Tethyan Sedimentary Sequence
In the central Nepal Himalaya, the TSS is a ~10 km thick unmetamorphosed to weakly metamorphosed Palaeozoic and Mesozoic sedimentary sequence, which was deposited on the northern Indian palaeocontinental margin and subsequently deformed during Himalayan orogenesis (Heim and Gansser, 1939; Gansser 1964; Fuchs, 1964; Fuchs 1967; Bordet et al. 1981). The TSS is bound to the north by the Indus-Tsangpo suture zone (Fig. 1) and to the south by the STD, separating it from the lower Greater Himalayan Sequence (Yin, 2006; Antolin et al., 2011) (Figs. 1, 2). The TSS represents the evolution of the Paleo-Tethys Sea, from the pre-rift stage (Cambrian–Ordovician) to the final break-up of Gondwana in Early Cretaceous (Gaetani et al., 1991; see Garzanti, 1999 for a detailed stratigraphic framework; Fig. 4).
Figure 4. Tethyan Sedimentary Sequence
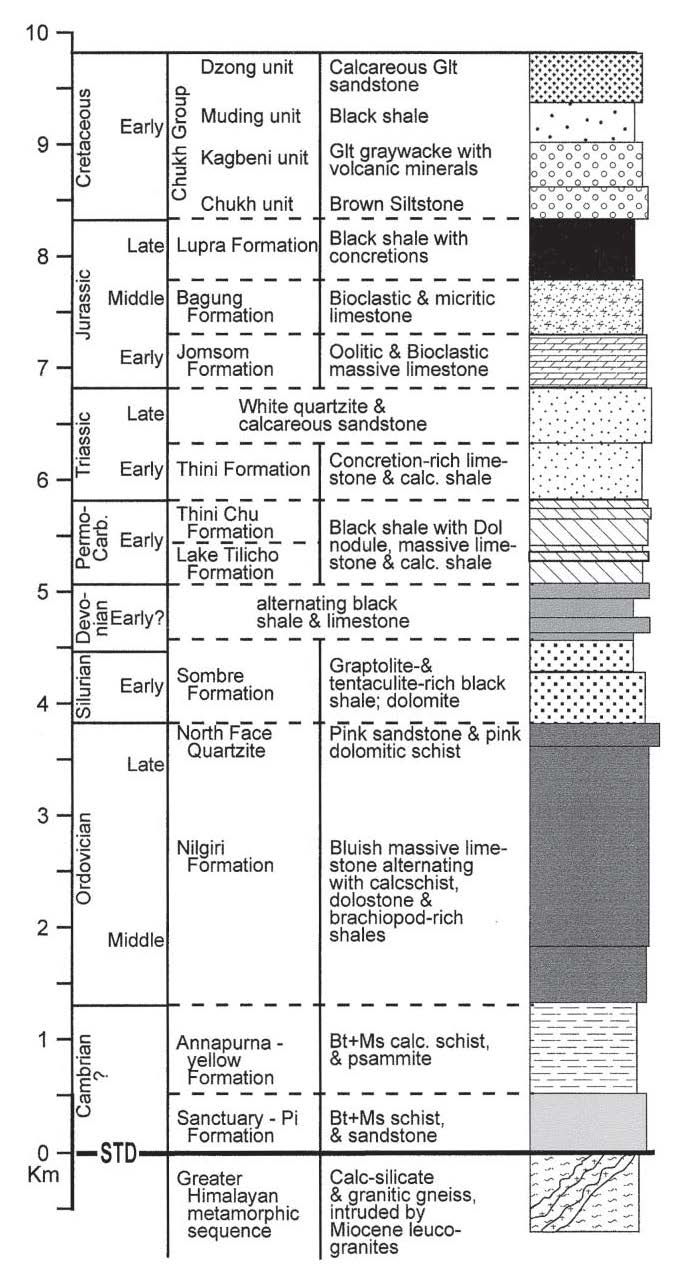
Tethyan Sedimentary Sequence stratigraphic column (Godin, 2003; Searle & Godin, 2003)
Figure 6. Structural cross-sections
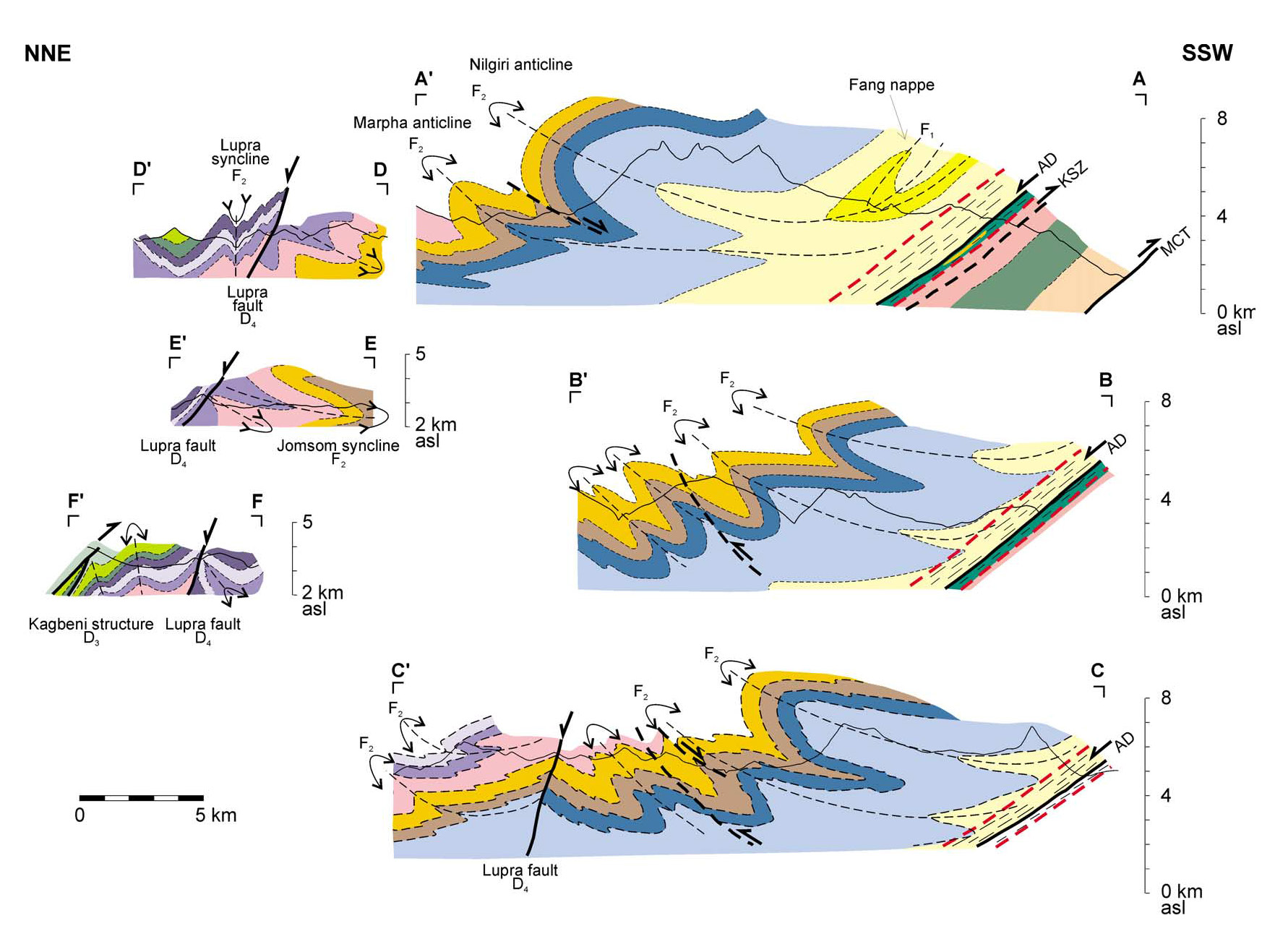
Structural cross-sections of the Tethyan Sedimentary Sequence (Godin, 2003)
In the Kali Gandaki valley, the Paleozoic section is characterized by massive limestone, calcareous shale and pelite, with local dolomite and quartzite horizons. The lowest part of this section is the Sanctuary Formation (Fig. 5; Pêcher, 1978), which comprises highly deformed black schist, sandstone and limestone. In the Annapurna area, it has only been observed on the Thulo Bugin ridge, in the core of the refolded Fang nappe. Overlying the Sanctuary Formation is the Annapurna Formation (also known as the Yellow Formation or the Larjung series: Bordet et al., 1981; Colchen et al., 1981, Colchen et al., 1986;), which is composed of calcareous biotite-grade psammitic and semi-pelitic schist and phyllite. Although they yield no direct chronologic constraints, the Sanctuary and Annapurna Formations have been interpreted as being Cambrian because they lie stratigraphically below the Ordovician Nilgiri Formation (Bordet et al., 1981). The overlying Nilgiri Formation comprises gray micritic limestone, grading upwards into pink dolomitic sandstone, calcareous shale, and siltstone. The Ordovician series is capped by a 400 m-thick calcareous arkose and siltstone unit termed the North Face quartzite (Bodenhausen et al., 1964). The upper portion of the Paleozoic is composed of alternating gritty dolomite, black shale, and limestone of the Sombre Formation (Silurian–Devonian), correlative with the Dark Band Formation of the Dolpo area, northwest of the Thakkhola region (Fig. 5; Fuchs, 1977). The Carboniferous–Permian units consist of a turbidite sequence dominated by calcareous shale with a minor siliciclastic contribution (Lake Tilicho and Thini Chu Formations; Bordet et al., 1981). The Mesozoic stratigraphy essentially comprises Triassic calcareous shale (Thini Formation), grading upwards to Jurassic fossiliferous limestone and black shale (Jomsom, Bagung and Lupra Formations), which are capped by the detrital units (conglomerates, sandstones) of the Lower Cretaceous Chukh Group (Bordet et al., 1981; Gradstein et al., 1992).
In the upper Kali Gandaki valley (Figs 5, 6), the Tethyan Sedimentary Sequence has undergone a complex structural evolution characterized by five deformational events (Godin, 2003; Kellett and Godin, 2009): southwest-verging isoclinal folds (D1); northeast-verging, tight, megascopic folds (D2); ductile extensional transposition at the base of the Tethyan sedimentary sequence along the north-dipping Annapurna detachment (D3) and related high-strain zone (Dt of Godin et al. 1999a); post-peak metamorphic, southwest-verging kink folds (D4) with related crenulation cleavages; and lastly northeast-trending, steeply dipping normal faults and spaced cleavages (D5) related to the Thakkhola graben system (Fort et al., 1982; Hurtado et al., 2001). The Tethyan Sedimentary Sequence has experienced only very low-grade to low-grade (<350˚C) metamorphic conditions with temperature increasing to the base of the TSS (Fuchs and Frank, 1970; Colchen et al., 1986; Garzanti et al., 1994; Carosi et al., 2007; Crouzet et al., 2007; Dunkl et al., 2011).
The second deformation phase (D2) dominates the structural character of the Tethyan Sedimentary Sequence with prominent north-verging structures (Godin et al. 1999a; Godin 2003; Kellett and Godin, 2009; Figs. 7, 8). The absolute age of the D2 folds is uncertain, although cross-cutting relationships indicate that they predate the dominant Miocene motion along the STDS at ~21 Ma (Guillot et al., 1993; Godin et al., 1999a; 2001; Kellett and Godin, 2009). Searle (2010) consider F2 folds contemporaneous to the motion of the STDS. Palinspastic bed length restoration of the north-verging folds implies a minimum 35% shortening, and a minimum 150% vertical thickening (Fig. 6; Godin, 2003; Kellett and Godin, 2009). These data indicate that north-verging folds played a significant role in pre-Miocene crustal thickening (150-180% thickening of 12 km stratigraphy = approximately 20 km-thick) of the Himalaya, possibly predating or coinciding with Eocene-Oligocene burial metamorphism (ca. 43-35 Ma) preserved in the underlying Greater Himalayan sequence (Godin et al., 1999a, 2001; Aikman et al., 2008; Carosi et al., 2010, in press).
Figure 7. Panorama of the Kali Gandaki valley
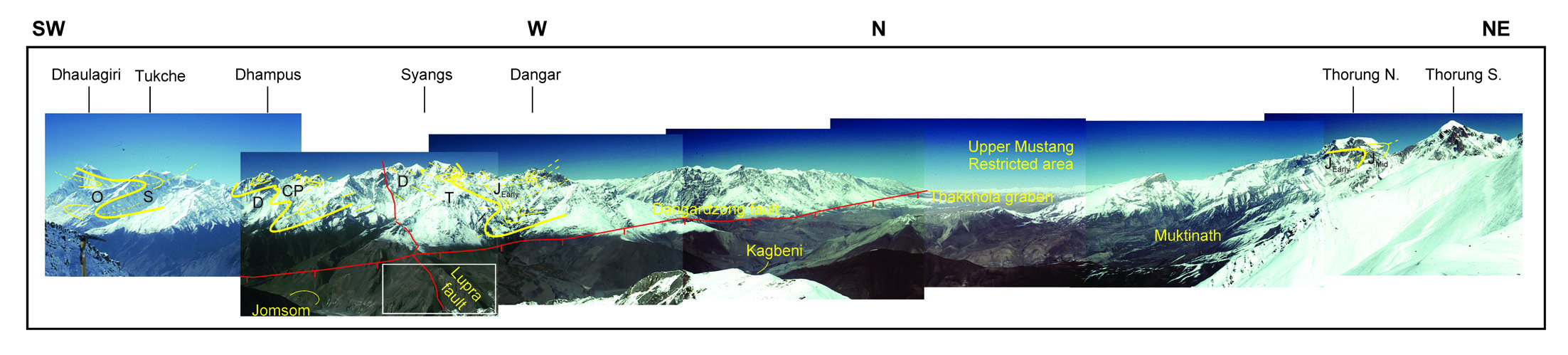
Panorama of west ridge of the Kali Gandaki valley from Dhaulagiri to Dangar summits (Godin, et al 1999a,b; 2003)
Competing models have been proposed to explain the presence of hinterland-verging folds (F2) in the Tethyan sedimentary sequence of central Nepal. Early works suggested that these folds were formed by gravity-induced sliding and drag folding coeval with motion along the STDS (Bordet et al. 1981; Colchen et al., 1981; Burg and Chen 1984; Burchfiel and Royden, 1985; Burchfiel et al. 1992; Searle, 2010). Others proposed that the north-verging folds represent features related to an early compression and crustal-thickening event that occurred prior to Miocene displacement along the STDS (Godin et al., 1999a, 2001). Alternatively, it has been suggested that the north-verging folds may have developed while the STDS was the active upper boundary of southward-extruding Greater Himalayan sequence rocks; the folds recording the opposite shear drag folding effect of a flowing mid-crustal channel (Carosi et al., 2007). More recently, Kellett and Godin (2009) argued that drag TSS associated with the southward transport of the mid-crustal Greater Himalayan sequence may have modified the geometry of already existing folds, causing the northward vergence of the folds in the TSS. This latter model has recently been kinematically validated through centrifuge analogue modeling (Godin et al., 2011).
The north-dipping, extensional Annapurna detachment, a segment of the South Tibetan detachment system, separates the base of the Tethyan sedimentary sequence from the GHS (Brown and Nazarchuk, 1993). Mapping and structural analysis reveals the presence of a 1500 m-thick high-strain zone localized in the lowermost part of the Tethyan sedimentary sequence (in the Larjung Formation) and the uppermost part of the GHS, which is linked kinematically with normal-sense ductile shearing associated with the Annapurna detachment (Godin et al., 1999a). Near Larjung, 1500 m structurally above the base of the TSS, the primary stratigraphy and D1 and D2 structures are transposed into parallelism with this broad high-strain zone, and only one penetrative fabric (S3) with an associated down-dip mineral lineation (Lm) is generally preserved (Godin, 2003). Local crosscutting relationships are observed between S2 and S3, implying that the F2 northeast-verging folds predate the penetrative fabric associated with the high-strain zone (Godin, 2003). Within the Annapurna detachment zone, shear-sense indicators consistently indicate down-to-the-northeast normal shearing (see detailed analysis in Godin et al. 1999a). However, several lines of evidence, such as asymmetric kink folds affecting partly annealed quartz ribbons (Fig. 8a of Godin et al. 1999a), also indicate that the high-strain zone was affected by subsequent localized southwest-verging deformation attributed to the D4 event.