The Aston and Hospitalet massifs form the largest domal structures in the Axial Zone and consist of large orthogneiss cores mantled by Cambro-Ordovician metasedimentary rocks. The two domes are separated by a narrow E-trending mylonite zone, the Merens Fault, which was active during the latest Variscan or the Alpine deformation (Figure 4, Carreras & Cirés 1986). Cambro-Ordovician rocks are overlain by Silurian and Devonian slate, separated from the Northern Pyrenean Zone by the steeply dipping North Pyrenean Fault. To the south, Cambro-Ordovician rocks are intruded by the late Variscan Mont Louis-Andorra pluton, and locally overlain by Silurian and Devonian slate in the southwest. The Soldeu-Lanous fault, a mylonite zone considered to be of the same age as the Merens fault, marks the southern boundary of the Hospitalet orthogneiss (van den Eeckhout 1986).
Figure 4. Geological overview
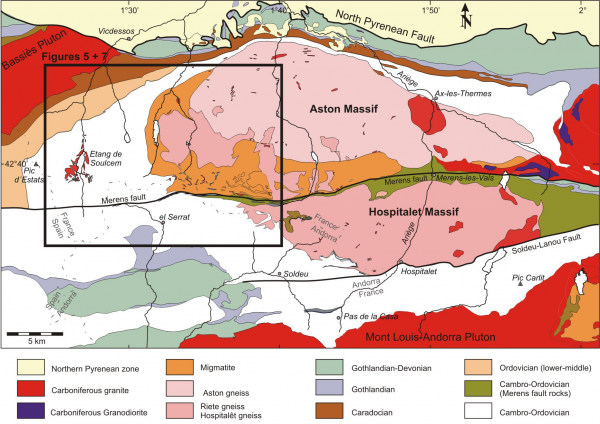
Geological overview of the Aston and the Hospitalet domes with location of Figures 5 and 7. Modified after Casteras et al. (1969), van den Eeckhout (1986) and Besson (1991).
The Cambro-Ordovician metasedimentary rocks are commonly graphite-bearing pelitic rocks composed of, depending on metamorphic grade, quartz, chlorite, mica, garnet, staurolite, andalusite, sillimanite, cordierite. Common accessory phases are zircon, apatite and ilmenite. Plagioclase occurs locally where it can be a major component. Epidote and tourmaline are locally occurring trace minerals. Horizons of marble and quartzite up to 100 m thickness, and mapable conglomerate and mafic lenses are intercalated with the metapelitic schist (Besson 1991, Casteras et al. 1969, van den Eeckhout 1986). Aplitic and granite pegmatite dikes intrude the metasedimentary rocks.
The orthogneisses are commonly garnet-bearing two mica granite gneisses, subdivided further based on appearance (Besson 1991, Casteras et al. 1969). A penetrative gneissic foliation is present throughout the domes, and development of augengneiss can be observed (van den Eeckhout 1986). The protolith age of the orthogneisses is not known.
A broad zone mapped as pelitic migmatites mantles the western and southern margin of the Aston gneiss (Besson 1991, Casteras et al. 1969). However, field work for this study has shown that in the western part the migmatites resemble a coarse paragneiss with a well preserved gneissic foliation. Actual migmatites, rocks that display signs of partial melting (leucosomes) or schlieren fabrics, have been observed closer to the contact with the gneiss. They are labelled as "M" on the map (Figure 5). The orthogneiss also experienced migmatization.
Figure 5. Foliation trend and mineral lineation
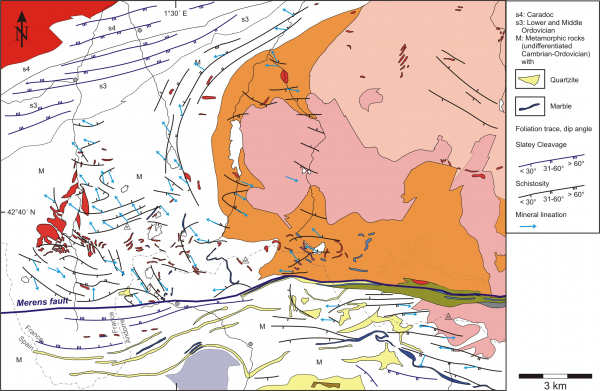
Foliation trend and mineral lineation of the western Aston and Hospitalet domes. Same geological symbols as in Figure 4. For clarification the colours of the Caradoc and Lower to Middle Ordovician units were replaced by signatures.
The orientation of original bedding of the Cambro-Ordovician metasedimentary rocks can still be recognized in the Hospitalet dome. In the eastern part of the dome, bedding is steeply northerly dipping, while in the western part bedding is folded by southwesterly dipping folds with easterly vergence (van den Eeckhout 1986). The major foliation, referred to as S2, is steeply northerly to northeasterly dipping in the eastern part. In the western part the main schistosity is E-W striking with predominantly moderate to steep southerly dips that grade to steep northerly dipping slaty cleavage further west, towards the border with France and Spain (Figs. 5, 6). A younger, generally steeper dipping crenulation cleavage of similar orientation locally overprints S2. A well developed mineral lineation, defined by mica on S2, is shallowly plunging with easterly trends (Figure 6). A foliation and lineation developed in the orthogneiss have orientations similar to those in the mantling metasedimentary rocks (Figure 6). van den Eeckhout (1986) traces the main schistosity of the metasedimentary rocks into the gneiss, but also notes an angle between the foliation along the contact, which is interpreted as the result of later mylonitic deformation.
Figure 6. Stereographic projection
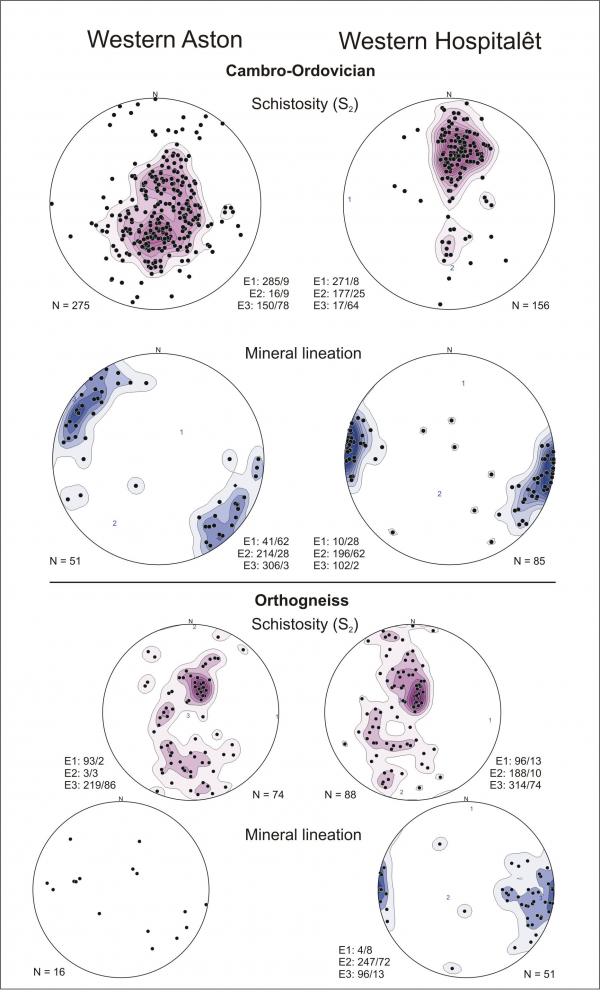
Stereographic projection of the major foliation and lineation in Cambro-Ordovician schist and orthogneiss. Data of the slaty cleavage, outlined in blue in Figure 5, is not included. Equal area, lower hemisphere projection with Schmidt net.
North of the Merens fault the orientation of foliation is more varied (Figure 5). Original bedding can be observed, though difficult to measure, in the lower grade slates along the northern flank of the Aston dome. The slaty cleavage is steeply dipping to subvertical with easterly strike orientation in the eastern and northern part of the dome grading into an east-northeasterly strike direction in the western part. The dip direction is generally northerly, but easterly dips prevail along the contact with the Bassiès pluton (Figure 5). If this is due to folding or represents dip fanning related to the intrusion of the Bassiès pluton remains to a conjecture. In the higher grade schistose rocks, isoclinally folded, sheared quartz layers are indicative of an older foliation. The main schistosity, which is referred to as S2, is a spaced schistosity which grades into a continuous schistosity with increasing metamorphic grade towards the contact with the orthogneiss. At the eastern termination of the Aston gneiss the schistosity wraps around the gneiss, dipping moderately to steeply east to southeast (Bon et al. 1994). In the western Aston dome near the gneiss core S2, strikes southwesterly with moderate northwesterly dip angles, and gradually shifts to northerly and southeasterly strikes with shallow westerly dip angles. Six kilometres west of the orthogneiss margin, orientation of S2 varies considerably. This can be attributed to late Variscan granites that are centred around the Etang de Soulcem, which deflected the schistosity during intrusion (Verhoef et al. 1984). The Merens fault, which is difficult to localize in the field, nevertheless results in a marked structural break. The slates to the south have a steeply northerly orientation, while the schist to the north vary in dip direction, but maintain a shallow attitude. This strongly suggests that the Merens fault postdates development of S2. As a result, S2 yields a wide clustering on stereographic projection, a distinct different appearance than the semi-girdle of the S2 in the western Hospitalet dome (Figure 6). Orientation of mineral lineation is also wider dispersed and with an average trend of 126° slightly oblique (24°) to lineation in the Hospitalet dome, although with similar shallow plunge angles.
The foliation and lineation in the Aston orthogneiss display a similar dispersion as those of the mantling schist, and are very similar to that of the Hospitalet gneiss (Figure 6). This suggests that development of S2 in the schists of the Aston dome is coeval with that of the foliation in the Aston gneiss, although this could not yet be supported by direct field observation.
Mylonitic zones are observed in the proximity of the Merens fault (Carreras & Cirés 1986) and as steep discrete metre-scale mylonitic bands throughout the Cambro-Ordovician schist (Soula et al. 1986).
Shear sense indicators in the mica schist are not very common, and are mostly c'-type shear bands (Figure 13). The inferred sense of shear is generally top-to-the-ESE or hanging wall up. Most of the shear sense indicators observed are located close to the contact with the orthogneiss. Other kinematic indicators such as boudinaged and/or rotated quartz veins are disregarded because of ambiguous interpretation.
Characterization of the metamorphic grade is established by means of metamorphic mineral assemblages and textural relationships observed in thin sections, which are listed on Tables 1 and 2. This method is preferred over mapping mineral or reaction isograds or metamorphic mineral zones. These methods all require very detailed sampling and knowledge of chemical equilibrium and bulk rock composition, which are very difficult to establish in this terrain. As a compromise, the first or last appearance of suitable major phases is projected as a line on the map (Figure 7). Important equilibria reactions which are inferred from the observed assemblages and textural relationships are listed on Table 2. It should be noted that there are no mineral analyses by electron microprobe or whole rock chemistry yet available in this stage of the study.
Figure 7. Metamorphic mineral assemblages
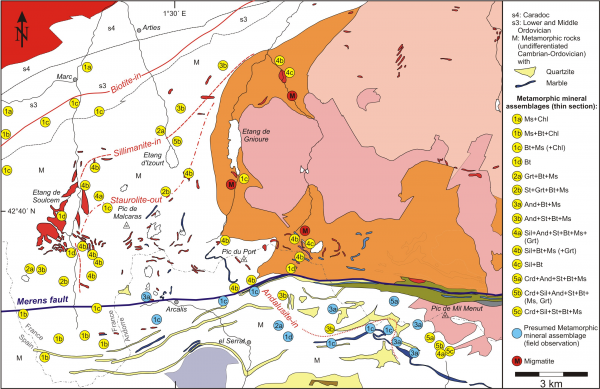
Metamorphic mineral assemblages and selected mineral isograds for the Cambro-Ordovician metasedimentary rocks of the western Aston and Hospitalet domes.
Outside the narrow contact aureole of the Bassiès pluton, near the villages of Marc and Arties, the lowest grade rocks are fine grained Ordovician slates. The slates possess a spaced cleavage and have average grain sizes of 50 µm. They are composed of quartz, muscovite and chlorite (assemblage 1a). Towards the southeast the grain size increases to approximately 100 µm, coinciding with the appearance of biotite (assemblage 1b, Figure 8a). With increasing biotite abundance, the amount of chlorite diminishes (assemblage 1c, Figure 8b). The local occurrence of biotite-quartz schist (assemblage 1d) appears not to be correlated to metamorphic grade and is most likely the result of bulk rock composition.
Figure 8. Thin section photographs from the western Aston dome
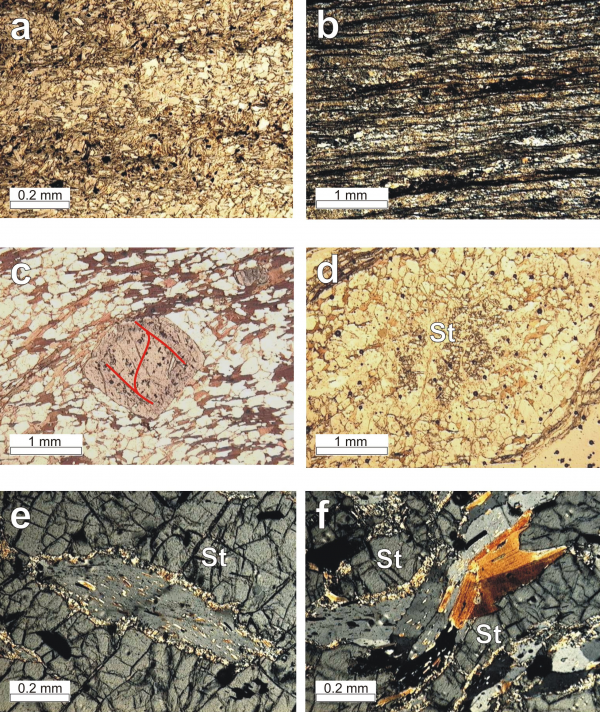
Fine grained muscovite-chlorite slate (assemblage 1b, sample 98-97). Plane polarized light (PPL).
Spaced cleavage developed in a muscovite-chlorite- biotite schist (assemblage 1c, sample 98-101). Crossed polarized light (XPL).
Garnet with graphite inclusion trails preserving an earlier crenulation cleavage. PPL.
Xenoblastic staurolite in a biotite-quartz matrix, partially altered to sericite (assemblage 2b). PPL.
Quartz lens in staurolite with parallel white mica and opaque inclusions.
Quartz lenses with folded inclusions overgrown by staurolite. XPL.
The next minerals to appear are garnet and staurolite (assemblages 2a and 2b). Garnets are commonly subhedral and contain inclusion trails of quartz and graphite. In some samples garnets display straight inclusion trails that are commonly obliquely oriented to the external foliation. In some localities inclusion trails in garnet preserve an older crenulation cleavage (Figure 8c). Periodical garnet growth can be inferred in some cases from zonation evident from a dusty core and a clear rim. Garnets commonly occur as an accessory phase, seldom amounting up to several weight percent, with sizes up to 1.5 mm. Large, centimetre sized poikiloblastic staurolite occur in garnet-bearing schist (assemblage 2b). Staurolite can form subhedral to anhedral, often twinned, porphyroblasts or have an irregular xenoblastic shape (Figure 8d). Alteration at the rims to fine grained white mica (sericite) is very common. Inclusions of opaque phases and quartz can form trails that are straight, often oriented obliquely to the external foliation, suggesting a relative rotation of the staurolite porphyroblast. At some localities, the inclusion trails trace a crenulation cleavage, which is absent in the matrix, indicating that the staurolite porphyroblast nucleated prior to the development of the present schistosity (Figure 8e, f). Smaller grain size of quartz found as inclusions than those in the matrix also suggest that staurolite grew at a time when the rock was finer grained. Due to the lack of textural relationships the sequence of appearance of garnet and staurolite can not be determined. Both phases can be viewed as the result of the breakdown of muscovite and chlorite:
muscovite + chlorite + quartz = almandine + annite + H2O
muscovite + chlorite = staurolite + biotite + quartz
The staurolite-forming reaction (2) also produces biotite, which appear as large biotite porphyroblasts truncated by the present schistosity.
The most prominent metamorphic phase to observe in the field is andalusite. It can form decimetre-sized porphyroblasts that are easily distinguished on the schistosity plane (Figure 9). Notable exposures are at the Etangs de la Gardelle, 1.5 km southwest of the Etang de Soulcem, and south of l'Estany Primer northwest of the Arcalis ski station in Andorre. The length axes of the andalusite blasts are randomly oriented, indicating absence of significant simple shear component during growth. Some twinned blasts also display significant growth across the foliation plane (Figure 9), suggesting that compressional strain was also negligible. Thin sections show that most andalusite porphyroblasts are subhedral and poikiloblastic with inclusions of biotite and quartz, and in places complete quartz layers. The inclusions commonly form trails that are either straight or concave, indicating interkinematic or synkinematic growth under coaxial deformation. Generally, the internal foliation continues into the external (Figure 10a). Oblique orientation of the internal foliation with respect to the external schistosity indicates postkinematic rotation of the blast. Centimetre-sized blasts can display curved inclusions that are the result of bending of the andalusite grain, evident in the continuous, fan-like extinction under crossed polarized light. Large broken blasts are also common. Alteration at the rims to fine grained white mica (sericite) is not uncommon. Two distinct generations of andalusite in one sample as proclaimed by Verhoef et al. (1984) were not seen. Along with andalusite, large biotite porphyroblasts also occur in the matrix (Figure 10b), suggesting the following reaction:
Figure 9. Block diagram of andalusite twins
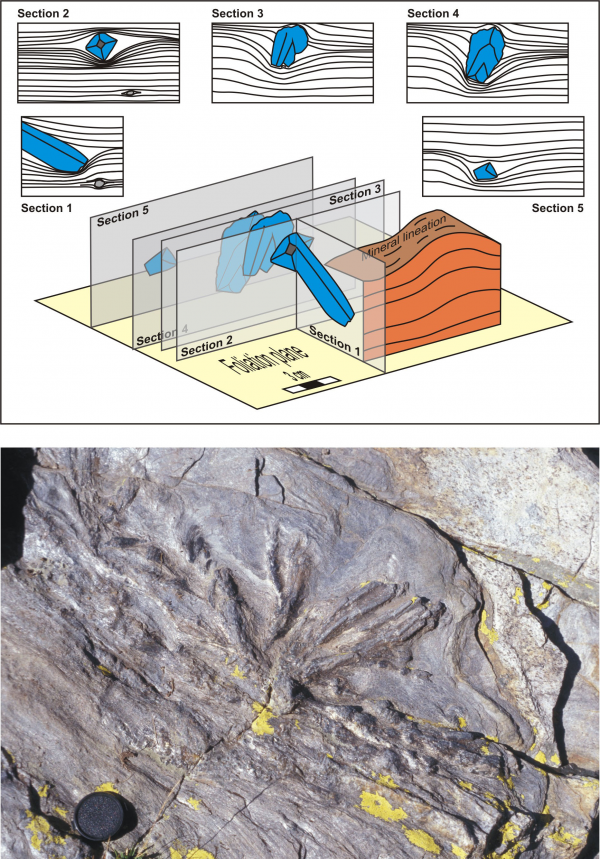
Block diagram of andalusite twins in a fine grained mica quartz schist from a locality southeast of the Soulcem granites. Section 1 is cut perpendicular to the mineral lineation, defined by muscovite grains, sections 2 to 5 are oriented parallel to the lineation. The twins are not aligned within the foliation plane, but describe an up side down "V". Also note that the andalusite twin in the foreground is tilted in opposite direction with respect to the mineral lineation than the twin in the background. Photograph shows radiating andalusite megablasts apparently growing in random orientation on foliation plane.
chlorite + muscovite = aluminosilicate + biotite + quartz + H2O
Coexistence of staurolite and andalusite (assemblage 3b) without any indication of resorption of any phase is seen as indication that both phases are part of the same assemblage. In that case reactions (2) and (3) take place simultaneously.
With the first appearance of fibrolitic sillimanite (assemblage 4a) staurolite displays signs of resorption. Staurolite can be found as relic, with embayed grain boundaries or completely xenomorph (Figure 10c), within andalusite. Large biotite inclusions in the surrounding andalusite (Figure 10d) indicate a staurolite-consuming reaction to form andalusite and biotite:
Figure 10. Thin section photographs from the western Aston dome
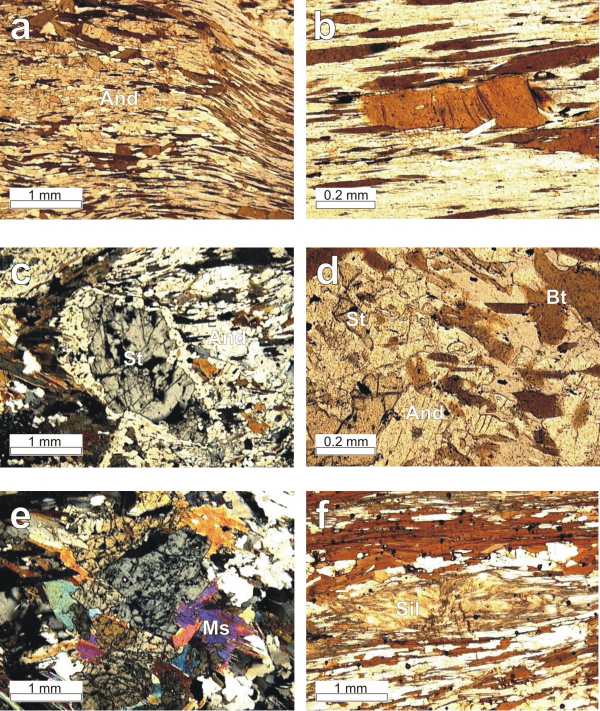
(a) Poikiloblastic andalusite with inclusion trails that continue straight into the external foliation and
(b) older biotite grain truncated by new schistosity (assemblage 3a, sample 98-114). PPL.
(c) Staurolite inclusion within andalusite displaying corroded rims (assemblage 4a, sample 98-73). XPL.
(d) Same sample showing new biotite growing within andalusite near a relic staurolite. PPL.
(e) Staurolite from the same sample rimmed by large muscovite, interpreted as retrograde reaction. XPL.
(f) Fibrolite lens completely replacing biotite in a sillimanite-biotite schist (assemblage 4b, sample 98-127). PPL.
staurolite + chlorite + muscovite + quartz = aluminosilicate + biotite + H2O
Staurolite that is not a relic in andalusite is rimmed by large muscovite porphyroblasts that overgrow the main foliation (Figure 10e). In places the original staurolite crystal is reduced to relic or has disappeared completely, so that muscovite porphyroblasts pseudomorph after staurolite. In theses rocks chlorite is also abundant as an alteration product. The textural relationships suggest that staurolite replacement in this case is not a prograde, but a retrograde metamorphic reaction, a reversal of the staurolite-forming reaction (2) (Guidotti & Johnson 2002).
A very common assemblage (4b) consists of sillimanite, biotite, muscovite and quartz, which is found east of the Soulcem plutons and close to the migmatitic zone of the western Aston gneiss. Fibrolitic sillimanite is first seen (assemblage 4a) nucleating in quartz. With increasing abundance the fibrolite needles grow epitaxial in biotite. No evidence for replacement of andalusite is found. Although primary muscovite is decreasing with increasing fibrolite abundance, fibrolite most likely did not form by reaction (3). First, there is not enough primary chlorite left. Second, nucleation of fibrolite on biotite suggests that fibrolite replaced biotite. Large, millimetre-sized muscovite grains overgrowing cleavage domains are observed near fibrolitic lenses. Kerrick (1987) and Kerrick & Woodsworth (1989) proposed that fibrolite had formed the decomposition of biotite, due to removal of K, Mg and Fe caused by acidic fluids emanating from adjacent magmatic intrusions. Contemporaneous muscovite would act as a local K sink. Fibrolite can amount up to 20 vol. %, completely replacing individual biotite grains, and form millimetre-long fibrolite lenses, which are characterized by foliation-parallel needles at the margin and irregularly oriented needles in the centre (Figure 10f). Spry (1969) referred to this process as irregular growth following seeded nucleation. In schists with a high abundance of sillimanite, prismatic sillimanite grains can be observed in the central part of the fibrolite lenses.
Coinciding with increasing sillimanite abundance the rocks become coarser grained and possess a continuous schistosity, attaining a gneissic appearance. Although some of the samples of the assemblage (4b) lie within the zone mapped as migmatitic by Casteras et al. (1969), the rocks generally have a well developed gneissic foliation as outlined in section 3 a., and should better be referred to as paragneiss. True migmatites have been observed closer to the actual contact with the orthogneiss.
In the southern migmatite zone, sillimanite-biotite schist (00-104) contain large centimeter-sized poikiloblastic garnets with inclusions of plagioclase, quartz, biotite and opaques that form distinct parallel layers. The shapes of the inclusions are irregular and the plagioclase grain boundaries are rounded. These garnets are most likely newly grown, overprinting the existing continuous foliation.
Cordierite is rare and only observed in the western Aston dome north of the Etang d'Izourt (assemblage 5b, 98-124). There it forms centimeter-sized porphyroblasts with several inclusions of relic staurolite, fibrolite lenses, biotite, muscovite and ilmenite (Figure 11a). Abundant fibrolite and biotite is present in the matrix. Poikiloblastic, millimeter-seized anhedral andalusite is found at the rim of cordierite. A thin section from the same sample without cordierite shows abundant andalusite, fibrolite lenses replacing biotite, abundant biotite, but no muscovite or illmenite (Figure 11b). Regarding the growth of cordierite the following can be concluded from these observations. Cordierite and andalusite can be produced from the breakdown of staurolite:
Figure 11. Thin section photographs from a cordierite-bearing schist
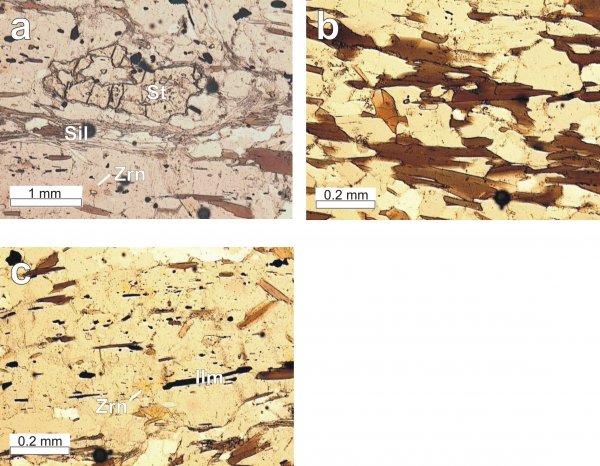
Thin section photographs from a cordierite-bearing schist (assemblage 5b, sample 98-124) of the western Aston dome.
Staurolite relic within cordierite, which covers the whole figure and contains inclusions of ilmenite (opaque phase). Embayed rims indicate that staurolite is being consumed. Note fibrolite needles nucleate in cordierite.
Section of the matrix at the same scale. Note the abundance of biotite and the absence of ilmenite.
Abundant ilmenite needles within cordierite result from released TiO2 during replacement of biotite by cordierite. PPL.
staurolite + quartz = cordierite + aluminosilicate + H2O
The presence of ilmenite as inclusions in cordierite, the small amount of biotite as inclusions compared to the matrix, suggests that biotite was consumed to produce cordierite (Figure 11c). TiO2 which is abundant in biotite, but is not incorporated into cordierite, nucleates as illmenite. A possible reaction is:
cordierite + muscovite = aluminosilicate + biotite + quartz + H2O
This reaction also produces muscovite, which occurs as inclusions in cordierite, but is missing in the cordierite-absent thin section. Though there is no direct textural evidence this reaction also involves the breakdown of andalusite.
The distribution of the metamorphic assemblages of the western Aston massif outlines a distinct increase of metamorphic grade towards the southeast. The three isograds that can be drawn with some reliability are the biotite-in, the sillimanite-in, and the cordierite-out isograds. Due to the paucity of data east and south of the Pic de Malcaras area, and west of Etang d'Izourt, the course of the sillimanite-in and staurolite-out isograds in these parts is presumptive. Verhoef et al. (1984) postulated a separate sillimanite isograd mantling the plutons in the Soulcem area, terming it the Soulcem thermal high. In contrast to their claims, this study has not recorded any sillimanite in the western Soulcem valley. Likewise, sillimanite-bearing rocks occur further to the southeast as shown by Verhoef et al. (1984). Future work will show if sillimanite extends east into northern Andorra up to the Aston gneiss, as it is implied in this study. The presently mapped metamorphic assemblages and the presence of pegmatite intrusions indicate the presence of heat source in that region.
The western Hospitalet dome is characterized by low grade muscovite-chlorite-biotite slate (assemblages 1b, 1c) from the French-Spanish border to el Serrat. The contrast is most evident at the Portella de Rialb, one kilometre south of the Pic du Port, where coarse grained sillimanite-biotite schist (assemblage 4b) is juxtaposed against fine grained slate along a steeply dipping shear zone, presumed to be the Merens fault. The grain sizes of the slates range from 50-200 µm, and a spaced cleavage is developed.
One kilometre further east andalusite and staurolite appear (samples 00-85, 00-99, assemblage 3b). This staurolite-andalusite zone forms a band approximately two kilometres wide and is bounded to the south, along the valley that extends eastward from el Serrat, by slate. It is not clear if the boundary is a structural break, i.e. shear zone. There is evident that it may be a metamorphic gradient. Sample 00-85, close to the mapped boundary, displays millimetre-sized staurolite and centimetre-sized andalusite porphyroblasts. Both phases contain quartz inclusions that preserve a crenulation folding and associated cleavage (Figure 12a). Andalusite also contain up to one millimetre-sized biotite porphyroblasts that overgrow the crenulation. The appearance and width of the crenulation is the same in staurolite and andalusite. Staurolite is also found as inclusion in andalusite, without any evidence for resorption. It is interesting to note that the matrix is a rather fine grained (50-200 µm) muscovite-chlorite-biotite schist (Figure 12b). These observations suggest that both staurolite and andalusite are part of the same paragenesis, being the result of the break down of chlorite and muscovite (reactions (2) and (3)), which also produced large biotite in the andalusite. Both phases statically overgrew an older crenulation cleavage, which since has been obliterated in the matrix. The matrix grain size, however, remained relatively fine. This changes further to the north in sample 00-99 (Figure 12c), which is a coarser grained andalusite-staurolite schist, similar in appearance to those in the western Aston dome. In this sample, staurolite and andalusite porphyroblasts are anhedral and poikiloblastic with straight inclusion trails, that are oriented parallel to the main schistosity in andalusite, and obliquely oriented in the staurolite. An older generation of biotite porphyroblasts, truncated by the main schistosity is present. Lacking any textural evidence for resorption, andalusite and staurolite appear to be of the same paragenesis, as in the previous sample.
Figure 12. Thin section photographs from the western Hospitalet dome
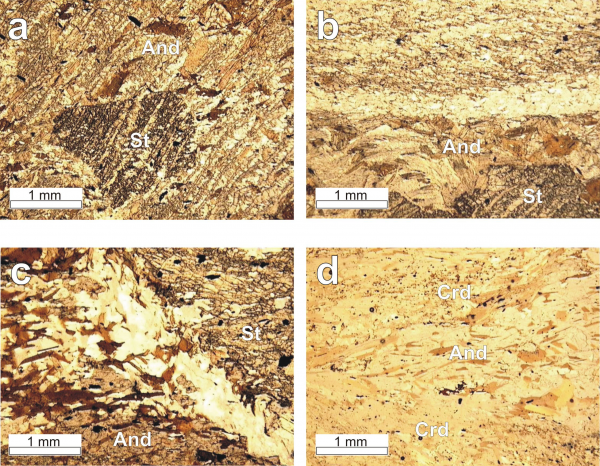
Subhedral staurolite porphyroblast found as inclusion in andalusite without signs of corrosion. Both phases overgrow the same crenulation cleavage, suggesting that both phases are part of the same paragenesis.
At the margin of the large andalusite blast the grain size difference to the matrix becomes evident. New biotite that formed along with andalusite is significantly larger than younger biotite in the matrix (assemblage 3b, sample 00-85).
A sample from the same assemblage approximately one kilometre further north displays a larger grain size of the matrix (sample 00-99). (d) Andalusite with embayed rims completely surrounded by cordierite. Note the presence of biotite in the andalusite, while cordierite contains abundant ilmenite inclusions. (assemblage 5a, sample 98-193). PPL.
The major difference compared to the western Aston dome is the negligible abundance of sillimanite. Cordierite on the other hand is more common, and occurs with staurolite, andalusite, sillimanite, biotite, muscovite, and garnet (assemblages 5a, 5b, 5c). The appearance is similar to the cordierite schist of the western Aston dome. Staurolite porphyroblasts are several millimetre in size, commonly rimmed by coarse new muscovite, cordierite forms large centimetre-sized porphyroblasts with abundant inclusions of ilmenite, and in places muscovite. Here, andalusite is found as an inclusion with embayed rim within cordierite, suggesting that andalusite was consumed to form cordierite (reaction 7), as postulated in section 4 c (Figure 12d).