Stop 2. Lower Wog Wog River Section
The base of the Kameruka Granodiorite pluton at this location is characterised by a wide zone of schlieren migmatites or diatexites (Figure 2 and Figure 6).
Schlieren Migmatites
The foliation they exhibit is a well-developed schlieric foliation, which traces out a broad, 2km wavelength, asymmetric, verging S-plunging antiform (see Figure 2: X-section). The migmatites at this location have an overall dip of approximately 45° to the east (parallel to the base of the pluton) and flatten out toward the hinge zone some 1.5km upstream.
The diatexites are characterised by the same, K-feldspar poor leucosome in which drawn out tails of xenoliths and schlieren define the foliation. This foliation is highly sinuous and anastomoses around variable proportions of remnant metasedimentary xenoliths. Some of the relationships between xenoliths and schlieric matrix, and the internal foliations in the matrix, are described here in detail.
Asymmetric xenoliths (Figure 8a) are probably the most common indicator that these rocks have undergone some degree of non-coaxial flow. The xenoliths are cordierite + sillimanite bearing, which suggest that temperature and pressure conditions were in the order of 6500 C and ~3 kbar. The xenoliths often exhibit evidence (e.g. oblique internal foliation orientations, rounded edges and variable axis orientations) to suggest that they have been rotated and rounded within the diatexite, and their terminatons have been drawn into highly elongate, schlieric tails. Such xenoliths are remarkably similar to asymmetric, σ porphyroblasts preserved in mylonites. Some xenoliths show evidence of complete rotation and thus show features more representative of δ porphyroblasts. A sense of shear can be hdetermined from the orientation and asymmetry of these xenoliths within the diatexites, which are magma-rich shear zones.
Figure 8. Shear Fabric in the Schlieren Migmatites
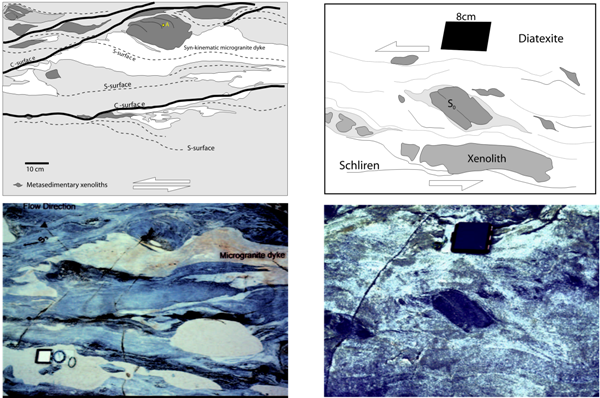
Fig. 8b) This photo shows evidence of a shear fabric developed in the schlieren migmatites. The fabric resembles an S-C fabric developed in mylonites and, like fig. 8a, is evidence that flow occurred in these migmatites in the magmatic state. Fig. 8a) Asymmetric metasedimentary xenolith contained within a matrix dominated by plagioclase + quartz-rich leucosome. The asymmetry exhibited by the xenolith is similar to s -type porpyhyroclasts preserved within mylonites and is evidence to suggest that the migmatites have experienced shearing in the magmatic state as no solid-state deformation is preserved within these rocks.
The second indicator of shear is the presence of variably developed S-C fabrics in some diatexites. In Figure 8b the S-planes are the dominant schlieric fabric and are concordant with a syn-migmatitic, microgranitoid dyke. It is also concordant with the long axes of some xenoliths (top left). The dyke termination marks one of several oblique C planes. Both the S-foliation and the microgranitoid dyke have undergone viscous drag, consistent with the asymmetry of the xenoliths at the top-left of Figure 8b, all of which suggest a component of sinistral/left-lateral movement.
In some examples, a number of metasedimentary xenoliths are stacked in an imbricate fashion (Figure 9a). The xenoliths are usually elongate tabular blocks that are aligned slightly oblique to the flow foliation within the migmatites. The sense of shear is determined by the direction of imbrication. Often, an accumulation of leucosome may occur in the low-strain pressure shadows at either end of the imbricate stack. This feature is also commonly observed at the ends of some single xenoliths that aligned slightly oblique to the foliation and can also be used to determine a sense of movement.
Figure 9. Imbricate xenoliths and Shear and Hybridisation
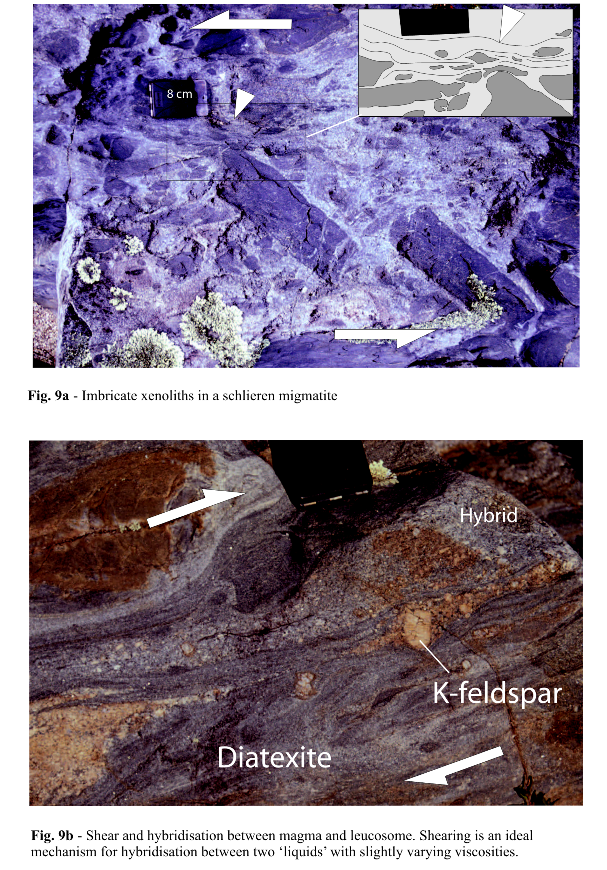
9a) Imbricate xenoliths in a schlieren migmatite. 9b) Shear and hybridisation between magma and leucosome. Shearing is an ideal mechanism for hybridisation between two ‘liquids’ with slightly varying viscosities.
The structures preserved within these migmatites all indicate that they represent shear zones that were active during high temperatures, when large proportions of leucosome facilitated movement. Not only do these structures suggest a component of shear, they can also be used to determine the sense of shear, which is vital when determining the role of migmatites and shear zones in the emplacement of granite plutons. The migmatites located on, and defining, the east dipping limb of the regional antiform show variable movement directions but there is a predominance of indicators showing normal and sinistral motion.
The migmatites in this zone also display evidence of hybridism with the Kameruka Granodiorite (Figure 9b, Figure 10 and Figure 10b). This relation demonstrates that migmatite formation, shear zone development and Kameruka pluton emplacement were all synchronous. The nature of the contact and the relations between the migmatites and the granite provide evidence on both the small-and large-scale processes operating during magma emplacement, as described below.
Figure 10. Hybrids
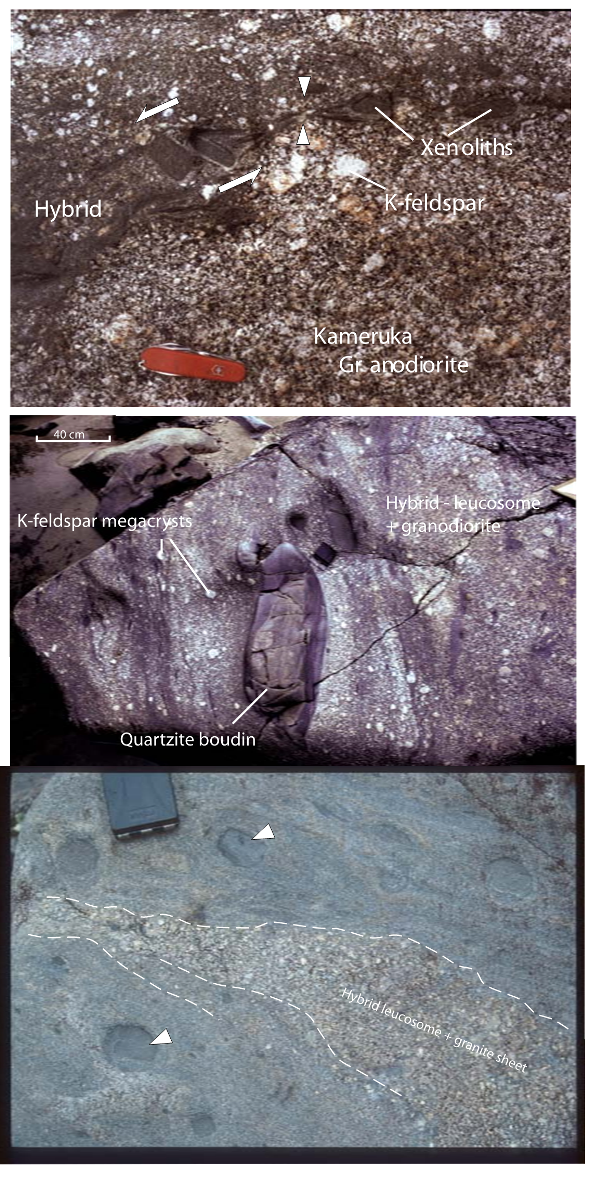
a) Hybrid Kameruka Granodiorite and leucosome. b) Hybrid Kameruka Granodiorite and leucosome. c) Hybrid granite sheet intruding parallel to fabric in migmatites.
The pluton-migmatite contact is characteristically inter-sheeted on a scale ranging from centimetres (Figure 10c) to tens of meters. With increasing proximity to the granite, the strongly foliated migmatites contain a progressively higher proportion of wider granite sheets such that, close to the base of the pluton, wide granite sheets are separated by a few narrow metasedimentary sheets. The orientation of the often narrow, but laterally continuous sheets is parallel with the flow foliation in the migmatites, and parallel to the pluton base. These relationships suggest a strong structural control on the style of emplacement of the Kameruka pluton.
Within the inter-sheeted contact zone, the granite sheets are hybrids that contain variable proportions of leucosome mixed with megacrystic granodiorite. The hybrid sheets always contain a large proportion of easily recognisable metasedimentary material including calc-silicate boudins, cordierite-bearing xenoliths and biotite schlieren that is continuous with the schlieren in the diatexites. Individual granite/hybrid sheet may display a number of features (Figure 10b). Firstly, the sheets may be graded with relation to the degree of hybridism with the migmatites. In this case, the top is often much more hybridised than the base of the sheet, possibly reflecting much more efficient mixing caused by the focus of turbulent flow along the top of the sheets. Secondly, the hybrid sheets may be graded in the concentration of K-feldspar megacrysts – just look at the boulder in my office for a small-scale example. A few sheets show higher numbers of megacrysts closer to the base of the sheet than the top. The hybrid sheets may also display preferred mineral alignment defined by K-feldspar and plagioclase laths.
The migmatite zones are usually slightly less hybridised than the granites. However, they sometimes contain completely isolated lenses or pods of granite surrounded by flow aligned schlieren. On a smaller scale, the migmatites may also contain isolated K-feldspar megacrysts. Both the lenses of granite and the isolated megacrysts are interpreted to be relict grains left behind after flow of the migmatites around a granite sheet. Could a similar process apply for the incorporation of feldspars into mafic magma?
Figure 11. Map of Major Features
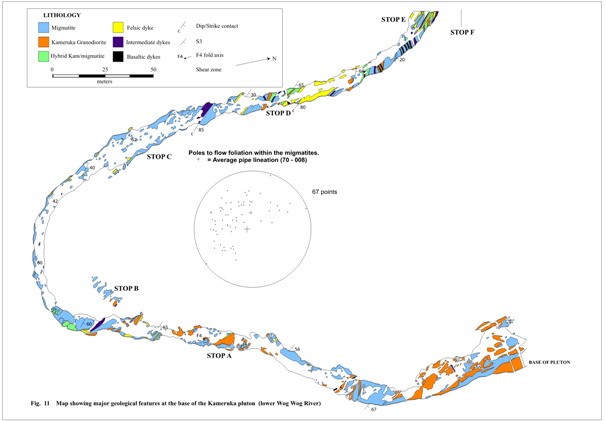
Map showing major geological features at the base of the Kameruka pluton (lower Wog Wog River).
Stop A. Granite Sheet close to the Base of Kameruka Pluton
At this site, metre-scale lenses and sheets of Kameruka granite are inter-layered with migmatite, and are sub-concordant with the schlieric foliation. The granite sheets are typical Kameruka Granodiorite, which is a coarse grained biotite granodiorite with variable K-feldspar megacryst content. The sheet terminate as a series of semi-concordant, narrow tongues, in this case bounded by a narrow mafic hybrid zone that contains isolated K-feldspar grains. The sheets have replaced sections of the country-rock, rather than simply prised them open, and the concordance of foliations with sheets suggests that it happened under syn-tectonic conditions. Locally, only isolated remnants of country-rock are preserved within the granite. These relations suggest that sheeting (parallel to the actively developing schlieren foliation) was probably a general mechanism of emplacement at the base of the Kameruka pluton.
Almost directly adjacent to the granite sheet, the schlieric foliation within the migmatites can be traced from gently east-dipping over a gently south-plunging open fold hinge. The fold has no axial planar cleavage, but at other sites where folds of this nature are observed, leucosome have intruded along the axial plane. This feature indicates that these broad, open folds are syn-migmatitic, having been generated after the flow foliation in the migmatites, yet prior to complete solidification of the leucosomes! In a few localities, granite also can be seen intruding along the axial plane of these folds. The folding displayed at this site is representative of the entire section of migmatites formed at the base of the Kameruka pluton. On the larger scale, the schlieren migmatites appear have been folded into a broad, gently south-plunging asymmetric S-fold (Figure 6). The fold axis to this large antiform lies approximately 2 km to the west. Clearly folding of the migmatites and granite sheets occurred during the final stages of crystallisation, or, cooling and crystallisation of the migmatites and pluton occurred after the initiation of compressive deformation. The exact timing and causes of cooling and crystallisation of the pluton are extremely important for unravelling the mechanisms and tectonic setting of emplacement. Syn-magmatic foliations and axial-planar leucosomes can be interpreted in any number of ways. The interpreted tectonic setting for emplacement will vary considerably depending on which interpretation is adopted. We should discuss some of the possible interpretations and implications.
Stop B. Opposing Senses of Movement in the Same Outcrop
A number of key features relating to sheeting of the granites and syn-migmatitic schlieren are displayed within this outcrop (Figure 9b). The most obvious feature is the medium grained, orange coloured granite lens contained within the schlieren. In the same foliation plane lies a single K-feldspar megacryst in a narrow granite sheet. The megacryst appears to have become separated from what was a more laterally continuous sheet of granite that was boudinaged during shearing. Directly above, a narrow granite-migmatite hybrid sheet shows evidence of a similar process, although it maintained along-strike continuity. Nonetheless, the sheet is reduced from -8cm to <1 cm from right of to left of the outcrop. These sheets of granitic material must have been magmatic during development of the foliation, and are therefore syn-tectonic.
Foliation in the diatexite was ascribed to non-coaxial flow at the beginning of this section. If so, sense of shear should be observed. In the rocks at Stop B, these include: 1. Rotated K-feldspar megacryst containing granite within the strain shadow at either end of the grain, 2. Asymmetry displayed by the cordierite bearing xenoliths with associated schlieric tails and 3. Weakly developed C-planes aligned at low angles to the primary foliation of the migmatites. These features suggest that the movement is dextral, or top to the right. This is opposite to the overall sense of movement determined for these migmatites.
Stop C. Asymmetric Xenolith
The migmatites at this site provide an excellent example of xenolith asymmetry and schlieren foliation development. The diatexites contain a high proportion of leucosome, with few metasedimentary xenoliths (Figure 8a) that are lensoid, with rounded comers and well-defined 'tails' defined by biotite schlieren. The asymmetry of the tails is similar to that of σ porphyroblasts in mylonites, suggesting a left-lateral sense of movement, which corresponds to the predominant shear sense determined for much of the migmatite zone.
Approximately 2m closer to the river, a semi-coherent block of mesosome can be seen breaking up in to smaller, blocky fragments separated by leucosomes that are concentrated in the interstices. It is possible to view this outcrop as an intermediate stage in the progressive disaggregation of large metasedimentary xenoliths into smaller, more common xenoliths. The individual fragments of the larger block appear to have been rotated during intense near-field shear movement. As the blocks rotated, leucosome has penetrated the voids or low-pressure zones created between the fragments. The progressive disaggregation of this xenolith highlights the dynamic nature of this zone during formation.
Stop D. Hybrid Zone
This site shows localised mixing between intrusive granitic melts and diatexite leucosome during emplacement of the Kameruka pluton. Central to this site is a 1-2m thick sheet of Kameruka granodiorite which dips to the northeast, concordant with the strongly foliated migmatites. Along the upper and lower margins of this sheet, the diatexites have variably mixed with megacrystic granodiorite to produce metre-scale zones of heterogeneous hybrid magma (Figure 10b).
Many typical features of hybrid zones can be soon at this site. These hybrid zones contain isolated grains of euhedral K-feldspar megacrysts, large anhedral quartz, euhedral plagioclase, and biotite grains comparable to those of the granite, dispersed in varying proportions throughout a much finer-grained groundmass of leucosome. The hybrid sheets also contain abundant, variably rounded pelitic and psammitic xenoliths, and calc-silicate nodules. Biotite schlieren in the hybrids is commonly continuous with schlieren in the migmatites and locally anastomoses around larger metasedimentary xenoliths. Biotite schlieren define the flow foliation in the hybrids, but it is enhanced by the preferential alignment of tabular plagioclase and K-feldspar megacrysts. It is also concordant with the adjacent granitic sheets.
The granitic sheets represent the injection of granitic magma along a high-temperature, leucosome-rich, shallow-dipping shear zone. The hybridised margins of these granitic sheets are interpreted as a deformation-induced feature where mechanical mixing has occurred between crystal-poor magma and host diatexite to produce contaminated granitic sheets and hybrid diatexites. Locally, the hybrids are remobilised as sheets that intrude into metatexite (Figure 12). In this example, the hybrid sheet is graded, suggesting settling of xenocrystic K-feldspar during flow. The discordance of metatexite foliation on either side of this dyke suggests that the dyke was remobilised into an active shear zone. These relations confirm the syntectonic granite sheeting during migmatisation.
Figure 12. Granite-migmatite hybrid dyke
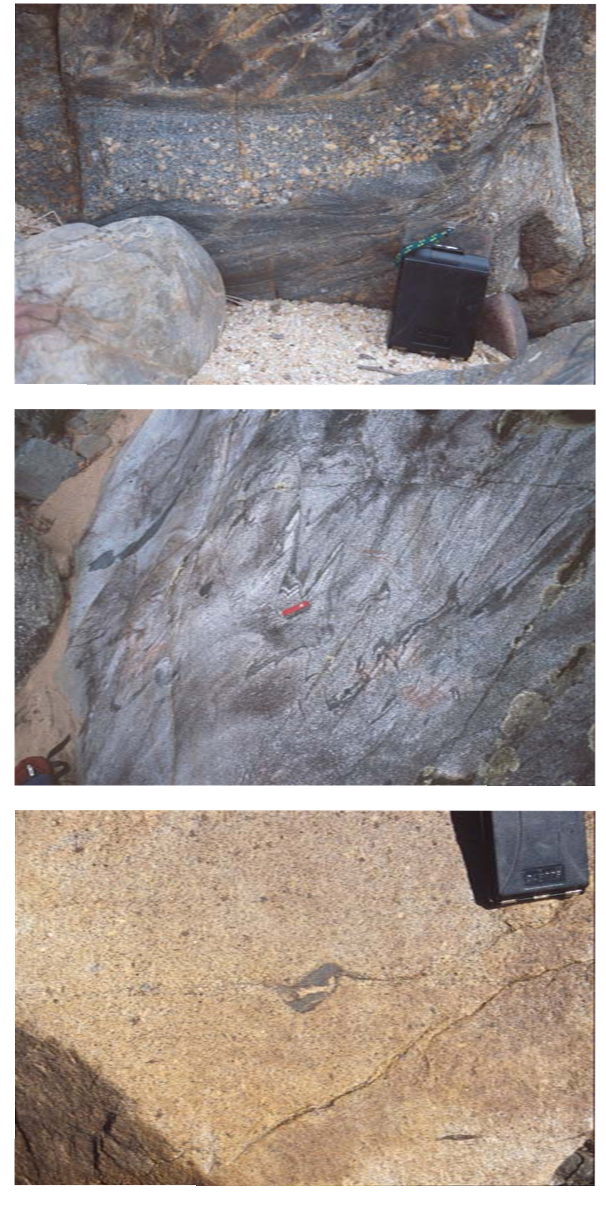
a) Granite-migmatite hybrid dyke showing size sorting of xenocrysts, with larger crystals concentrated on the lower side. Note the discordance of migmatite layering (S3) in either side of the dyke, suggesting intrusion into an active shear zone. b) Magmatic folds in microgranite dyke. c) Rotated metasedimentary xenolith within microgranite dyke suggesting shear flow during emlacement.
Chemistry of Hybridisation between Migmatite and Kameruka Pluton
(This section is based on work by Bryce Healy who is currently in the final stages of his PhD at Newcastle University)
Ten analysed hybrid rocks range in composition between 68-73% SiO2 and define a relatively scattered trend between the Kameruka Granodiorite and the metasedimentary trend. They plot close to the analysed leucosome from the diatexites (Figure 13). The chemical scatter can be confined between these three components; the metasediment, the leucosome (~75% SiO2, and the Kameruka Suite at the mid-point of its linear array (~69% SiO2). The array suggests the hybrid is a mixture of all three components, which is consistent with the field relations.
Figure 13. Selected Harker variation diagrams
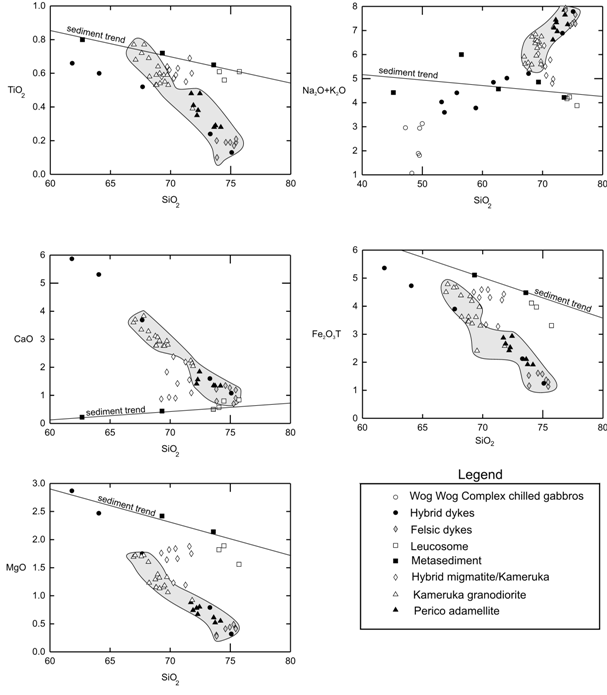
Selected Harker variation diagrams for the Kameruka supersuite (KSS) and associated rock type s. The enclosed field shows the compositional range of the KSS granitoids. The solid line represents the linear trend defined by analysed metasediments. These diagrams show that the hybrid migmatite-granite samples define a relatively scattered trend between the high SiO2 end of the Kameruka granodiorite array and the metasediment trend close to the analysed leucosome from the migmatites. (Acknowledgement must go to Bryce Healy for analysis and diagram preparation).
Figure 13b. Selected Harker variation diagrams - chemical variation
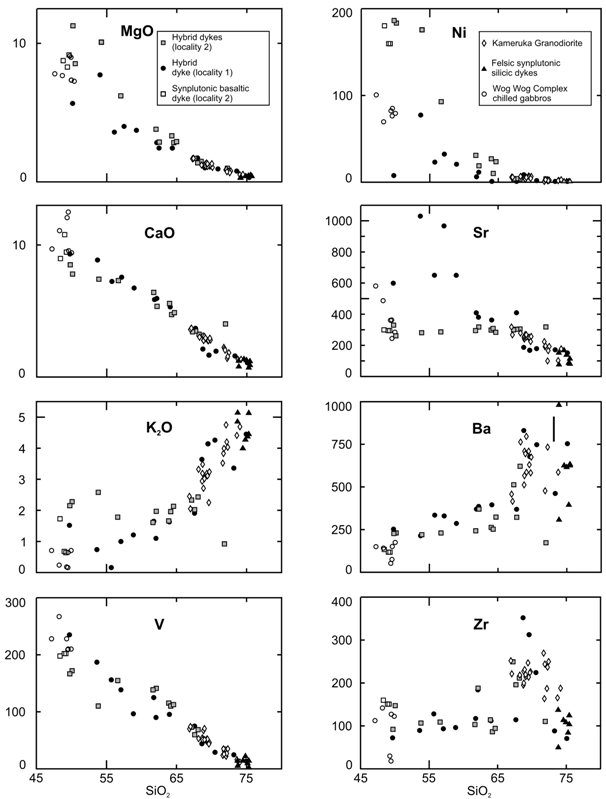
Selected Harker variation diagrams which show the chemical variation in composite dykes
Two samples of granite sheets within this intersheeted contact zone were also analysed. These granite samples fall off the linear trend for the Kameruka Supersuite data and are slightly displaced towards the metasedimentary trend. Biotite mineral compositions for these hybrid rocks and the two granite samples are intermediate between the biotite compositions of the leucosome/sediment and the Kameruka granodiorite (Figure 14). The biotite chemistry not only confirms the intermediate nature of the hybrids, it also confirms the whole rock data that indicates local contamination of the granite by the diatexite.
Figure 14. Biotite Mineral Compositions
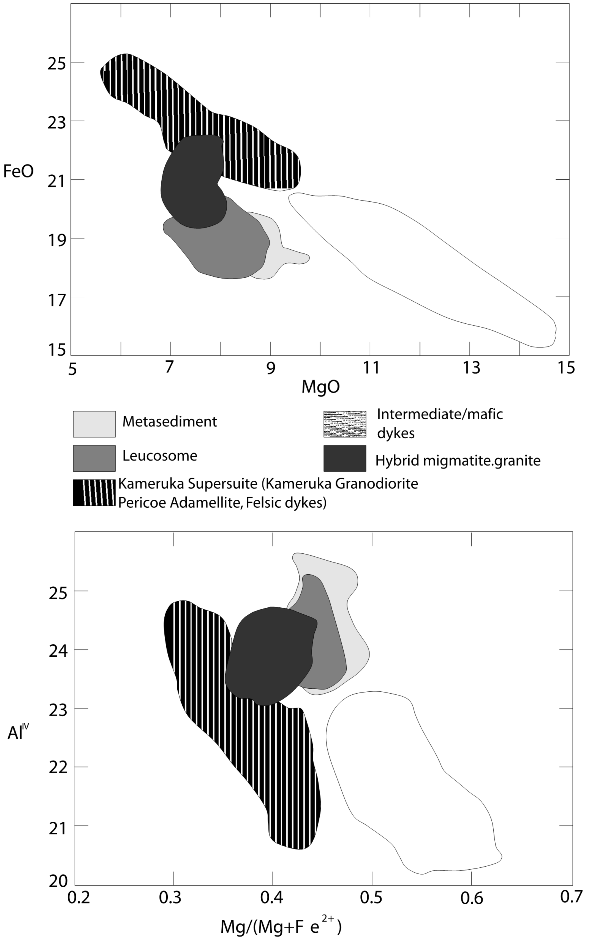
Part of (a) FeO vs MgO and (b) Al iv vs Mg/(Mg +Fe2+) for biotite mineral compositions taken from the Kameruka Supersuite (KSS) leucosome, metasediment, hybrid migmatite-granite and a range of intermediate and mafic dykes. These plots show that the biotite compositions from the hybrid migmatite-granite sit between the leucosome/metasediment and granites from KSS. Diagram thanks to Bryce Healy.
Field evidence, whole rock and mineral geochemistry at this stop suggests that hybridisation involved multicomponent mixing between granitic crystal mush, leucosomal melt and residual fragments of metasediment. The presence in the hybrids of large mineral grains from the granite and refractory fragments from the metasediments, suggests that the granitic sheets were emplaced as highly viscous crystal-rich magmas, which mechanically disaggregated along their margins into leucosomes dominated diatexite. The parallelism of foliation in the granitic sheets, hybrid margins and diatexites suggests that injection and mixing were facilitated by deformation associated with shearing.
Stop E. Synmagmatic Folding in Microgranitoid Feeder Dyke
Within one of the large microgranitoid dykes, biotite schlieren define a series of highly irregular, asymmetric, semi-continuous folds that vary in both style and orientation (Figure 12b). The folds are characterised by variable axial-plane orientations and have strongly attenuated limbs suggestive of formation in a shear/flow dominated setting. The biotite schlieren are considered to represent either the boundaries of individual pulses of magma injected parallel to the margins of the dyke, or sorting of mafic minerals during laminar flow in the dyke. The folding itself may have resulted from differences in magma flow rates within the dyke. Further support for variations in the flow rates within the dyke and the formation of these zones comes from an excellent example of a rotated metasedimentary fragment within a dyke, (Figure 12c), which is very similar to δ porphyroblasts formed by sinistral rotation within mylonites.
Stop F. Composite dykes, silicic pipes, and causes of mafic-silicic hybridism Silicic Pipes
This site represents a composite dyke in which a felsic and mafic dyke have come into contact when both magmas were still molten. The synchronous intrusion of these dykes has produced a large composite body containing delicate and spectacular pipe and diapir-like structures defined by the felsic melt, as illustrated in Figure 15.
Figure 15a. Silicic Pipes
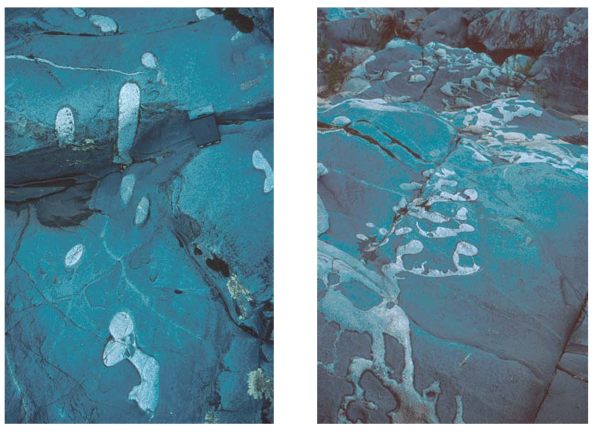
This series of photos shows the arrangment of silicic pipes and sheets developed within a composite dyke. See text for a complete description of these amazing features.
The dyke itself is bounded by migmatites to the east and Kameruka Granodiorite to the west. A flow foliation within the adjacent Kameruka Granodiorite and the migmatites is truncated by the composite dyke, which shows that it postdates the main phase of migmatite formation. However, mingling relationships between the felsic melt within the composite body and the surrounding Kameruka Granodiorite suggests that the formation of the composite body is coeval with the final stages of crystallisation of the Kameruka Granodiorite.
The array of structures developed in the dyke have been interpreted as resulting from rising of the less dense felsic melt and is therefore gravitationally controlled (e.g., Wiebe 1993). The pipe and sheet structures can be used to infer the original orientation of vertical in these rocks during cooling of the migmatites and granite. The internal geometry of the composite dyke is primarily characterised by two sets of sheets that are distinguished from each other by their orientation and the pipes. The first set of sheets dip shallowly to the west and form the surface to which the pipes and a second set of sheets join.
The average lineation orientation (8 measurements) of the pipes is 70-008. As felsic material attempts to rise, it forms small diapirs and pipes that reflect the original vertical attitude of the dyke. In effect, the pipes were originally a sub-vertical lineation. The northerly plunge exhibited by the pipes suggests that the terrain has undergone a southerly tilt of ~10°. This is consistent with a shallow southerly plunge on the megascopic fold defined by the migmatites.
Geochemistry
Geochemical data for a range of rock types associated with the Kameruka pluton are presented in Figure 13b. Data for the Kameruka pluton is from Beams (1980) and the remaining data, obtained by standard XRF techniques. The range of rock-types include the intermediate composition enclaves, back-veins and host-rocks of composite dykes at locality 1. Silicic dykes, many of them syn-plutonic, were sampled from locations below and within the pluton. Wog Wog Mafic Complex chilled gabbro compositions are presented to demonstrate their similarity to mafic and intermediate syn-plutonic dykes.
The composite dykes exhibit a complete spectrum from basaltic (48% SiO2 to granitic (76% SiO2 compositions. The most silicic dykes resemble haplogranite compositions, whereas the most mafic component closely resembles chilled gabbros of the WWGC. The dykes form a smooth, sub-linear to curvilinear array between these two end members, for almost all elements. This applies particularly to FeO, MgO (Figure 13b) and the compatible transition elements, such as Ni and V (Figure 13b). CaO also forms a sub-linear array trending toward the WWGC (Figure 13b), but Sr shows enrichment relative to WWGC at intermediate compositions (Figure 13b), indicating decoupling of the Ca-Sr system. Ha (Figure 13b) generally follows the sub-linear K2O trend (Figure 13b), although the unusual Ba enrichment in some more felsic dykes suggests contamination with the Ba-rich host Kameruka granite. The same felsic dykes have the elevated Zr contents of the host granite (Figure 13b), confirming limited interaction with the surrounding crystal mush during injection (recall that some felsic dykes are from within the pluton, rather than from a locality below the pluton).
The chemical data show a sub-linear array for most elements, with end-members represented by the syn-plutonic silicic dykes and the WWGC chilled gabbros. This array supports a mixing model involving these mafic and silicic end members to produce the compositional spectrum seen in the composite dykes.