Timing of K-feldspar megacryst growth in the TB: Experiments indicate that granodioritic magma becomes saturated in other minerals, such as hornblende, biotite and plagioclase, before K-feldspar [Piwinskii & Wyllie, 1970; Clemens & Wall, 1981]. Therefore, the K-feldspar in megacrystic granodiorites must have grown larger than the other minerals because of its much lower ratio of nucleation rate to growth rate in the magma concerned, not because it began to crystallize earlier than the other minerals [ Vernon , 1986]. Experiments have shown that in typical felsic magmas, about 60-70 per cent of the magma is still melt when K-feldspar nucleates [Clemens & Wall, 1981; Winkler & Schultes, 1982], and this provides enough room for the K-feldspar megacrysts to develop [Vernon, 1986]. The zonally arranged, much smaller inclusions of plagioclase and biotite (Figure 3a, Figure 3b, Figure 9d) reflect the much higher ratios of nucleation rate to growth rate of these minerals, which were crystallizing during growth of the K-feldspar. Field observations in the TB noted above indicate that many K-feldspar megacrysts had grown prior to being concentrated and/or aligned into general clusters (Figure 4), in dike-like bodies (Figure 5), schlieren troughs (Figure 6), schlieren tubes (Figure 7) and small plumes (Figure 8). Some megacryst plumes continued to move as diapirs (Figure 8a-d, Figure 10e) or by slumping along internal contacts within the chamber (Figure 4g, Figure 4h, Figure 6h). In some places K-feldspar megacrysts with fully developed zoning were either fractured (Figure 3b) or partly dissolved during contact melting (9b, 9d). Furthermore schlieren layering, defined by a modal increase of mafic minerals (Figure 9a, Figure 9b), may be deflected around megacrysts and/or concentrated around them requiring that the megacrysts already existed, in order to act as buttresses during the deflection.
All these structures are in turn overprinted by a magmatic foliation and steep lineation (Figure 2, Figure 10), which requires that at least many K-feldspar megacrysts had grown prior to the late formation of magmatic fabrics and final crystallization. We have also observed additional "cross-cutting" or relative timing relationships that place further constraints on the timing of K-feldspar megacryst growth in the Johnson granite, CP granodiorite, and HD granodiorite, respectively (Figure 10). Angular of the K-feldspar – bearing CP phase (Figure 10a) and individual K-feldspar megacrysts, presumably from the CP (10b), occur in the Johnson granite porphyry, which is typically fine grained and free of megacrysts . Thus megacrysts and/or megacryst-rich pieces of granodiorite existed before incorporation into the Johnson granite. Some schlieren tubes in the CP phase have K-feldspar megacrysts aligned parallel to the tube margins and are cut by felsic dikes that grade into leucocratic patches rich in K-feldspar megacrysts (Figure 10c). Both the tube and leucocratic patches are locally overprinted by a magmatic foliation. This indicates early alignment of K-feldspar megacrysts parallel to the trough walls, subsequent formation of K-feldspar – rich patches, and finally formation of the late magmatic foliation.
Figure 10. Timing relationships arranged from younger to older
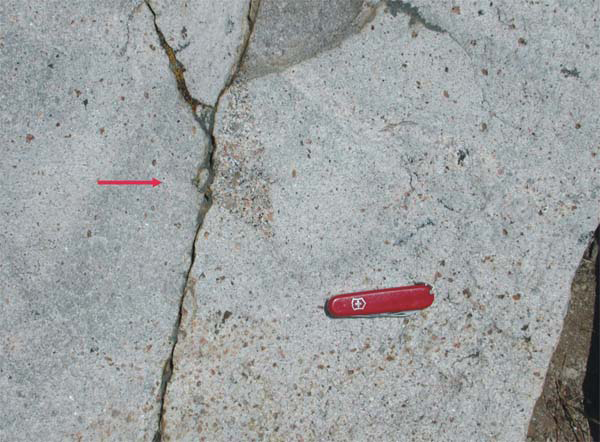
Timing relationships arranged from younger to older, that is from the central Johnson granite porphyry to HD granodiorite:
J 160: (UTM: 0291272 4194254) Angular xenolith (arrow) of CP phase in Johnson granite porphyry. The angular shape and truncation of K-feldspar grains indicate that this is a solid fragment of CP broken off after K-feldspar growth and transported into the Johnson granite. 8 cm knife for scale; (View).
J 160 (UTM: 0291272 4194254) K-feldspar megacrysts (arrows) that we interpret as having been transported from the CP granodiorite into the Johnson granite porphyry. 8 cm knife for scale; (View).
CP 111 (UTM: no data) Schlieren tube in CP phase with K-feldspar megacrysts aligned parallel to the tube margins. This tube is cut by felsic dike that grades into leucocratic patch rich in megacrysts. Both these features are overprinted by a magmatic foliation parallel to the 15 cm ruler; (View).
CP 281 (UTM: 0298025 4206295) An accumulation of K-feldspar megacrysts, some touching but with no molding, and of microgranitoid enclaves in a patch of CP granodiorite (possibly part of a former dike) surrounded by late aplite and intruding volcanic host rock along the eastern margin of the TB. Megacrysts indent or are wrapped by host enclaves (arrows). 36 cm hammer for scale; (View).
SM MZ 429 (UTM: 0298590 4203427) megacryst-rich dike of CP that has intruded magmatically folded schlieren layers, some with aligned megacrysts, and sheets of mingled HD and CP granodiorites. Dike was thickened and diapirically rose into layers and sheets. Our interpretation is that CP magma was more buoyant, and thus rose and deformed adjacent layers. 15 cm ruler for scale; (View).
HD-CP 460 (UTM: no data) Contact (marked with line) between HD and CP granodiorites. Microgranitoid enclave with K-feldspar megacryst occurs near mingled zone along contact. A magmatic foliation, parallel to the 15 cm ruler, cuts across contact and overprints the enclave; (View).
CP 738 (UTM: 0297500 4200149) K-feldspar crystal cut by aplite vein. ~4 cm chapstick for scale. Some minerals in enclav e are al so parallel to this foliation ; (h) CP SP kspar-aplite-2 (UTM: no data) K-feldspar megacrysts protruding into or transecting a late aplite dike. Dark rims on megacrysts were drawn with pen to emphasize crystal margins. 15 cm ruler for scale. (View).
Another example in the CP is the accumulation of K-feldspar megacrysts and megacryst-indented microgranitoid enclaves in a patch of CP granodiorite both of which are now in a late aplite dike that has intruded the volcanic host rock along the eastern margin of the TB (Figure 10d). This requires initial clustering of megacrysts and microgranitoid enclaves in the CP and then transport within the aplite dike. Along the contact between the CP and HD granodiorites, we have observed several other relationships. In a sheeted and mingling zone in the Sawmill Canyon area (Figure 2b) K-feldspar megacryst-rich dikes of the CP intruded into magmatically folded schlieren, some with aligned K-feldspar megacrysts, and sheets of mingled HD and CP granodiorites (Figure 10e). Some of these dikes began to thicken and diapirically intrude the surrounding layers and sheets. One interpretation is that the CP magma was more buoyant and thus rose and deformed adjacent layers.
Whatever the correct interpretation, it requires the alignment of megacrysts in the schlieren prior to diapiric rise of megacryst rich masses. We have also found microgranitoid enclaves with K-feldspar megacrysts in mingled zones along the HD-CP granodiorites contact: this contact and the enclaves are overprinted by a magmatic foliation (Figure 10f) as are other microgranitoid enclaves in the HD granodiorite. These observations imply early mingling of enclaves and K-feldspar megacrysts, juxtaposition of the CP-HD magmas, and finally overprinting by late strain prior to final crystallization. And finally, K-feldspar megacrysts are typically cut by late magmatic veins (Figure 10g). We are aware of only a few possible exceptions to the above observations and interpretations. For example, some megacrysts transect aplitic veins (Figure 10h).
However, aplites may form relatively early in the pluton history by fracturing of the incompletely crystallized host granite magma [e.g., Pitcher and Berger, 1972, p. 221; Hibbard & Watters, 1985 ]; if so, the megacrysts could have nucleated in either the vein magma or the host granite magma, or could have grown on existing K-feldspar crystals in either magma, and then continued to grow across the contact between both magmas [Vernon, 1986, p. 24]. Alternatively, if the aplite was formed late in the history of the pluton, this structure could represent continued growth of an existing K-feldspar megacryst into relatively late magma filling the vein [Vernon , 1986].
A similar explanation may account for dendritic aggregates of K-feldspar megacrysts transecting schlieren (Figure 3c,d); i.e., the megacrysts could have continued growing into magma with flow layering under conditions of strong supercooling. Evidence against concentration of megacrysts by in situ growth: Higgins (1991) suggested that concentrations of K-feldspar megacrysts in the TB granitoids are due to "Ostwald ripening”, which he inferred to have dissolved existing K-feldspar crystals, making flow channels available for K-rich melt to precipitate and therefore concentrate K-feldspar megacrysts by in situ growth in the channels. An alternative explanation is that interstitial melt has been removed, passively concentrating the megacrysts — i.e., a "log jamb” situation (e.g., Clarke & Clarke 1998; Weinberg et al. 2001). Moreover, the evidence discussed in the previous sections indicates that K-feldspar megacrysts in granodiorites commonly move as large independent crystals and concentrate mechanically, rather than being the result of crystallization in situ.
As noted by Gilbert (1906, p. 322) and Wilshire (1969, 244), the K-feldspar megacrysts typically do not interpenetrate (Figure 3a, Figure 3b, Figure 4a-e, Figure 6g, Figure 9b, Figure 9d, Figure 10d), even where in contact, but almost always remain separate in the concentrating process; simultaneous, in situ growth of K-feldspar crystals would be likely to produce molding of one megacryst around another. Where local contact does occur, compositional zoning is truncated (Figure 9b, Figure 9d), which can indicate contact melting [Park & Means 1996]. Furthermore, K-feldspar megacrysts in the TB show normal zoning in Ba [Kerrick, 1990], as do many K-feldspar megacrysts [Vernon , 1986].
In contrast, dissolving early-formed small crystals should add progressively more Ba to the larger crystals though the effect may be minor, in view of the probable small size of the crystals inferred to have been dissolved. In a few places, the most abundant, aligned K-feldspar megacrysts occur in the most mafic (hornblende-rich) schlieren (Figure 6, Figure 7), even around folded layers, indicating hydraulic equivalence between the two minerals at the sizes concerned. The model of Higgins (1999) produces layers rich in K-feldspar, not hornblende. Moreover, if the megacrysts were formed in situ from percolating K-rich melt, there would be little constraint on them to grow with such a strong parallel alignment. In addition, a K-rich granitic melt would also be expected to precipitate some biotite, plagioclase and quartz with the K-feldspar.
It is also difficult to explain magmatic fabric characteristics by the Higgins model. We infer that the slightly younger magmatic foliation /lineation, which cuts contacts between intrusive units in the TB (Figure 2), recorded strain increments superimposed on a relatively static chamber [Paterson et al., 2003]. In the CP granodiorite and the Johnson granite porphyry, this regional magmatic foliation /lineation is in places defined by K-feldspar megacrysts , suggesting that they were present in the magma prior to fabric formation, and subsequently have been reoriented due to regional strain while a melt was still present in the system.
A final argument is that mafic schlieren (Figure 6, Figure 9a-b) and microgranitoid enclaves (Figure 10d) commonly are indented by K-feldspar megacrysts. In the Higgins (1999) model, the megacrysts would have to physically push aside the mafic layer or enclave during their growth. Below we suggest an alternative interpretation that extraction of melt occurred , leading to collapse of the mafic layer or enclave around the strong megacrysts.
Types of processes that physically moved/accumulated K-feldspar megacrysts: In an earlier section we noted structures associated with the physical movement and/or accumulation of K-feldspar megacrysts in the TB (also see Gilbert 1906). We now speculate on the processes that are implied by these structures/accumulations.
Where K-feldspar megacrysts are concentrated, the magma between the megacrysts must have been preferentially removed. We infer this to have taken place by a "filter-pressing” mechanism [ e.g., Kerr and Tait 1986; Park and Means 1996; Weibe 1996; Clarke & Clarke 1998; Weinberg et al. 2001]. We suggest that the fracturing (Figure 3a, b) and contact melting (Figure 9a, d) of K-feldspar megacrysts in clusters imply that zoned megacrysts grew, and then were physically accumulated during removal of magma, during which some impinged on one another, possibly causing the cracking or contact melting. The "pressing” was driven by buoyancy, but the details varies from place to place. For example, we interpret the deflection of schlieren layering around megacrysts (Figure 6c, 6d, 9a, 9b, 9c) as a result of filter pressing driven by sinking of the overlying layer, which would increase the proportion of the mafic minerals (as the more felsic melts rise). Alternatively, megacryst-rich plumes may rise and cause filter pressing on their upper surfaces along which concentrations of mafic minerals form (Figure 6a, 8a, 8b, 10e). Concentrations of megacrysts in dikes (Figure 5) may partly result from melts draining off as flow in the dikes slow and the dike wall collapse inwards.
Imbrication (tiling) of megacrysts presumably occurred during magmatic strain caused by flow [Blumenfeld, 1983; Paterson et al., 1989; Paterson et al. 1998; Vernon 2000], leading to concentration of megacrysts as they piled up behind obstacles or more slowly moving megacrysts (Figure 4b, 4c, 4e, 9d). Again the magma between the megacrysts must be "removed” and thus move at a faster velocity than the megacrysts [e.g., Koide and Bhattacharji 1975; Bergantz 2000].
The general clusters preserve little evidence of how they formed. They typically are not associated with preserved evidence of flow [e.g., schlieren layers, boundaries, etc] and occur in a variety of different settings in the chamber. Only rarely are they associated with dikes indicating that melt drained off (Figure 10c) . However we think it likely that these clusters reflect regions where K-feldspar megacrysts "piled up” during general flow in combination with removal of the magma between crystals as described above.
We interpret schlieren troughs, including grading in the schlieren and cross-cutting of one trough by another (Figure 6), to be equivalent to sedimentary troughs and grading and thus to reflect local magma flow channels [e.g., Barrière 1981; Bateman 1992]. The scattered to locally concentrated K-feldspar megacrys ts, along with mafic minerals and microgranitoid enclaves in the trough-bounding schlieren layers (Figure 6, 7) indicate hydraulic equivalence of these objects. Koide and Bhattacharji (1975) and Weinberg et al. (2001) ascribed this kind of layering to shear sortin g, and Barrière (1981) discussed possible boundary effects that lead to sorting of minerals during such shear flow .
The "schlieren tubes" (Figure 7) and diapirically rising "plumes” (Figure 6a, 8, 10e) are interpreted by Weinberg et al. (2001) to form by compositional and thermal instabilities that drive local flow in closed channels through a crystal mush. Our observations strongly support their interpretations and add some additional information. Almost all tube and plume axes plunge greater that 70 o in the TB suggesting gravity played a dominant role in their formation. The rare exceptions typically have evidence that the tubes/plumes "fell over" and collapsed into the surrounding magma. The tubes typically cut through older layering (if present), and in a few places we have observed their 3D forms (Figure 7b, 7c). Some of the plumes consist of up to 80% modal percent of K-feldspar megacrysts (Figure 8) indicating that liquid was removed from the plume by a "filter pressing" mechanism. Others distort overlying schlieren layers (Figure 6a, Figure 10e) and have concentrations of mafic minerals along their upper surfaces (Figure 8a, b), again suggesting a filter pressing mechanism during rise of the plumes. We suggest that shear-flow sorting and filter pressing mechanisms led to the K-feldspar concentrations, which in turn led to additional compositional buoyancy driving further upward motion. Both tubes and plumes are overprinted by a magmatic fa bric, indicating that these features represent local flow before the magma reached its solidus.
Megacryst concentrations in dike-like bodies with diffuse (Figure 5q) or sharp (Figure 5c, d) boundaries may reflect different processes. We interpret bodies with sharp boundaries as true dikes in which magma was flowing along a crack formed in a crystal-rich magma. Some of these dikes extend at least 100s meters suggesting that magma viscosities in the dike were not high and thus that the magma in the dikes originally had a greater proportion of melt and/or was volatile-rich. In these dikes, liquid was driven off into megacryst poor veins, by filter pressing through the dike walls, or by flow along the dike as the dikes cooled, collapsed and concentrated the megacrysts. However, we cannot determine to what degree megacrysts were concentrated before entering the dikes as opposed to concentration of megacrysts by loss of liquid during late freezing and shrinking of the dikes. Dike-like megacryst concentrations with diffuse boundaries (Figure 5a) may represent zones of local "filter pressing” and megacryst accumulation , or may be former planar dikes in a crystal mush, the dikes having been deformed by continued flow of the mush. may rise into surrounding materials by thermal or compositional buoyancy-driven motion (Figure 6a, 6h, 10e).
We are aware of three possible interpretations of the megacryst concentrations derived from the CP phase that now reside in the megacryst-free outer HD phase (Figure 4h): (a) previous accumulations slumped off a reasonably crystallized CP margin into the HD magma; (b) previous accumulations along a margin between two magmas diapirically rose into the HD magma; and (c) magma mingling occurred between the two magmas driven by flow along the margin [e.g., Bergantz 2000] . At present the CP is considered to be younger than the HD [e.g., Coleman et al. 2004] , and we thus favor one of the latter two interpretations.
The introduction of K-feldspar megacrysts into microgranitoid enclave magmas by magma mixing (commonly before the formation of enclaves by magma mingling) implies the existence of separate megacrysts at a relatively early stage of the history of the host magma. Arguments in favor of magma mixing, rather than in situ growth of K-feldspar megacrysts in enclaves have been discussed by Reid et al. (1983) and Vernon (1983, 1986, 1990, 1991).
The alignment of megacrysts reflect late strain in the chamber which realigned already existing K-feldspar megacrysts and possibly brought them together during strain as interstitial melt was driven off.