FAQ- 3. Do the mineral assemblages of successive vein generations reflect the fluid compositions at different stages?
Determination of a fluid evolution history for the copper orebodies is, to a large extent, influenced by interpretation of overprinting criteria in the alteration system (Heinrich et al., 1989, Waring, 1990). Of most interest are the depositional conditions and fluids responsible for the economic sulphides. Perkins (1984) and Swager (1985) illustrated and described a sequencing of dolomite and quartz veins and breccias, all of which contained sulphides, principally chalcopyrite. Analysis of veins from epigenetic deposits generally assumes that the veins are essentially dilational, and consequently that all minerals were deposited within each vein generation prior to the subsequent generation. In these scenarios the evolving fluid composition must be capable of depositing the observed sulphides in the overprinting veins. Such an argument has been applied to Mount Isa copper genesis by J. M. Proffet (internal report to MIM Exploration-1992), that since chalcopyrite is observed in veins which have been deformed during the mine folding ( D3 of Perkins 1984 and Main Slaty Cleavage event of Proffett 1992), then this represents the timing of the bulk of the the copper orebodies. Chalcopyrite in still younger veins is thus the result of "remobilization" or a younger generation deposited with the components of that vein. An alternative interpretation where the sulphides are not deposited in any of the veins until the youngest veins have formed, changes the notion of timing of the orebody and implies an evolving fluid history consistent with that late deposition.
Significance of Replacement Veining
A key factor in the mineral deposition evolution is the question of whether the dolomite-silica-sulphide breccia systems are predominantly dilational or replacive. Arguments that dolomitic breccia systems develop from veins which are predominantly replacive rather than dilational, are advanced in Perkins (1984) see example Figure 7. Notwithstanding relatively sharp vein and breccia fragment boundaries, detailed examination shows that vein walls and breccia fragments cannot be fitted together, by movement along bedding and/or cleavage. Even though there has been some movement of fragments the net result is a pseudobreccia resulting from wall rock replacement by coarse-grained dolomite during deformation. Vein systems contain successive generations of dolomite, the earliest of which show deformation twinning. Chalcopyrite exhibits replacive growth across the boundaries of these dolomites, similar to the galena/sphalerite relationship illustrated in Perkins (1997, fig 15e) and shows no evidence of being deformed or of subsequent dissolution.
Figure 7. Shallow east-dipping vein and breccia systems in a dolomitic slate.
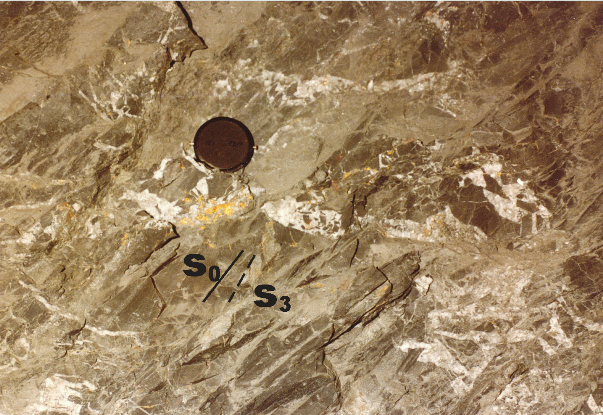
Dolomitic slate showing nature of breccia vein system. Even though fragment and vein margins are quite sharp, many of the breccias are "blind”, the fragments are almost in situ and cannot be fitted together by movement along bedding (S0) and/or cleavage (S3). This shows that wall rock replacement is the dominant process in vein and breccia formation. Replacement of the coarse-grained dolomite by chalcopyrite is quite variable. 18E Sublevel. 4576mN, 1908mE. Looking north.
The youngest veins in the copper orebodies dip at 250-350 east (Figure 8), and commonly contain mostly quartz, even where the earlier veins and breccias are dolomitic. Using the arguments developed in Perkins (1984, 1997) indicating that quartz in the orebody zones is pseudomorphic after dolomite, this suggests that veins of this orientation were preferentially silicified. The stronger association of chalcopyrite with quartz explains why these veins are the most chalcopyrite-rich in the outer dolomitic parts of the "silica-dolomite". Even where cross-cutting dolomite alteration lobes are totally replaced by silica (Figure 9), the shallow east-dipping veins are commonly visible. The relationship of shallow-dipping veins to Mine folding (D3) is illustrated in Figure 10 and Figure 11. These veins are superimposed on an open syncline and a breccia-imposed fault. Chalcopyrite masses have grown along, and over, these post-folding veins. Breccia textures in Figure 11 can be compared with those in Figure 8 with the difference being the deposition of quartz and chalcopyrite at the expense of dolomite. At this scale, as well as at the microscopic scale, there is no evidence of significant dilation associated with chalcopyrite deposition.
Figure 8. Breccia vein complex superimposed on a bedding-dolomitized sequence.
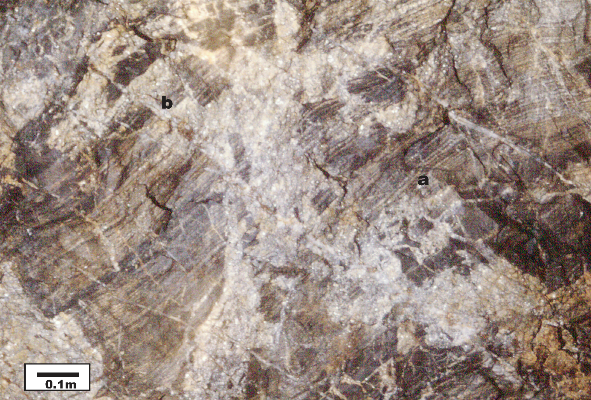
The gently folded bedding (major anticlinal hinge to the right) can be traced through the unrotated residual fragments. Many of the breccias terminate abruptly (eg. a) and are consistent with fracture-controlled replacement by dolomite rather than dilation. Gently east-dipping veins (eg. b )are mostly quartz but show dolomite residuals. Chalcopyrite is locally continuous across the vein boundary. 9 Level, 5415mN. Looking north.
Figure 9. Margin of silicified dolomite lobe.
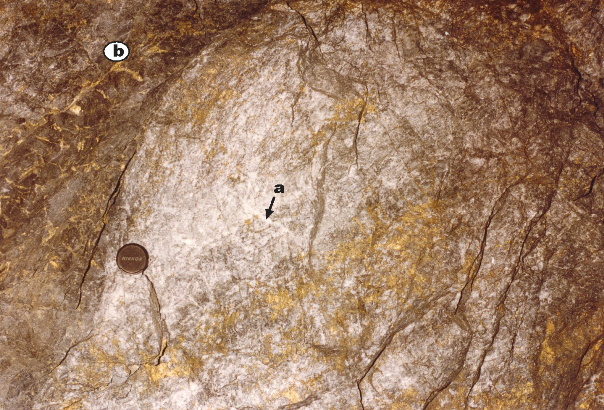
Cross-cutting mottled grey zone of former dolomitic alteration (now totally silicified) showing vague outlines of gently east-dipping veins. The vein (a) above the lens cap is truncated to the right by a chalcopyrite-rich zone along relict bedding, dipping intermediate west. Shallow east-dipping veins in the silicified black non-laminated layer contain high proportions of chalcopyrite (b). 18E sublevel, 4345mN,1972mE. Looking north.
Figure 10. Open D3 fold with silicification and mineralization.
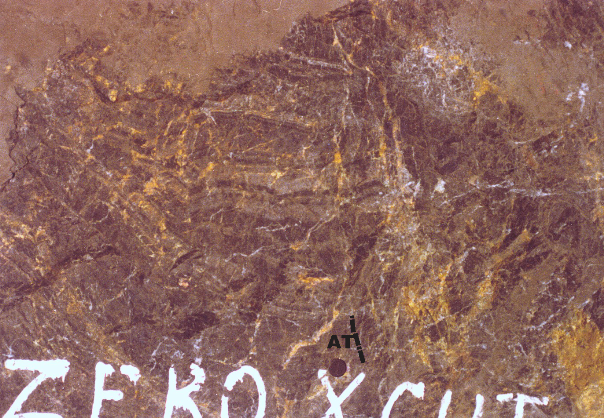
The axial trace (AT) of the D3 syncline is shown. Sub-horizontal and shallow east-dipping veins continue across the fold irrespective of bedding orientation. 1100 orebody, 4500mN. Looking south.
Figure 11. Detail of Figure 10.
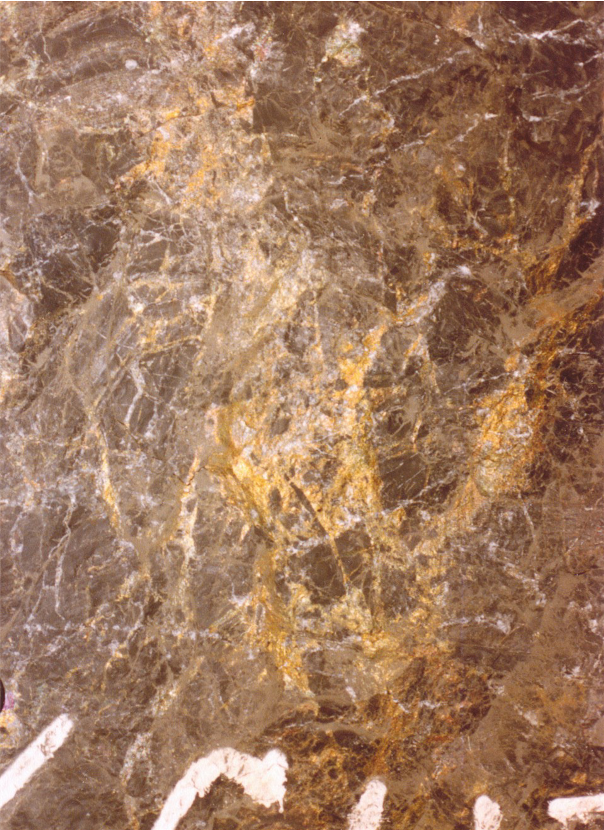
Sub-vertical breccia zone cutting across the fold and following the axial trace of the anticline. Matching of beds shows a displacement across the zone of approximately 0.5m (right side up). The horizontal narrow quartz vein 100mm above "C" has not been displaced but is overprinted by chalcopyrite.
Implications for physico-chemical conditions of sulphide deposition
Deformation throughout the rock mass appears to have been essentially complete by the time of chalcopyrite (and also galena and sphalerite) deposition. Since textures at both meso and micro-scale show volume-for-volume replacement of gangue minerals by sulphides, geochemical models have to account for absolutely minimal porosity. Permeability appears to have been accommodated by dissolution of pre-existing phases by replacement, and fluid conditions must account for mass dissolution of these phases.
There is no evidence for successive generations of chalcopyrite deposition, indicating an evolving fluid which deposited sulphides as the final phase. Heinrich et al. (1989) supported the observations of Perkins (1984) that quartz deposition was pseudomorphic after dolomite, but used fluid inclusion and stable isotope data to argue against a model of simulaneously advancing fronts of dolomitization and silicification. They could not establish conditions under which the NaCl-rich brine responsible for deposition of quartz could have changed through the orebody environment to become the CaCl2-rich fluid involved in the main phase of dolomite growth. It is difficult to determine conditions and fluids responsible for chalcopyrite, galena and sphalerite formation since associated non-sulphides were previously deposited, and conditions could have changed markedly since that stage. More work could potentially be done on fluid inclusions in sphalerite to give more controls on the mineralization stage.