to be inconsistent with them. Three of these aspects are discussed below.
Distribution of fine-grained pyrite
Differing interpretations exist on the extent to which concentrations of fine-grained pyrite are continuous along the same stratigraphy or transgress across it. The most detailed attempts to portray the distribution of fine-grained pyrite are by Perkins (1998) and Painter et al. (1999). The former focussed on the Mine area and the latter on the north end of the Mine and northwards. Both use a combination of their own observations and Mine records. Because of its fine grain size, (Figure 15) volumetric percentages of the earliest pyrite are notoriously difficult to estimate, with wide variation often showing between adjacent drill holes logged by different geologists. Added to this is the problem alluded to by Perkins et al 1998 (p. 1156) and Painter et al. (1999) of the difficulty of distinguishing between true fine-grained pyrite and "brassy pyrite" which is coarser-grained and far less continuous along bedding exposure. Within copper orebodies, there is the added complexity of allowing for the proportion of coarse-grained dolomite, quartz and chalcopyrite. Although relict bedding rich in fine-grained pyrite is common (Figure 16, Figure 17 and Figure 18) it may nonetheless constitute a minor percentage of the total rock volume.
Figure 15. Fine-grained pyrite relationships.
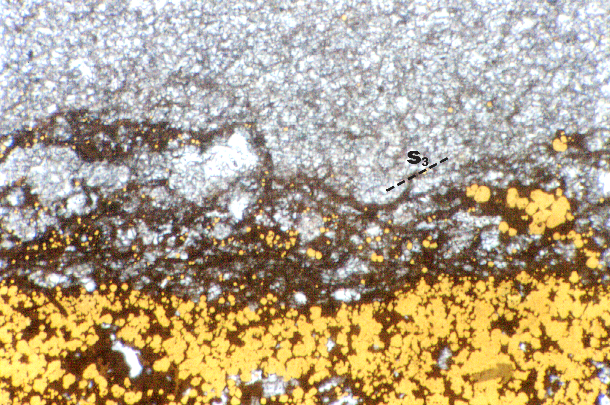
Fine-grained pyrite along bedding (horizontal) and seams which anastomose between bedding and cleavage S3. The grain size and variability of packing show how difficult it is to estimate pyrite percentage. Part transmitted/part reflected light. Field of view 0.6mm. 6 orebody 11 Level, 7172mN, 1652mE.
Figure 16. Fine-grained pyrite in the outer zone of silica-dolomite.
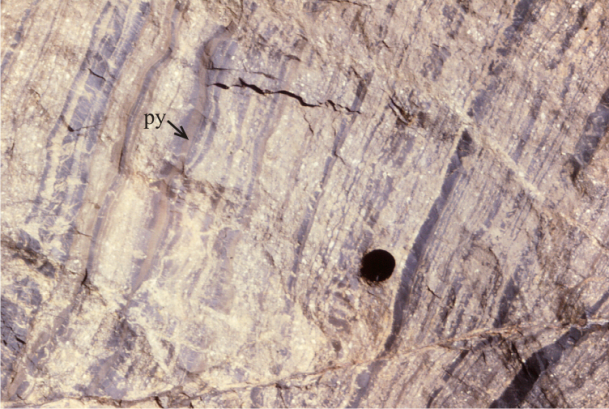
Sequence of dolomitized (whitish, banded) and silicified (dark grey) layers in the bedding-altered part of the silica-dolomite, showing layers rich in fine-grained pyrite (py, khaki). 400 orebody area 5/200 orebody stratigraphy 9 Level 5415mN. Looking north.
Figure 17. Fine-grained pyite in high grade silicified zone of silica-dolomite.
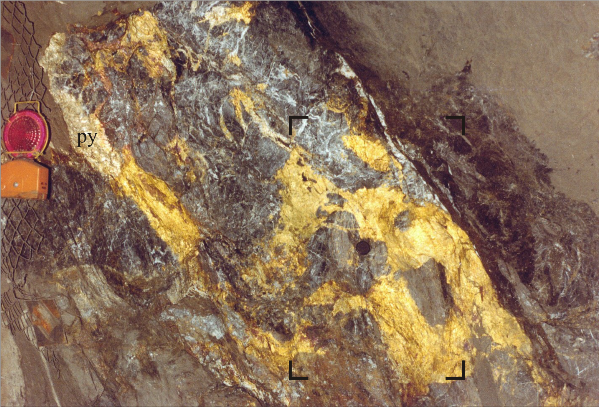
Chalcopyrite-rich breccia with relict blocks containing a network of quartz veins after dolomite and some blocks with a high percentage of fine-grained pyrite along relict bedding. Looking south-west. Apart from an area of coarse-grained pyrite (py) the remaining near-massive sulphide in the matrix is chalcopyrite. The area of Figure 18 is shown. 18B sublevel 4802mN 1780mE.
Figure 18. Close-up of Figure 17.
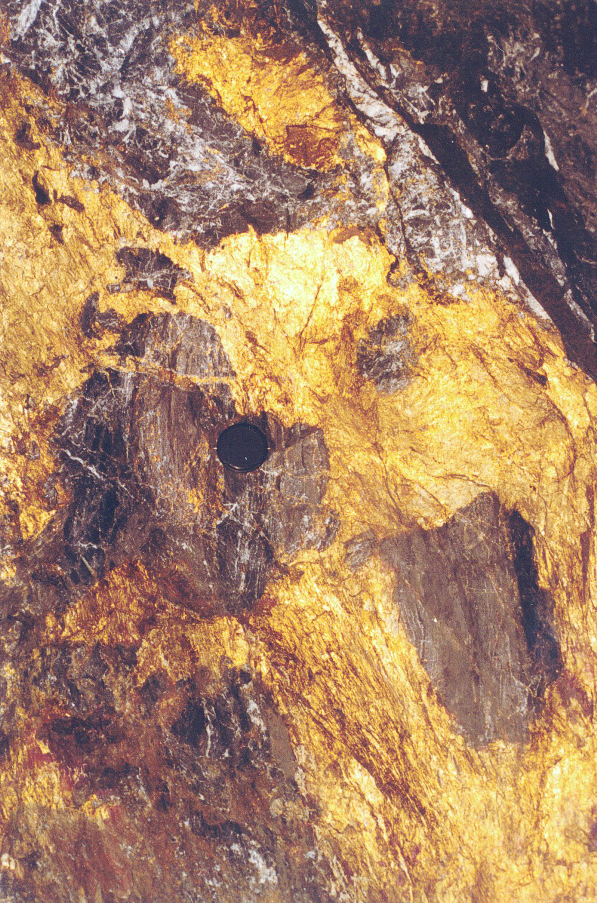
Brown near-vertical layers in the block beneath the lens cap and the adjacent block are rich (~60%) in fine-grained pyrite.
Both authors agree that there is considerable continuity of fine-grained pyrite along bedding (see especially Painter et al. figures 7 and 8). Perkins (1998, fig. 6b) shows that within a sequence ranging in thickness from 35mm to 400mm (B sequence) fine-grained pyrite only occurs over a strike length of 2500m. In addition, there is no change in microfacies where pyrite is not deposited (Perkins, 1998, fig. 7). Painter et al. (1999) maintain that "local peaks in concentration of fine-grained pyrite are also evenly distributed throughout the formation" p. 893. Observation of fig. 7 of Painter et al. does appear to show a diminution of fine-grained pyrite concentration along strike from the Mine (K754) to QZ10. There is no evidence in their data to reflect the gross transgression of concentration across stratigraphy illustrated by Perkins (1998, fig. 5b), which, despite the difficulties of estimation mentioned above, is so marked. There is not sufficient "brassy pyrite" for the Mine pyrite transgression to be explained by this overprint alone, as suggested by Painter et al. p. 909. In fact, to the north of Mine the 8 orebody sequence in QZ10 contains significant pyrite while the overlying 7 orebody sequence is almost devoid of it. This is the reverse of the trend expected if the transgression to the hangingwall were still in evidence at this northing. In summary, it seems more likely that the transgression occurs in the mine where it is related to the Mount Isa Fold but not to the north of it in the area studied by Painter et al. (1999).
Timing of fine-grained pyrite deposition
This remains a debated issue. It is agreed (eg. Grondijis and Schouten, 1937; Perkins, 1997; Painter et al., 1999) that fine-grained pyrite everywhere precedes the deposition of sphalerite, galena, and chalcopyrite. Perkins (1998) presented evidence for fine-grained pyrite deposition subsequent to the S3 cleavage and Painter et al. (1999) argued for diagenetic deposition. Although both authors agree that fine-grained pyrite has precipitated primarily in the finely laminated siltstone sequences, there are differences in interpretation of these sequences. Painter et al. base their sedimentological interpretation on the detailed work of Neudert (1983), where the laminated sequences are regarded as being derived from the compaction of the cross-laminated siltstone bases of the graded beds of the rythmite facies. While not being a sedimentological study, Perkins (1997, fig. 4) illustrates correlation of individual laminae over 3 km which would appear to indicate that each lamination was deposited sequentially, and would preclude the whole package being the basal part of a single bed. This publication was not referred to by Painter et al. (1999).
There are significant differences in interpretation of the timing of fine-grained pyrite deposition between these two papers. Painter et al. (their fig. 10) note that fine-grained pyrite appears to overprint the carbonaceous seams in their "laminated siltstone portion of a rythmite facies bed" and larger scale dissolution seams (their fig. 11), indicating that this pyrite "must have occurred either during, or later than, the development of diagenetic dissolution textures in the Urquhart Shale". In terms of an earliest time limit for deposition, this accords with Perkins (1998). However, Swager (1985) and Perkins (1997) argue that carbonaceous seams parallel to bedding are enhanced during one or more periods of deformation prior to the main episode of folding in the Mine. If this is so, pyrite overprinting such seams is necessarily post-diagenetic.
Observations of Painter et al., (1999) and Perkins (1998) appear to be inconsistent with respect to the relationship between fine-grained pyrite and cross-cutting cleavage seams. Figures 8 and 9 of Perkins (1998) illustrate concentrations of fine-grained pyrite along, and also transecting, cross-cutting cleavage (S3) seams, and individual zoned pyrites overgrowing narrow seams. In contrast, Painter et al. (1999, fig. 12) show a series of four wavy bedding-parallel laminae containing abundant fine-grained pyrite. Interstitial mudstones show a well-developed cross-cutting foliation (probably S3). In the text, this foliation is referred to as "carbonaceous" (p.895), but appears in the photograph to be more planar and penetrative than the commonly anastomosing carbonaceous S3 cleavage. If this cleavage is not carbonaceous, then this could explain the lack of fine-grained pyrite along it, and need not necessarily mean that the pyrite is earlier than the cleavage. Perkins’ evidence of post- S3 deposition from a series of examples have not been refuted, however the example of Painter et al. requires re-examination.
Sources of metals and sulphur
According to Painter et al. (1999) all components for the formation of fine-grained pyrite were endogenic to the system with sulphur derived from sulphate evaporites and iron from the carbonates. This is in contrast to Perkins (1997, 1998) where it was argued that local sulphates were replaced by dolomites and quartz before the formation of fine-grained pyrite at a late stage in the deformation history. In this model, both iron and sulphur was introduced. Examples such as Figure 16, Figure 17, and Figure 18 indicate that the sulphur from fine-grained pyrite was not a sulphur source for chalcopyrite deposition. Both studies support a model of sulphate reduction at the site of precipitation, although that of Perkins (1998) does not countenance a bacteriogenic reaction. Painter et al. (1999) provide detailed analysis of the sulphur isotopes of all major sulphide species, and conclude that the Pb-Zn and Cu orebodies probably shared a common sulphur source. They envisaged either a cogenetic model similar to that of Perkins (1997) or a two-stage model. Their synoptic diagram (fig. 20) with lead-zinc orebodies formed while bedding was still horizontal suggests they favoured the latter alternative.