The serpentinites represent about 40% of the Monviso ophiolitic massif (e.g., Lagabrielle 1987, Blake et al. 1995, Schwartz et al. 2001) (Fig. 5). Serpentinites are particularly well exposed in the Lago Superiore, Passo Gallarino and Viso Mozzo units. The intimate association of serpentinites with the eclogites from cm to cartographic scale suggest that they shared similar metamorphic evolution. The orientation and the sense of shear observed in the serpentinite matrix are similar to those observed in the surrounding metabasites: top to the west under greenschist facies metamorphic conditions (Schwartz et al. 2000, 2001).
The serpentinites observed in the Monviso unit are mostly hydrated close to 100% and few samples show relict diopside grains which are highly chloritized and deformed. Serpentinites show penetrative foliation with locally pseudomorphic textures. Pseudomorphic structures are observed with relict magnetites that still underline early mesh boundaries whereas former pyroxenes (bastites) correspond to magnetite-free areas (Fig. 8). The core of meshes or bastites recrystallized at high grade with interpenetrative serpentine blades (Fig. 8). Textural evidences coupled to a micro-Raman spectroscopic study show that antigorite is the main variety, as expected from its stability under high pressures (Auzende et al. 2005). Antigorite grains show no apparent compositional variation with low Al2O3 and Fe2O3 (<2 wt%). Lizardite and chrysotile form secondary veins during retrogression.
Figure 8. Photo micrographs
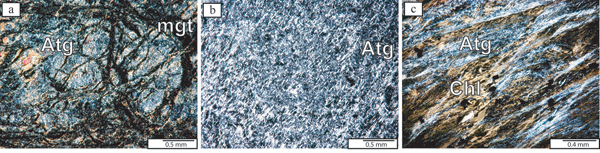
Photomicrographs of serpentinites from Monviso under cross-polarized light after Auzende et al. (2005). a) Interpenetrative antigorite surrounded by fine grained magnetite that outlines the pseudomorph of olivine (Sample 624-2: Viso Mozzo), b) Typical antigorite interpenetrative texture in metabasalt? (Sample 623-3: Lago Superiore), c) Sheared serpentinite where antigorite and chlorite (Chl) define the foliation and post-kinematic chlorite crystals cut the foliation (Sample 625-6: Lago Superiore).
The Microstructure of antigorite was investigated by electron imaging coupled to SAED with a transmission electron microscope (Auzende et al. 2005). The results show that antigorite crystals display similar texture and compositions. However, all studied SAED patterns show sharp modulation diffraction spots without streaking in the a* direction (Fig. 9). The evidence suggests intracrystalline polysomatic regularity in Monviso samples with the constant A dimension (modulation wavelength). This regularity is also apparent on TEM images, showing a highly ordered structure along a. Nevertheless, while the modulation length is constant in each crystal, this dimension significantly varies from one antigorite grain to one another within the same TEM preparation.
Figure 9. Electron micrographs
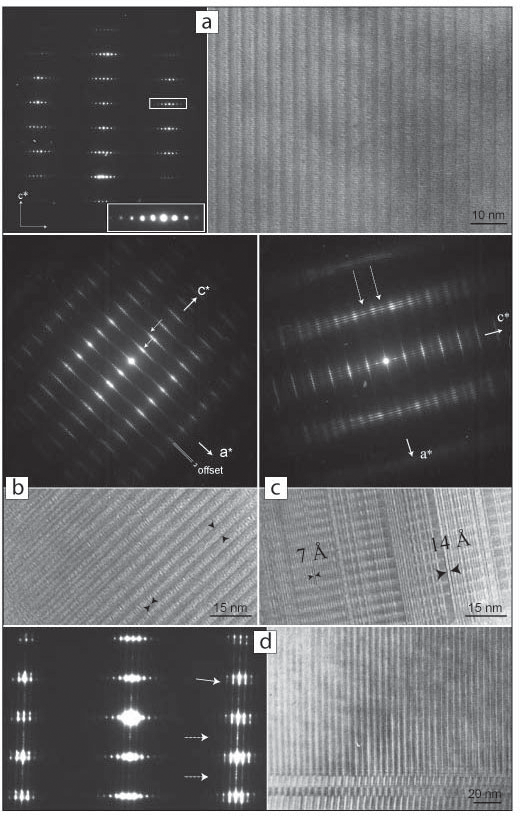
Electron micrographs and SAED patterns of antigorite crystals from Monviso oriented along [010] direction (after Auzende et al. 2005). a) Highly ordered structure along a* and c*, b) Offset structure. The offset is the deviation between the alignment of satellites around a (h00) sublattice spot and that of the neighbouring (h00) group (Passo Gallarino, 624-7), c) Stacking variations, alternating 1- and 2-layer polytypes. The dashed arrows in the SAED pattern point at the intensity reinforcement due to the double periodicity along c*. Besides the polytypic variations, the pattern shows diffuse streaks which affect the lattice and modulation spots along c*, indicating the loss of periodicity due to planar faults (Passo Gallarino, 624-7), d) Streaking along c* indicates stacking disorder. The white arrow shows spot rotation due to twinning and dotted white arrows indicate supplementary diffraction spots with 14.6 Å periodicity showing 2-layer polytypism (Monviso, Lago Superiore, 623-3).
The modulation wavelength is commonly expressed by the m-value, as the number of tetrahedra in one modulation. According to our data from SAED patterns of the 96 antigorite crystals oriented along [010], antigorites from Monviso have m-values varying between 16 and 20 (41 to 52 Å), with a dominance of crystals with m = 18 (46 Å) and 19 (49 Å) (Fig. 9). The average m-values for the three units are close considering their standard deviation.
+ (exhumation timing) Timing and mechanism of exhumation
As previously mentioned, the Monviso unit is generally considered as a fragment of the Upper Jurassic Tethyan oceanic lithosphere, subducted at the Paleocene-Eocene boundary (Lagabrielle and Cannat 1990). However, the eclogites show metamorphic pressures varying from 1.2 GPa to 2.4 GPa and independent of the size of blocks from decimetres to kilometres (Messiga et al. 1999, Schwartz et al. 2000, Castelli et al. 2002). This suggests that the Monviso massif does not represent a continuous fragment of the Tethyan lithosphere. Instead, it is an assemblage of tectonically juxtaposed slices that were subducted to different depths. Furthermore, PT conditions of different units do not show any logical arrangement (Fig. 6), suggesting that this tectonic juxtaposition was unlikely to have taken place during the exhumation. Finally, the basal unit records variable P-T conditions from a minimum of 1.3 GPa and 470 °C to 2.0 GPa and 575°C (Blake et al. 1995, Schwartz et al. 2000, Castelli et al. 2002). The evidence collectively suggests that the Monviso is a tectono-metamorphic melange with variable eclogitic blocks in a serpentinite matrix. Our proposed interpretation is supported by numerical modelling by Gerya et al. (2002) and Stöckert and Gerya (2005) which showed that a subduction channel with low viscosity (~ 1019 Pa.s) favoured the formation of a large melange. If the Monviso massif represents the exhumed part of a subduction channel, the varied ages of eclogites between 61 ± 10 Ma and 45 ± 1 Ma may be related to the different blocking temperatures of isotope systems. This is a long standing problem in metamorphic rocks (Monié and Chopin 1991, Arnaud and Kelley 1995, Rubatto and Hermann 2003). Alternatively, these ages may signify different events in the serpentinized channel. This may be supported by the fact that these ages do not overlap each other. Thus, we favour the latter interpretation. In this case, it gives a duration for operation of the serpentinized channel of 5 to 15 Ma.
The exhumation of rocks requires a mechanically weak zone at the interface between the subducting plate and overlying mantle peridotites (Cloos 1982, Closs and Schreeve 1989, Platt 1993, Allemand and Lardeaux 1997). At the depths greater than 40-50 km, hydrated peridotites can play the role of the lubricant (Hermann et al. 2000, Guillot et al. 2000, Gerya et al. 2002). The exhumation of dense eclogitic blocks (>3500 kg.m3) in a buoyant serpentinite matrix (<2700 kg.m3) requires a dynamic return flow (corner flow). Assuming a Newtonian rheology of serpentinites in subduction conditions (Gerya and Stöckert 2002, Auzende et al. 2005), the velocity and the size of the exhumed eclogitic blocks are controlled by Stoke’s law (Cloos 1982). In our previous paper (Schwartz et al. 2001) we have numerically evaluated the possibility that the eclogitic blocks similar to those in the Monviso can be exhumed in a low viscosity channel (Fig. 10). The exhumation of decimetric to kilometric eclogitic blocks at a velocity of one cm/yr requires the low viscosity channel of about 10 km width and a minimum viscosity of 1020 Pa.s. The viscosity is similar to the result obtained by Guillot et al. (2001) and Stöckert and Gerya (2005). The viscosity imposes that the channel is composed of partially (up to 50%) hydrated peridotites.
Our proposed interpretation is supported by the geophysical evidence for a 10 km thick hydrated peridotites layer along Pacific subduction zones (Bostock et al. 2002, Billen and Gurnis 2003, Seno and Yamasami 2003). The cross section of the Monviso (Fig. 4) suggests that the serpentinite subduction channel had a minimum thickness of 4 km. The total length of this channel is difficult to estimate, but may be 40 to 50 km long if the Monviso massif extends beneath the Dora Maira massif and has a depth of 10 km on its western part (Paul et al. 2001) (Fig. 4). The first assumption is supported by geophysical data farther north of the study area (Escher et al. 1997).
Figure 10. Schematic evolution
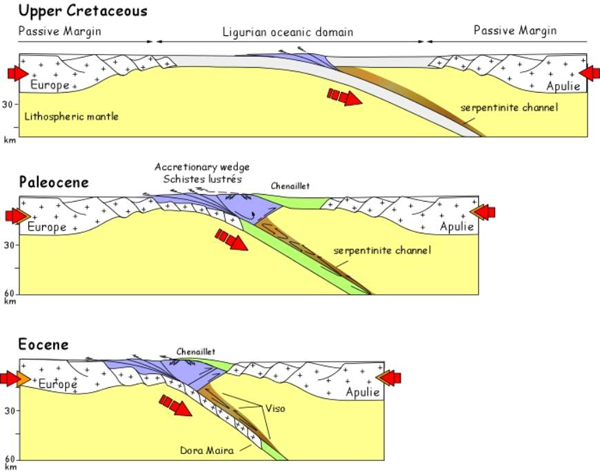
Schematic evolution of the Piedmont subduction zone from Upper Cretaceous to Eocene. The Schistes Lustres accretionary wedge is separated from the serpentinite channel overlying the subduction zone. Note that the serpentine channel records internal motions during the Paleocene, whereas, the initiation of the subdcution of the Continental European margin in Eocene time stopped the activity inside the subduction channel, leading to the final exhumation of the Monviso eclogitic blocks.
The length of the subduction channel is estimated using the PT conditions of eclogitic fragments in the Monviso massif. If the massif is a tectonic melange, different eclogitic blocks record the pressures of the subduction channel. The pressure difference of 1.2 GPa between the highest pressure unit (2.4 GPa, Lago Superior) and the lowest pressure unit (1.2 GPa, Passo Galarino) corresponds to a depth difference of ~ 40 km.
A question remains in respect to the relationship between the southeast dipping subduction of the European continent margin and the exhumation of the eclogitized oceanic crust (e.g. Agard et al. 2002 for a complete discussion). Polino et al. (1990) and Allemand and Lardeaux (1997) proposed that the burial and the subsequent exhumation of the oceanic plate occurred only during active subduction whereas other authors proposed that the main exhumation of the oceanic plate occurred when the buoyant continental margin subducted (Chemenda et al. 1996, Ernst 2001). In contrast, Rosenbaum and Lister (2005) proposed a collisional event at 45 Ma.
The relationship between the exhumation and continental subduction is clear north of the study area along a Gran Paradiso Zermatt-Saas transect. The HP metamorphism in Zermatt-Saas ophiolite is dated at 40.6 ± 0.6 Ma by Sm-Nd isotopes whereas the HP metamorphism of the European continent at the Gran Paradiso massif is estimated to have occurred at a 43.0 ± 0.5 Ma (Rb-Sr isochron based on microsampling). The data suggest that HP metamorphism was synchronous. Stöckhert and Gerya (2005) also show by numerical simulation that the peak of metamorphism in the Internal Alps is probably contemporaneous for continental and oceanic units, 10 to 20 Ma after the initial subduction of the European continent. In the Monviso area, the relationship between oceanic and continental subdution is not so clear. In fact, the HP metamorphism in the Monviso ophiolite occurred between 60 and 45 Ma whereas the UHP metamorphism in the nearby continental unit of Dora Maira is dated between 31 and 35 Ma (Duchêne et al. 1997, Gebauer et al. 1997, Cliff et al. 1998, Rubatto and Hermann 2001). We can assume than the subduction and subsequent exhumation of the Monviso massif occurred before the continental subduction of the Dora Maira continental massif (Fig. 10). However, a low convergence rate, about 6-8 mm.yr-1, of the Western Tethys from Late Cretaceous to Eocene (Facenna et al. 2001, Schettino and Scotese 2002) suggests a minimum of 17 Ma for the time required for the Dora Maira massif to reach the depth of 120 km for the UHP metamorphic conditions. This implies that the continental subduction initiated before 45-50 Ma in the studied area, i.e. at the time of the HP metamorphism in the Monviso unit. Thus we conclude that the exhumation of the Monviso massif is broadly contemporaneous with the continental subduction of the Dora Maira massif (Fig. 10).