5 South Tibetan detachment system
For the final portion of the field trip we travel to northern Sikkim to examine a spectacularly exposed section of the South Tibetan detachment system (STDS) in northern Sikkim (Fig. 1.1). We will focus on the structurally uppermost GHS rocks, which are deformed by the detachment system north of the village of Thanggu, near the India-Tibet border. These rocks preserve a history of Tertiary metamorphism and partial melting, deformation, and exhumation in the footwall of a large scale, orogen-parallel detachment.
The STDS is a set of low-angle normal-sense structures that cuts through some of the highest peaks of the Himalayan orogen, including Dhaulagiri, Annapurna, and Everest (Chomolungma), to name a few, and separates high-grade metamorphic rocks of the GHS in its footwall from low grade metamorphic to sedimentary rocks of the Tethyan sedimentary sequence (TSS) in its hanging wall. The detachment system is situated along strike of the crest of the range, where the steep, deeply-incised southern front of the Himalayan mountain chain meets the broad, undulating high-altitude hills of the Tibetan plateau (Fig. 1.1).
The formation of normal-sense structures like the STDS which seem to indicate slip occurred at low angles (≤30◦ dip) is in apparent contradiction with Anderson’s theory of basic fault mechanics, which predicts that normal-sense structures will form at relatively high (~60◦) dip angles, while low-angle structures should be thrust sense (Anderson, 1951). Partly for this reason, geologists did not recognize low-angle normal sense detachments (LANDs, also sometimes referred to as LANFs - low-angle normal faults) in the field until the 1970’s and 1980’s (e.g., Armstrong, 1972; Davis and Coney, 1979; Wernicke and Burchfiel, 1982). LANDs are now known to be common structures, and have been mapped in several tectonic environments (e.g., extensional provinces such as the Basin and Range, metamorphic core complexes such as those in the Cordillera, slow-spreading mid-ocean ridges such as the Mid- Atlantic Ridge, and active collisional orogens such as the Himalaya). LANDs from these different tectonic settings share many common features, including: low (≤30◦) dip angles; a contrast in structural style above and below the detachment; pooling of magma in the footwall at the detachment surface (often attributed to decompression melting of footwall rocks as they are denuded and resulting in a thermal and rheological contrast across the detachment); and, the juxtaposition of high-grade metamorphic rocks exhumed from the middle or lower crust in the footwall against unmetamorphosed or low-grade upper crustal rocks in the hanging wall. Some of the first studies on LANDs, in metamorphic core complexes of the Basin and Range extensional province of the southwest USA and the North American Cordillera, pointed to an exclusively extensional tectonic setting for their formation. The STDS was the first LAND discovered to have accommodated lithospheric contraction and remains the best studied worldwide, although to date, kinematically similar structures have been identified in other active and ancient orogens including: Pamir (e.g., Brunel et al., 1994); Canadian Cordillera (e.g., Carr et al., 1987; Brown and Gibson, 2006), and Hellenides (e.g., Xypolias and Kokkalas, 2006; Ring et al., 2007).
The STDS was first described by Burg et al. (1984) as a normal fault that deformed leucogranites at the top of the GHS metamorphic package, and that in places juxtaposed low-grade metamorphosed Jurassic schists against staurolite-kyanite gneiss. Investigating crystallographic preferred orientations in quartz crystals within leucogranites in southern Tibet east of Bhutan, Burg et al. (1984) demonstrated that the upper boundary of the GHS was a shear zone of gently- dipping mylonite gneiss hundreds of metres thick that had an opposite shear sense to the regional thrusting. They further demonstrated that shearing post-dated emplacement of the leucogranites, which at that time were already suspected to be produced from partial melting within the GHS above the MCT. This timing relationship demonstrated that the normal-sense structure was likely Miocene in age, and thus coeval to either the MCT or the MBT. Burg and colleagues noted the apparent incongruity of a north-directed normal-sense ductile shear zone within the south- directed thrust-sense Himalayan orogen, and explained its formation by sliding of the TSS along a gravity-driven décollement (Burg et al., 1984).
Burchfiel and Royden (1985), noting the along-strike continuity of the structure, further suggested that it may have accommodated as much as tens of kilometres of extensional northward displacement of the TSS, and probably formed after development of the TSS fold and thrust belt and coevally with leucogranite intrusion and thrusting on the MCT or MBT. It was later confirmed that the STDS and the MCT were indeed coevally active (Hodges et al., 1992), and that the STDS extends west as far as NW India (Herren, 1987; Kündig, 1988).
5.1 The STDS in Sikkim
In Sikkim, rocks deformed by the STDS are accessible along its northern border at intermediate altitudes (4000-5500 m above sea level) by road or trekking trails. The contrast in metamorphic grade and hence compentency of rocks in the hanging wall and footwall of the STDS is marked, and the arid Tibetan plateau is sparsely vegetated, such that the STDS trace can be observed relatively clearly from satellite imagery (Fig. 5.1). It can be seen in Fig. 5.1 that although the STDS is regionally an east-west striking and north-dipping structure, the detachment system locally dips towards NE in Sikkim, and is offset by a small, north-south striking extensional fault, apparently synthetic to major NS-striking structures that form late graben systems such as the Yadong graben (SE corner of Fig. 5.1) across the Himalayan chain and are seismically active (Pradhan et al., 2013).
Figure 5.1. Digital elevation model of northern Sikkim
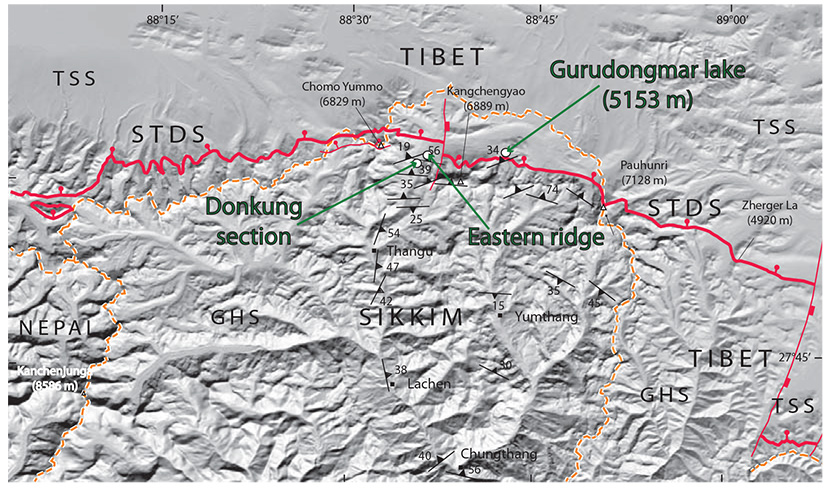
Digital elevation model of northern Sikkim showing the trace of the STDS, as well as main foliation of the upper GHS, modified from Kellett et al. (2013)
The road which today travels through Thangu wraps around to the east once north of the Himalayan range, to provide access to Gurudongmar Lake, one of the highest lakes in the world (5148 m) and a sacred pilgrimage route for Buddhists, Sikhs and Hindus alike. The first stop for this section of the virtual field trip is near Dongkung, along this road north of Thangu (Fig. 1.1, Table 5.1) where GHS rocks are deformed in the footwall of the STDS. Note that an “inner line permit” is needed to access the areas to the north of Thangu.
Table 5.1. Virtual field trip stops for South Tibetan detachment system
Locality | Lat | Long | Description | |
---|---|---|---|---|
5.1 | Dongkung section | 28.01477 | 88.59277 | transect across base of STDS |
5.2 | N of Dongkung section | 28.02326 | 88.59514 | Large-scale cross-sectional views of STDS, Tethyan Himalaya hanging wall and GHS footwall |
5.3 | Eastern ridge | 28.01139 | 88.6042 | Northernmost outcrop of STDS |
5.4 | Gurudongmar Lake | 28.03472 | 88.70833 | float and outcrop in the uppermost footwall of the STDS |
5.2 Dongkung section (N 28.01477˚, E 88.59277˚)
The Dongkung transect is located north of Thanggu, and west of the road leading to Gurudongmar Lake (Figs. 5.1, 5.2, Table 5.1). Dongkung itself comprises a group of pastoral structures north of the geological section. Outcrop is relatively continuous along a gully. The GHS at this site comprises variably migmatitic, locally mylonitic metapelitic (Fig. 5.3a, b) and augen (Fig. 5.4a) gneiss with local quartzite layers and mafic lenses (Fig. 5.4b). Leucosomes suggest in situ partial melting (e.g. Fig. 5.3a,b), while abundant cross-cutting leucogranite dykes (locally aplitic) (e.g. Fig. 5.4a) indicate transported melt, likely derived from
Figure 5.2. Google Earth image looking south down the valley towards Thangu (out of view)
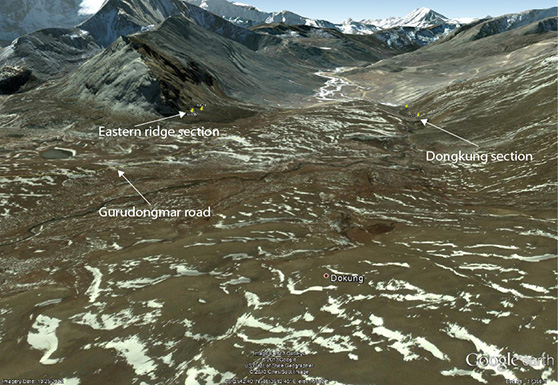
This view shows the locations of the Dongkung and Eastern ridge sections on either side of the road to Gurudongmar Lake, both of which record STDS deformation
Figure 5.3. Biotite-garnet-sillimanite migmatite gneiss
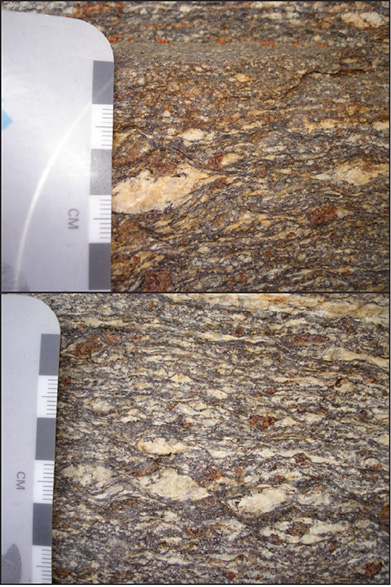
Shear bands cut leucosomes and indicate top-down-to-north (right) shear sense. View is towards west in both photographs
The main fabric in the rocks dips to NE north of the southernmost outcrops (N 28.014777, E 88.592767, ~4730 m). The dominant shear sense transitions from top-up-to-south to top-down- to-northeast with pervasive top-northeast shear sense indicators observable from 28.016050, 88.592817 and continuing northward. Sillimanite and quartz define mineral stretching lineations that on average plunge shallowly towards NNE (Fig. 5.5a) while biotite and fine gneissic banding defines the main foliation in gneiss (Fig. 5.5a) and mylonite (Fig. 5.5b). Discrete brittle fault surfaces coated in chlorite slickenfibres exhibit minor displacement and are parallel to stretching lineations. Top-down-to-northeast shear sense indicators in outcrop include shear bands (Fig. 5.2a), -type K-feldspar porphyroclasts, including large -type structures formed around mafic lenses (Fig. 5.4d), bookshelf sliding in mafic lenses (Fig. 5.3b), and weak S-C fabric. Crystallographic preferred orientation fabrics in quartz-rich metapelitic gneiss record well-defined type II crossed girdle fabrics (Fig. 5.6). This implies that dynamic crystallization of quartz was occurring during deformation, consistent with quartz defining the mineral stretching lineation. Moreover, these type II fabrics may indicate constrictional strain (Bouchez, 1978; Schmid and Casey, 1986). With the exception of SK63, the fabrics are broadly symmetrical and cannot be used to determine shear sense. SK63, however, shows a strong asymmetry indicating a top-up-to-the-south sense shear. Such a shear sense is antithetic to the dominant observed direction, however, SK63 was collected from a localized region of asymmetric folding in which the dominant regional mylonitic fabric was not developed. The shear sense recorded by this sample may thus preserve the relict deformation fabric that predates the main STDS mylonite fabric regionally preserved in the area, indicating that top-north deformation overprints an earlier top-south deformation.
Figure 5.4. Augen gneiss, pressure shadows, bookshelf sliding and asymmetric mafic boudin
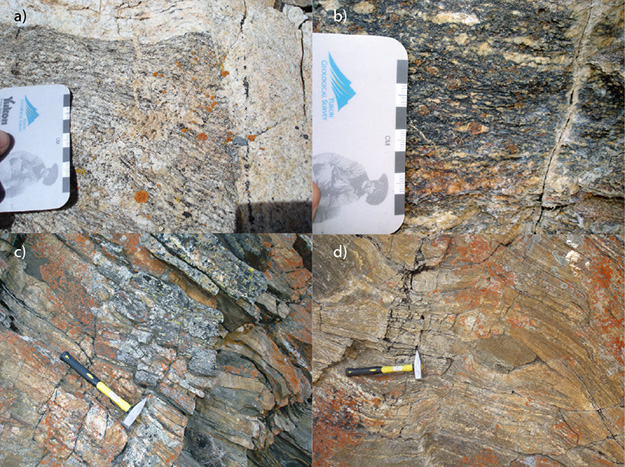
a) Migmatite augen gneiss with large concordant leucosome at top of photo, cut at a high angle by a relatively undeformed leucogranite dyke. b) Pressure shadows around garnet grains in mylonite gneiss. c) Bookshelf sliding of blocks in fractured mafic lens. Hammer handle is ~30 cm. d) Asymmetric mafic boudin hosted by migmatite gneiss. View is towards west in all photographs
Figure 5.5. Equal area stereonets displaying structural measurements
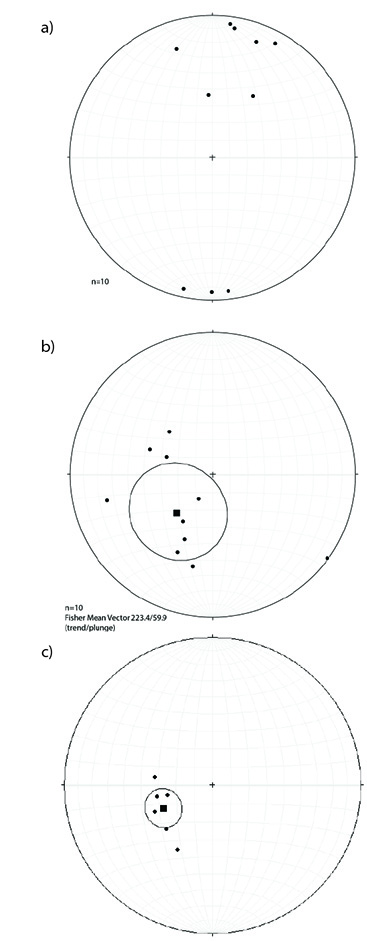
a) Stretching lineations at Dongkung section predominantly dip shallowly towards NNE. b) Poles to foliation planes indicate that main foliation dips moderately towards NE, while mylonitic fabric c) dips somewhat more shallowly towards ENE. Stereonets plotted using Stereonet v.7.2.1 by R. Allmendinger
Leucogranite dykes show little internal deformation in outcrop, but in thin section some of them exhibit quartz grain size reduction and subgrain rotation recrystallization, deformation twins in plagioclase, myrmekite, tartan twins in K-feldspar, and a preferred orientation of biotite and muscovite, indicating they have experienced subsolidus ductile deformation at relatively high temperatures (Kellett et al., 2013).
Both outcrop and thin section observations indicate that STDS deformation occurred subsequently to peak temperature metamorphism under sillimanite-stable conditions. Shear bands wrap around garnet grains, leucosomes record subsolidus deformation and are cut by shear bands (e.g. Fig. 5.2 a,b), and fibrolite is typically associated with shear bands. These observations are corroborated by in situ Th-Pb monazite geochronology of gneisses (e.g. SK63 of Fig. 5.6). Monazite included in garnet are ≥23.4 Ma, while monazite associated with shear bands in the matrix are as young as 14.6 Ma (Kellett et al., 2013). Zircon rims from cross-cutting and weakly-deformed leucogranite dykes also indicate crystallization until ca. 14 Ma (U-Pb ages) at >700 °C (Ti-in-zircon). These data together suggest that the footwall of the STDS was deformed by top-down-to-the-north shear between 23.6-14 Ma. Near identical ages for last zircon growth, last monazite growth, retention of Ar in muscovite and retention of fission tracks and He in apatite illustrate the transient rapid cooling of this section of the STDS during Middle Miocene (Kellett et al., 2013).
Figure 5.6. Crystallographic preferred orientations
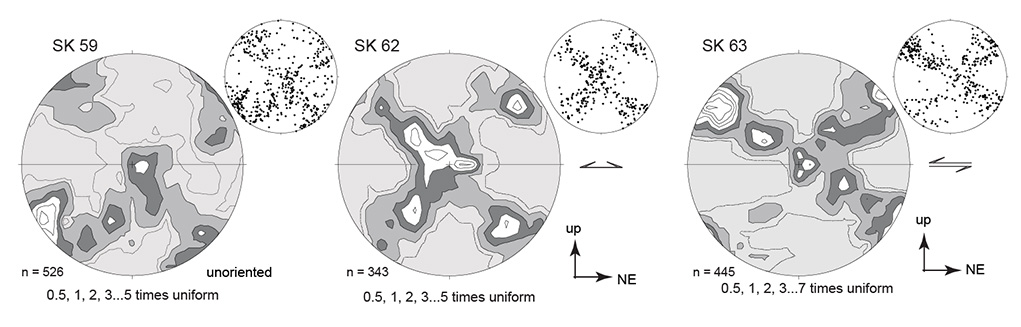
Crystallographic preferred orientations of quartz in quartz-rich GHS metapelitic gneiss from the Dongkung section illustrating the non-coaxiality of deformation. SK 62 is a garnet-biotite-sillimanite (in order of increasing abundance) gneiss with fibrolite occurring in shear bands, myrmekite, dominantly grain boundary migration recrystallization with local subgrain rotation recrystallization in quartz, biotite+quartz+sillimanite strain shadows around pervasively fractured garnets. Internal fabric in garnets is at a high angle to the gneissic fabric. Accessory monazite in this sample yielded Th-Pb ages ranging ~32-14.6 Ma with youngest monazite included in garnet yielding 23.6 Ma (Kellett et al., 2013). SK 63 is a garnet-biotite- sillimanite gneiss with dominant grain boundary migration recrystallization and minor subgrain rotation recrystallization of quartz, deformation twins in plagioclase, and myrmekite. Sillimanite occurs both as prismatic grains and as fibrolite and, along with biotite and quartz ribbons, defines the main fabric of the rock. Garnets lack internal fabric and are pervasively fractured. SK 59 is a sillimanite-garnet-biotite gneiss with myrmekite texture, deformation twins in plagioclase, undulose extinction in quartz, and biotite+quartz pressure shadows around garnet
5.3 N of Dongkung section (N 28.02326˚, E 88.59514˚)
Walking out of the gully to the north, one gains a spectacular cross-sectional view of the STDS towards the east (Fig. 5.7). To the NE, the undulating topography forming the southernmost extent of the Tibetan plateau is comprised of black slate of the TSS. Other TSS lithologies mapped in the hanging wall of the STDS in Sikkim include Devonian to Jurassic carbonate and clastic rocks (Wu et al., 1998; Edwards et al., 2002), with a sliver of Neoproterozoic-Cambrian slate, schist intercalated with sandstone, quartzite and marble mapped further to the west (Pan et al., 2004). To the SW, high-grade gneiss and leucogranite of the GHS exhibit a more resistant, blocky weathering profile, and form the northernmost peaks of the Himalayan range. The two - part nature of the STDS can be observed in Figure 5.7. The footwall GHS rocks comprise a diffuse, ≤ 1km thick ductile shear zone with top-to-NE sense of shear. This ductile shear zone is cut by a steeper, ductile-brittle fault that juxtaposes the unmetamorphosed TSS hanging wall against the amphibolite- to granulite-grade gneisses. These structures may be akin to the better known Lhotse (lower) and Qomolangma (upper) detachments which cut through Mt. Everest (e.g. Searle et al., 2003). The lower structure has been interpreted as the upper boundary to a mid-crustal channel of deformed rock that flowed to the south during Miocene, while the upper boundary is interpreted to be a later Miocene normal fault that has unroofed and cooled the paleo-channel (Kellett and Grujic, 2012, Kellett et al., 2013).
Figure 5.7. Panoramic view of the South Tibetan detachment system
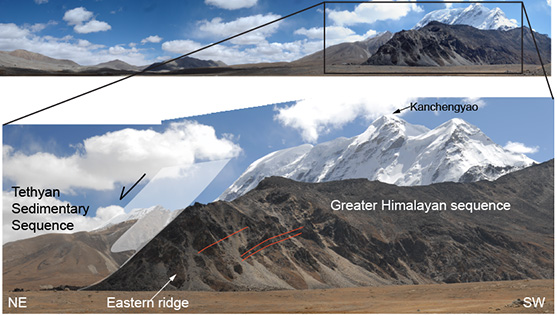
The outcrops identified by stars in the lower photo are 75 m apart. The dip surface of the upper discrete fault is show in white, while red lines show the shallower dip of the lower diffuse ductile shear zone in its footwall. The stark contrast in lithology and weathering profile was used to delineate the regional trace of the STDS in Sikkim shown in Figure 5.1. Lower photograph is modified from Kellett et al. (2013)
5.4 Eastern ridge (N 28.01139˚, E 88.60420˚)
Following the road towards Gurudongmar Lake north from the Dongkung section, one can access a ridge to the east of the valley. Here called the eastern ridge (Fig. 5.2), outcrop comprises migmatized, garnet-biotite-sillimanite gneiss and augen gneiss of the GHS (Fig. 5.8b, c), locally restitic in composition, and local bands of both biotite-bearing dark quartzite (Fig. 5.8a) and calcsilicate. As in the Dongkung section, STDS deformation appears to have postdated peak metamorphism and occurred under sillimanite-stable conditions. Muscovite is locally associated with shear bands. Like the Dongkung section, gneisses are locally mylonitized and folded, however mylonitiza tion is more generally pervasive. Rocks exhibit isoclinal folds that predate mylonitization (Fig. 5.8a,b) and well-developed S-C fabric (Fig. 5.8c) with kinematics consistent with the Dongkung section, top-down-to-NE. Fibrolite is pervasive and particular ly defines the mylonitic foliation. In thin section, flame perthite is preserved, and dynamic recrystallization of quartz by grain boundary migration is pervasive. Crystallographic preferred orientations of quartz c-axes in quartz-rich samples from this area record that dynamic recrystallization as strongly developed, largely symmetric, point maxima (Fig. 5.9) This pattern indicates activation of the prism <a> plane (Schmid and Casey, 1986) and is significantly different than the type II fabrics recorded structurally lower in the Dongkung section (Fig. 5.6). The change in c-axis orientations may indicate that the present fabrics developed in a kinematic environment approximating plane strain deformation. Moreover, the dominance of prism <a> slip is consistent with these structurally higher fabrics being developed at high temperatures during non-coaxial strain (e.g. Lister and Dornsiepen, 1982; Law et al., 1990).
Comparatively, rocks at this site record a higher degree of crystal-plastic deformation than in the Dongkung section. This is evidenced by the more pervasive mylonitization, developed S-C fabric, crystallographic preferred orientation patterns in quartz and the generally finer grain size. This strain localization could be rheologically controlled by the relatively more quartz-rich lithologies at eastern ridge, or by locally higher strain rates up-structural-section, consequently preserving a strain gradient.
Figure 5.8. Isoclinal folds and S-C fabric
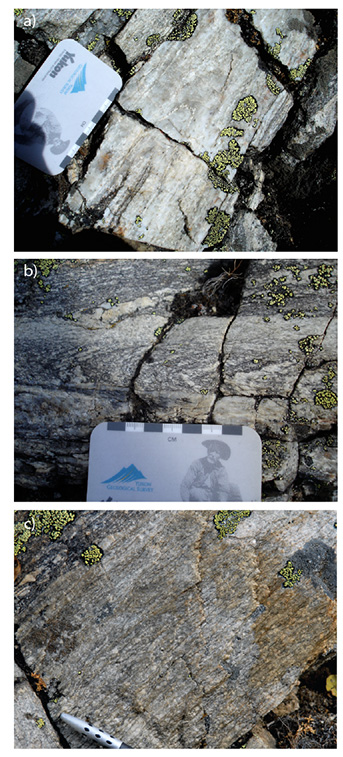
a) Isoclinal fold in quartzite. b) Isoclinal fold cut by mylonite fabric developed in augen gneiss and leucosomes. c) S-C fabric in augen gneiss. View in all photographs is towards E
Figure 5.9. Crystallographic preferred orientations in quartz
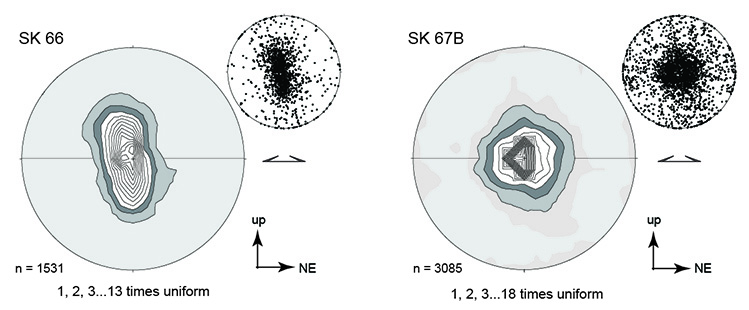
Crystallographic preferred orientations in quartz from a) feldspar-bearing quartzite and b) mylonitized augen gneiss
5.5 Gurudongmar Lake (N 28.03472˚, E 88.70833˚)
Gurudongmar Lake is the final stop in this northern section. The lake sits on the southernmost edge of the Tibetan plateau and reflects the north face of Gurudongmar peak and neighbours in its waters (Figs. 5.10, 5.11). These peaks south of the lake comprise gneiss as well as substantial leucogranite bodies (Fig. 5.12). On the eastern flank of the lake, gently north-dipping, variably incompetent and bedded outcrop is diagnostic of TSS rocks (Fig. 5.13), also evidenced by abundant slate float occurring along the path which
Figure 50. Google Earth image of the Gurudongmar Lake area
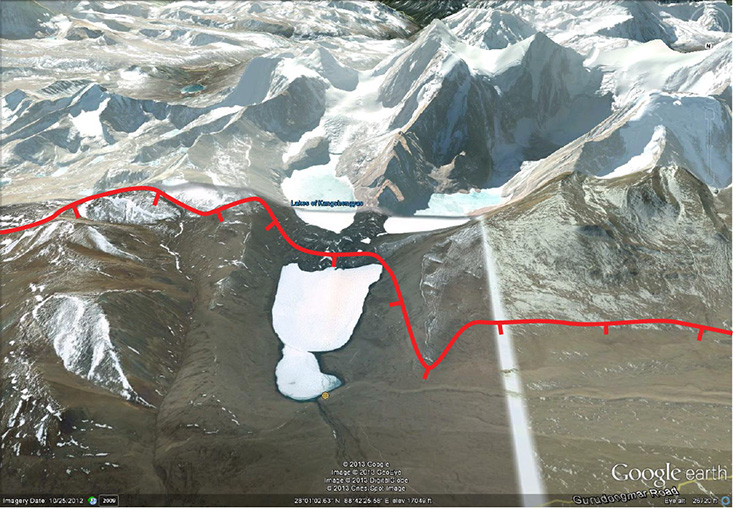
View is tilted and towards south. North-south length of Gurudongmar Lake is 1.8 km. The interpreted trace of the South Tibetan detachment system upper structure is shown in red, separating GHS rocks in the footwall to the south from TSS rocks in the hanging wall to the north
Figure 5.11. View of footwall GHS rocks

View to SSW of footwall GHS rocks that form Gurudongmar and neighbouring peaks, lining the south shore of Gurudongmar Lake. The path that circles the lake can be viewed along the shoreline. A thick layer of glacial moraine till separates the lake from the mountains
Figure 5.12. Close up view of Gurudongmar peak
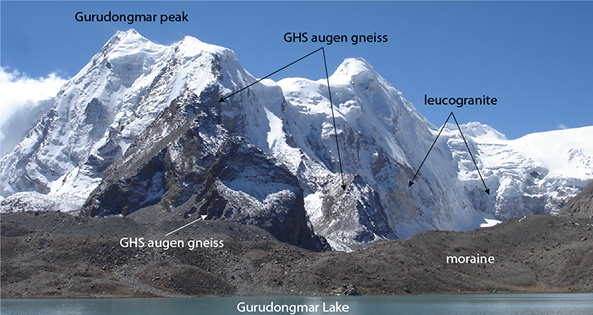
Leucogranite bodies stand out with their pale weathered surface in comparison to the darker augen gneiss. Lake surface elevation is 5154 m, and Gurudongmar peak reaches ~6500 m above sea level
Figure 5.13. View towards the eastern shore of Gurudongmar Lake
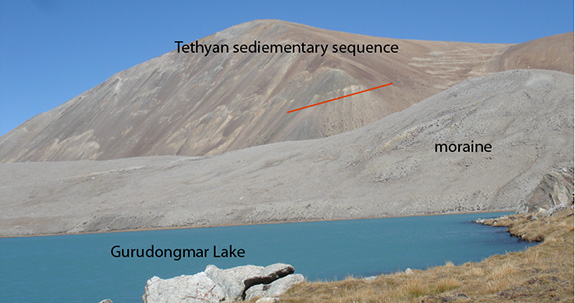
The hill in the background is comprised of north-dipping grey, tan and rusty layers (N-dipping tan layer highlighted with a white line intentionally below outcrop) of relatively incompetent rock, likely slate and other siliciclastic sedimentary rocks of the TSS. Comparing with Figures 5.10 and 5.12, the STDS passes just beneath the lake, with its surface expression buried by glacial till