Itinerary
We start the itinerary walking from Jomsom village (2720 m) and we follow the dirt road to the North up to Kagbeni village (2810 m). From here we come back from the same road cross-cutting all the three main tectonic units (TSS, GHS and LHS) and the STD and MCT.
TSS stops
Stop. 1.
Locality: West bank between Lupra khola and Jomson (N 28°47'55.19" E 83°45'27.74")
Theme: Lupra Fault. Large cliff, view from river bed (also good place to hunt for shelligrams in the river bed)
We move to the North and just after the old Jomsom village we can observe in the road cliff outcrops of the Jomsom Formation (Fig. 4) with alternating limestone, shale and sandstone. Crinoid ossicles, brachiopods and bivalves can be frequently observed.
The Lupra fault was first recognized by Colchen et al. (1986) and Gradstein et al. (1992), and subsequently studied in detail by Godin (2003). The Lupra fault is a steeply north-dipping, west-striking fault clearly visible in the cliffs above the Kali Gandaki river, a few kilometers north of Jomsom. The trace of the fault can be observed on the south slope of the Syang peaks where it marks a sharp structural break, and extends 20 km to the east. It terminates abruptly just south of the village of Lupra, where it is cut by a north-striking subsidiary fault of the Thakkhola graben system. It has not been observed east of this point. The Lupra fault is best expressed within the down-dropped block of the Thakkhola graben, where it has a greater offset. On the west bank of the Kali Gandaki river, the fault marks a sharp contrast between recumbent north-verging folds to the south, with a weakly folded homoclinal panel to the north. At this locality (Fig. 9), the footwall is composed of an overturned limb of a north-verging fold, within Jurassic rocks of the Jomsom, Bagung and Lupra Formations. The hanging wall consists of a north-dipping homoclinal panel of upright Triassic to Early Jurassic rocks (Thini and Jomsom Formations). Based on the stratigraphic evidence of this locality, the Lupra fault can be interpreted as a reverse fault that juxtaposes Triassic rocks in its hanging wall with Late Jurassic rocks in its footwall.
On the east bank of the Kali Gandaki, however, just south of the village of Lupra, the Lupra fault juxtaposes rocks of similar age (Lower Jurassic), but with contrasting structural style. In this area, the Lupra fault marks the boundary between upright folds (higher structural level) in the hanging wall and recumbent folds (lower structural level) in the footwall. It therefore appears that the Lupra fault also marks a structural break in the north-verging fold belt. On this basis, the Lupra fault can be interpreted as a normal fault. Unfortunately, the fault trace is located under screen slopes mainly comprised of very friable shales of the Lupra Formation or on inaccessible cliff faces. Therefore, no fault-slip data, such as slickenlines, extensional fractures or stylolites have been observed. Significant strike-slip motion along the Lupra fault cannot be ruled out, although no such evidence has been found.
The only remnants of Cretaceous rocks of central Nepal are preserved within the Thakkhola graben (Bordet et al., 1981; Colchen et al., 1981). They are be bounded by the Dangardzong fault to the west, the Muktinath fault to the east, and the Lupra fault to the south. Furthermore, the Lupra fault also marks the southern limit of the Pleistocene–Holocene lacustrine sediments that fill part of the Thakkhola graben, mainly comprising the flat plains of the Dangardzong and Phalla villages (Fort et al., 1982). The strong morphological expression of the Lupra fault within the Thakkhola graben, the higher-level structures and young sediments preserved in its hanging wall, and its steeply dipping nature all support an important normal-sense component for the fault, and a possible link with the Thakkhola graben (Godin, 2003). The apparent old-over-young stratigraphic relationship visible in the Kali Gandaki could represent some earlier thrust motion along the fault plane (Godin, 2003).
Stop 2.
Locality: Lupra village in the Lupra khola (optional side trip) (N 28°48'6.90" E 83°47'26.95")
Theme: Black shales of the Jurassic Lupra Formation, containing large ammonite and belemnite concretions. Lupra village – in the Lupra khola (optional side trip).
The 250 m thick Lupra Formation occurs over a distance of > 1500 km. It is likely more than double that thickness but intense, intra-formational deformation and pinching out renders accurate thickness estimation impossible in outcrop (Gradstein et al., 1992). The formation comprises pale- to medium-grey to black shale, which increases in silt content upwards (Gradstein et al., 1992). Some organic-rich layers are present but lamination on a fine-scale appears to be absent (Gradstein et al., 1992).
The Lupra Formation is well known for its deep shelf to upper slope macrofossils (shallower paleoenvironments would not have sustained the variety of organisms) (Gradstein et al., 1992). The presence of abundant terrestrial, organic-rich laminae within the shale could indicate that there was land nearby, but it is much more likely that the material was transported long distances out onto the shelf-slope break position (Gradstein et al., 1992). Further evidence for a deep marine depositional environment is the lack of coarse clastic material or shallow-marine facies found in a coeval lateral position (Gradstein et al., 1992).
Concretions up to 1 m in diameter are common and typically have macrofossils –ammonites, belemnites, gastropods, brachiopods and bivalves – in their cores (Gradstein et al., 1992). The concretions are generally larger in the older strata with a higher proportion of smaller concretions at higher levels (Gradstein et al., 1992). Ammonites occur throughout and are locally abundant, especially in the younger portions of the formation (Gradstein et al., 1992). The concretions probably formed during shallow to deep burial; the preservation of three-dimensional burrows in some concretions indicates that their initial growth preceded compaction (Gradstein et al., 1992). The Lupra shale is high in organic content, which was highly sought after by sulfate reducing bacteria. This reduction of the organic material formed pyrite, which often replaces the macrofossils’ structures.
The unit is best exposed around the village of Lupra (Fig. 10; in the Lupra khola, east of the Kali Gandaki). However, concretions (Fig. 11) can be found amongst the cobbles and pebbles of the Kali Gandaki between Lupra Khola and Jomson.
Stop 3.
Locality: Kagbeni; (N 28°50'12.11" E 83°46'51.32")
Theme: Kagbeni structure (reverse fault) in Early Cretaceous rocks with continental fossils (leaves, etc). Kagbeni – west bank across river (bridge). Careful not to cross northward into Mustang restricted area.
The sedimentary succession preserved in the Late Jurassic to Early Cretaceous strata of the Thakkhola region represents the passive-margin deposits accumulated during the break-up of Eastern Gondwana (Garzanti, 1999). Using paleomagnetic data it has been postulated that during the Early Cretaceous (and possibly as early as the Late Jurassic) Nepal was at a paleolatitude of approximately 38ºS. At this latitude, conditions would have favored siliciclastic deposition–assuming that Mesozoic latitudinal distribution of sediment types is similar to the present day deposition–replacing the tropical carbonate paleoenvironments of the middle Jurassic (Gradstein et al., 1992).
Interestingly, the Early Cretaceous Chukh Group deltaic strata preserved in the Thakkhola region are coeval with regressive units found in northern Europe (“Wealden”) and North America (Gradstein et al., 1992). These rock units suggest the periodic uplift of the continental margin, and may possibly be associated with major first and second order eustatic sea level drops (Gradstein et al., 1992). The Chukh Group (Chukh, Kagbeni, and Muding units) contains rift-related volcaniclastic material that was derived from the coeval Taltung Formation of the Lesser Himalaya and transported northward to the Thakkhola region (Gradstein et al., 1992). Volcanic activity during the Early Cretaceous took place after the onset of sea-floor spreading and during the break-up between India and Australia (Gradstein et al., 1992). The Early Cretaceous ended with an overall drowning of the clastic depositional shelves (Garzanti, 1999).
The Chukh Group crops out in the tectonically folded and faulted anticline on the west bank of the Kali Gandaki across from the village of Kagbeni (Fig. 12; Gradstein et al., 1992; Godin, 2003). The Kagbeni unit is found at the base and top of the Chukh unit at this locality due to structural duplication (Godin, 2003). It contains pebbles, large wood fragments and fossil leaves (Fig. 13; Gibling et al., 1994). The Chukh Group is interpreted to represent a delta complex in which the distributary channels and delta front environments are dominant (Gradstein et al., 1992; Gibling et al., 1994).
From here we move back to the south following the same road down to the beautiful Marpha village.
Stop 4.
Locality: Marpha (along trail above Marpha Gompa – west bank of Kali; N 28°45'16.96" E 83°40'42.77")
Theme: view across valley to west face of Nilgiri (North verging folds in the Ordovician limestone); also outcrop of fold interference pattern F2-F3 in Silurian calcareous shales (Sombre Fm).
Hike up for approx. 1hr. (to elevation ~3,200m). The trail starts in the center of Marpha, just south of the Gompa (Buddhist temple) in the center of village. The trail shows exposure of Siluro-Devonian calcareous shales, displaying F2-F3 interference patterns (Fig. 14). This is also an excellent place to view the spectacular “Nilgiri” anticline: The F2 folds visible in the Kali Gandaki valley generally constitute structures parasitic to crustal-scale northeast verging folds visible on the west face of the Nilgiri Mountains, where Ordovician limestones are folded in a kilometer-scale overturned antiform (Fig. 15; Bordet et al., 1981). Macroscale examples of parasitic folds of the Nilgiri anticline include the Marpha anticline (visible on the east bank of the Kali Gandaki at Marpha) and the Jomsom syncline exposed on the east bank of the Kali Gandaki immediately north of Jomsom (Godin, 2003). These structures are part of a major fold train that extends at least to the upper reaches of the Kali Gandaki in the upper Mustang–Lo Manthang area 50 km north of Kagbeni.
Stop 5.
Locality: south of Larjung village (N 20° 40’ 57.33’’ E 83° 36’ 41.70’’).
Theme: deformation in the marbles of the Annapurna Yellow Formation (Larjung Formation).
After the village of Larjung the cliff road cut exposes the banded marbles of the Annapurna Yellow Formation with a penetrative foliation (in detail it is an S3 foliation according to Godin, 2003) that dips gently to the northeast (Fig. 16a). Metre to cemtimetre scale F2 folds can be observed folding the previous S0-1 foliation (Fig. 16b, c). The S2 foliation is marked by the shape preferred orientation of recrystallized calcite crystals as well as layers of white mica and biotite. (Fig. 16d). Stable isotope data from calcite and quartz indicates there is sharp decrease in temperature from nearly 580°C at the base of the Annapurna Yellow Formation to nearly 325°C in the higher part of the Nilgiri Formation (Montomoli et al., 2013). This temperature gradient is also reflected by changes in calcite deformation mechanisms and a decreasing upward grain size.
Stop 6
Locality: North of Kalopani; side khola at Kokhetanti (N 28°39'52.97"
E 83°35'26.02").
Theme: STDS – with sheared Annapurna-Larjung-Yellow Fm, cross cut by 22 Ma leucogranitic dykes. Top north normal overprinted by reverse sense shear.
The contact between the Tethyan Sedimentary Sequence and the Greater Himalayan Sequence is visible in a stream gully at Kokhetanti, just northwest of the village of Kalopani; this corresponds to the Annapurna detachment, a segment of the South Tibetan detachment system (Figs. 1, 2). Gansser (1964) noted that although this contact appears conformable, it delineates a clear boundary between two domains of contrasting structural style. Many workers followed Gansser’s observations, and although some speculated or proposed a faulted contact (Bodenhausen and Egeler, 1971; Bordet et al., 1981; Caby et al., 1983; Colchen et al., 1986; Pêcher, 1991), the Annapurna detachment was not clearly documented until the 1990s (Brown and Nazarchuk, 1993).
The Annapurna detachment is characterized by a 1,500-m-thick high strain zone localized in the lowermost part of the Tethyan sedimentary sequence and the uppermost part of the Greater Himalayan metamorphic sequence (Godin et al., 1999a). The high-strain zone affects the lowermost section of the Ordovician Nilgiri Formation, the entire Annapurna (Yellow-Larjung) Formation (Figs., 16, 17, 18), and the uppermost 100 m of Unit III of the Greater Himalayan metamorphic sequence (Figs, 19, 21).
Several mesoscale shear-sense indicators associated with the high strain zone are readily visible. Shear-sense indicators include systematic asymmetric isoclinal folds and boudins (Figs. 17, 20), extensional shear bands, and S-C fabrics, which consistently indicate down-to-the northeast normal shearing across the high strain zone (Godin et al., 1999a). Farther to the north-east gray foliated marble of the Ordovician Nilgiri Formation can be observed overlaying the Larjung Formation (Fig. 20d). Other shear-sense indicators include pegmatite dike arrays that have been extended or shortened according to their orientation with respect to the shear plane. Leucogranite dikes (garnet + muscovite + tourmaline) intrude the high-strain zone (Fig. 18). Similar dykes are not found in the TSS exposed structurally above, nor in the adjacent regions (e. g. Searle and Godin, 2003) apart Jumla-Karnali area in Western Nepal where granites and dykes intrude the TSS (Bertoldi et al., 2011; Carosi et al., 2013a).
According to Godin (2003) several lines of evidence indicate that the high strain zone was affected by subsequent localized southwest-verging deformation. Top-to-the-southwest shear-sense indicators, including oblique boudinaged calcite veins, are locally associated with systematic southwest-verging kinks overprinting the Annapurna detachment fabric (Godin et al., 1999a).
The immediate footwall rocks exposed between Kalopani and Kokhetanti comprise:
• Unit III augen gneiss, which has an interpreted magmatic and crystallization age of 484 ± 9 Ma (Godin et al., 2001). This Ordovician granite is intruding into sillimanite-bearing metapelites that also contain Ordovician monazites, suggesting these rocks preserve a pre-Himalayan Ordovician metamorphic event (Godin et al., 2001).
• Ca. 34 Ma U-Pb monazite and zircon ages from kyanite-bearing leucosomes interpreted to coincide with Oligocene burial metamorphism of the GHS, coeval with the development of north-verging folds in the overlying TSS (Godin et al., 1999b; 2001).
The immediate hanging-wall rocks (within the high-strain zone) around Kokhetanti:
• Cross-cutting weakly-deformed leucogranitic dikes dated at ca. 22.5 Ma, indicating the ductile strain associated with the Annapurna detachment ceased by ca. 22 Ma (Fig. 18, Godin et al., 2001).
Figure 17. Shear sense indicators in the Annapurna Fm
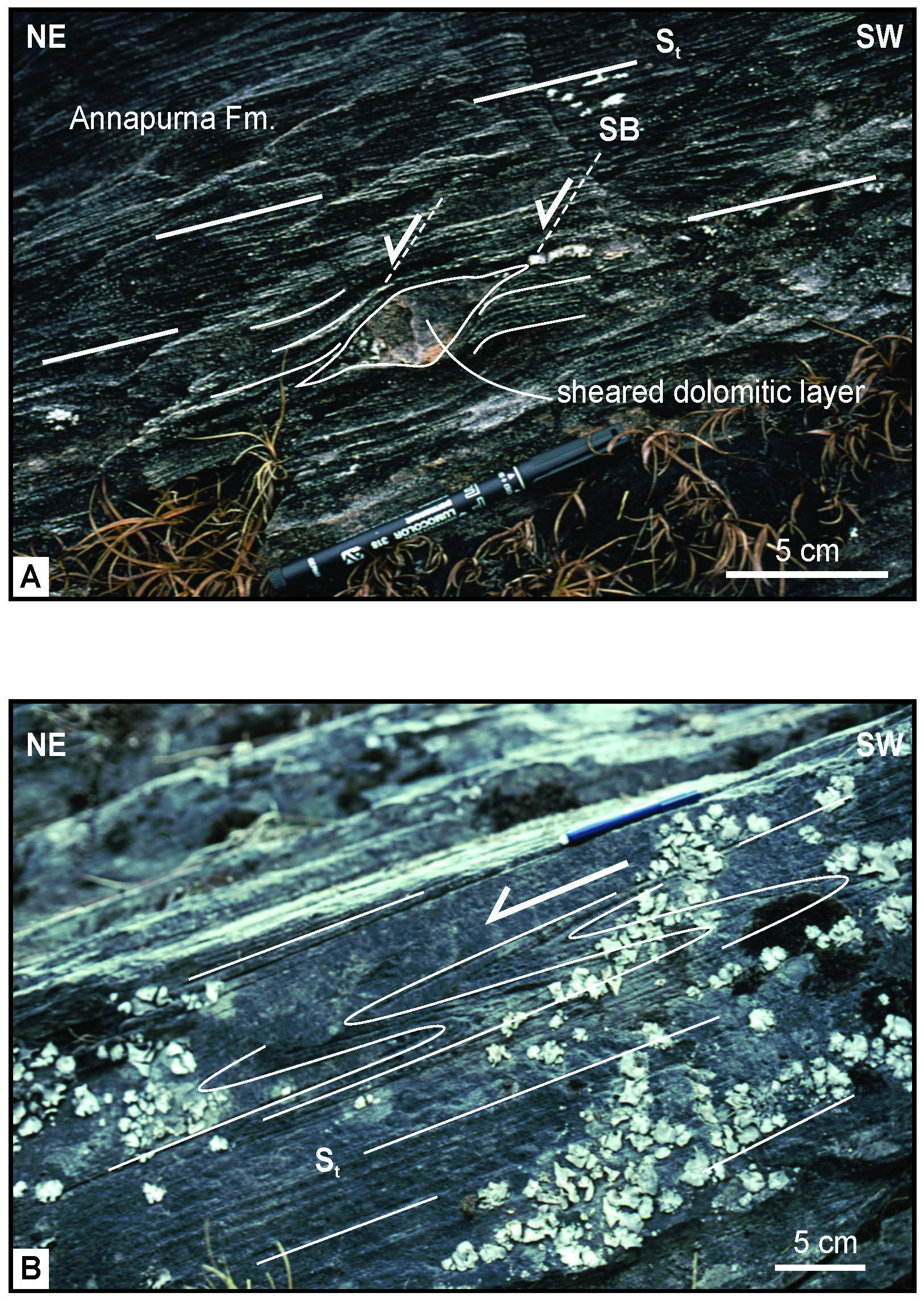
Outcrop photos with shear sense indicators in the Annapurna Fm. A: sheared dolomitic layer; B: asymmetric folds
Figure 18. Cross-cutting dyke
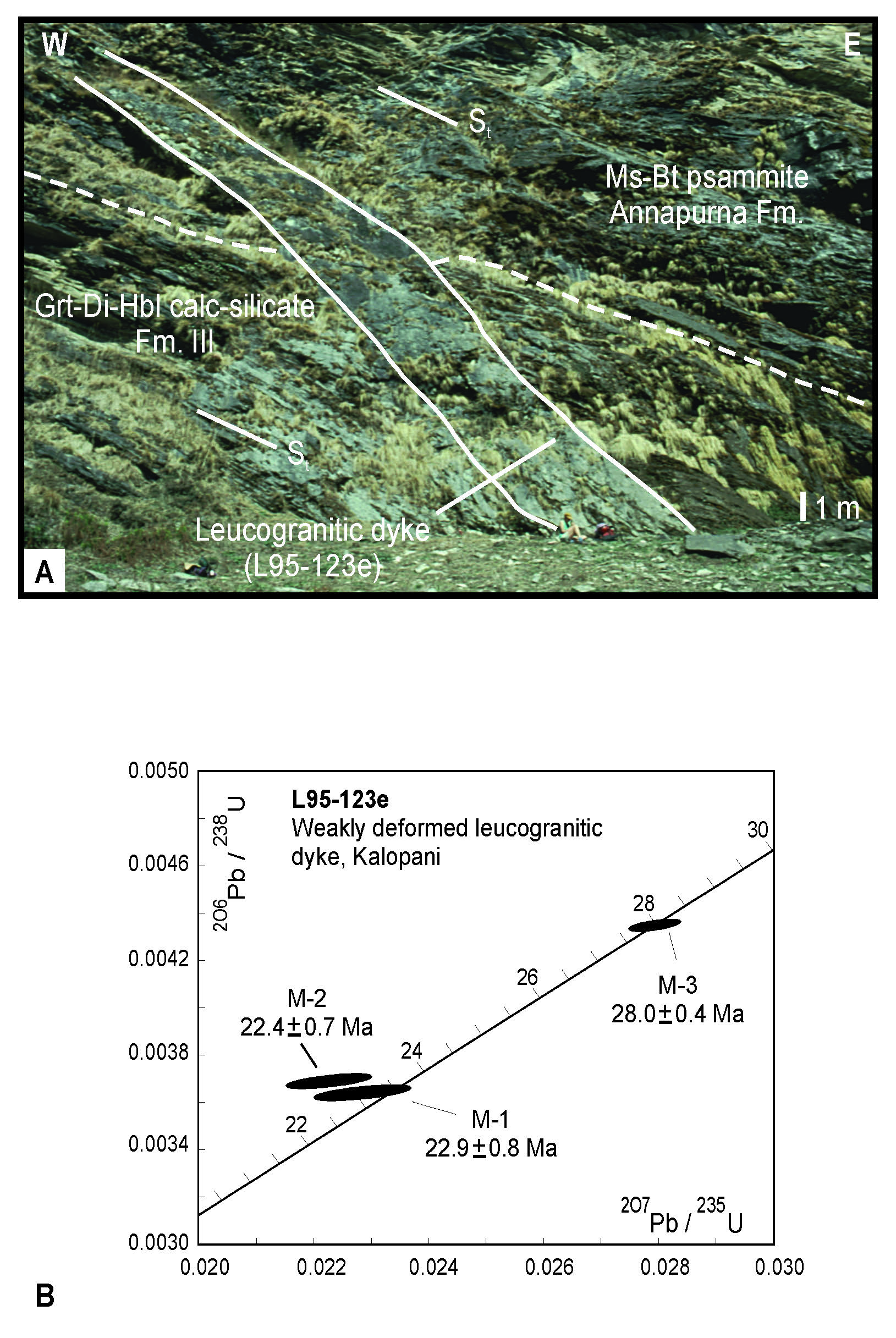
Outcrop photos showing a cross-cutting dyke (A) and its U-Pb age (B) (Godin et al., 2001).
We move to the South toward the village of Kalopani and we enter in the metamorphic sequence of the GHS. The stops 6, 7 and 8 are shown in Fig. 19 (indicated as 1, 2 and 3 respectively in the figure).
Figure 19. Itinerary of the stops 6-8 and geological feature of the area
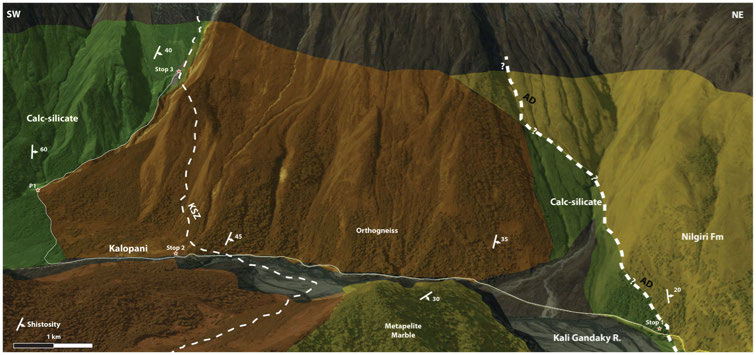
The stops are indicated by red stars (Stop1= 6, Stop 2 is 7 and Stop 3 is 8). KSZ: Kalopani shear zone; AD: Annapurna Detachment
Figure 20. Annapurna Yellow Formation (Larjung Formation)
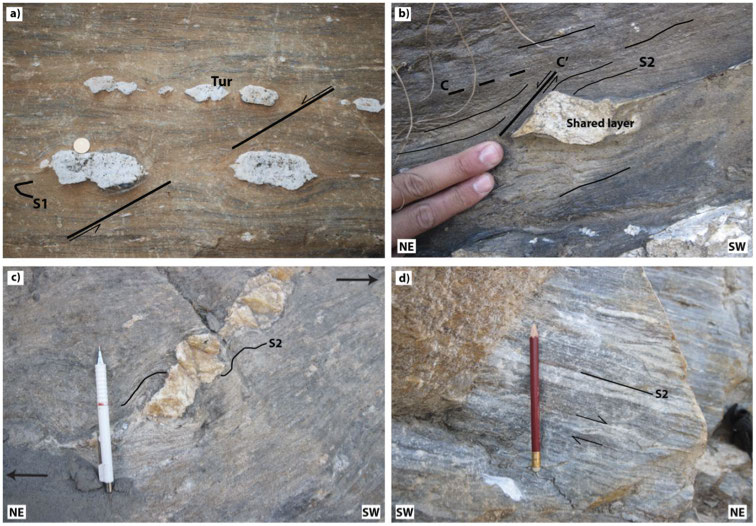
Rocks and associated structures related to the high strain zone of the Annapurna Detachment. A: Shear-type boudins of leucogranitic dykes in the foliated metapelites and calc-silicates. B. Extensional shear band of a leucogranitic dyke affecting the metapelite of the Larjung Formation. C: Flanking fold showing a dextral movement towards the north-east. D: Foliated marble with a rotated centimeter sigma-type quartz-fish pointing a top-to the NE sense of shear
Stop 7.
Locality: Kalopani-Lete (N28° 38’ 37,2”- E83° 35’ 43,8”)
Theme: Top-to-the South ductile shear zone in the upper part of the GHS.
Near the village of Kalopani, the west side of the valley is characterized by the homoclinal foliation of the GHS dipping moderately to the north (Fig. 21). Proceeding along the main road a few hundreds of meters to the north of Kalopani village we come across a road cut exposing augen gneiss of the Unit 3. The Kalopani shear zone (KSZ; Vannay and Hodges, 1996) crops out about 1 km north of the village of Kalopani. It is a 20-50 m thick ductile shear zone (Fig. 19) that strikes NW-SE and dips moderately to the NE. Elongated crystals and boudins mark the stretching lineation trending NE-SW and plunging 30-40° to the NE. A mylonitc foliation (S2), which transposes the main foliation, is systematically observed at different scale. S2 is marked by shape-preferred orientation (SPO) of biotite and muscovite (Fig. 21 a-b).
Figure 21. Geologic cross section of the Annapurna-Dhaulagiri area
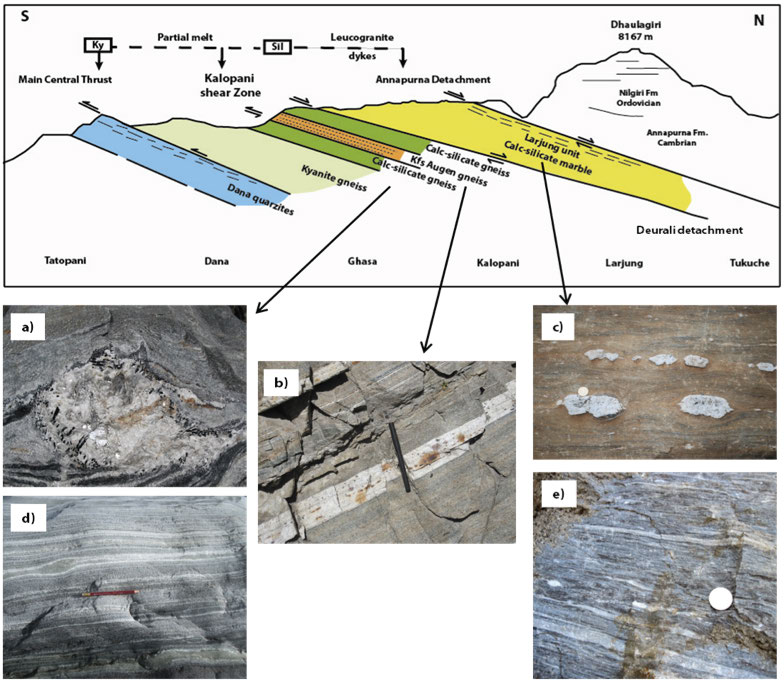
North-south geologic cross section of the Annapurna-Dhaulagiri area in the Kali Gandaki valley. The arrows recalls the structural position of the rock types in the images. Modified after Searle (2010). a) muscovite and tourmaline in situ melt from orthogneiss in Unit 3, the leucosome is sheared and shows asymmetric wings; b) sheared tourmaline and garnet leucogranitic dike parallel to the S2 foliation; c) metapelite from Larjung Formation; d) penetrative S2 foliation in a calc-silicate of Unit 2; e) foliated gray marble from Annapurna Yellow Formation (Larjung Formation).
The meso- and micro-scale kinematic indicators such as: shear bands, mineral fish, rotated porphyroclasts, mantled objects and boudins record a top-to the SW sense of shear (Fig. 22 a, b,c ). Microstructural observations indicate high-T deformation as evidenced by lobated grain boundaries, quartz/feldspar ribbons, pinning microstructures, blocky undulatory extinction patterns in quartz, and myrmekites (locally asymmetric) in feldspar porphyroclasts (Fig. 23).
Figure 22. Kalopani shear zone
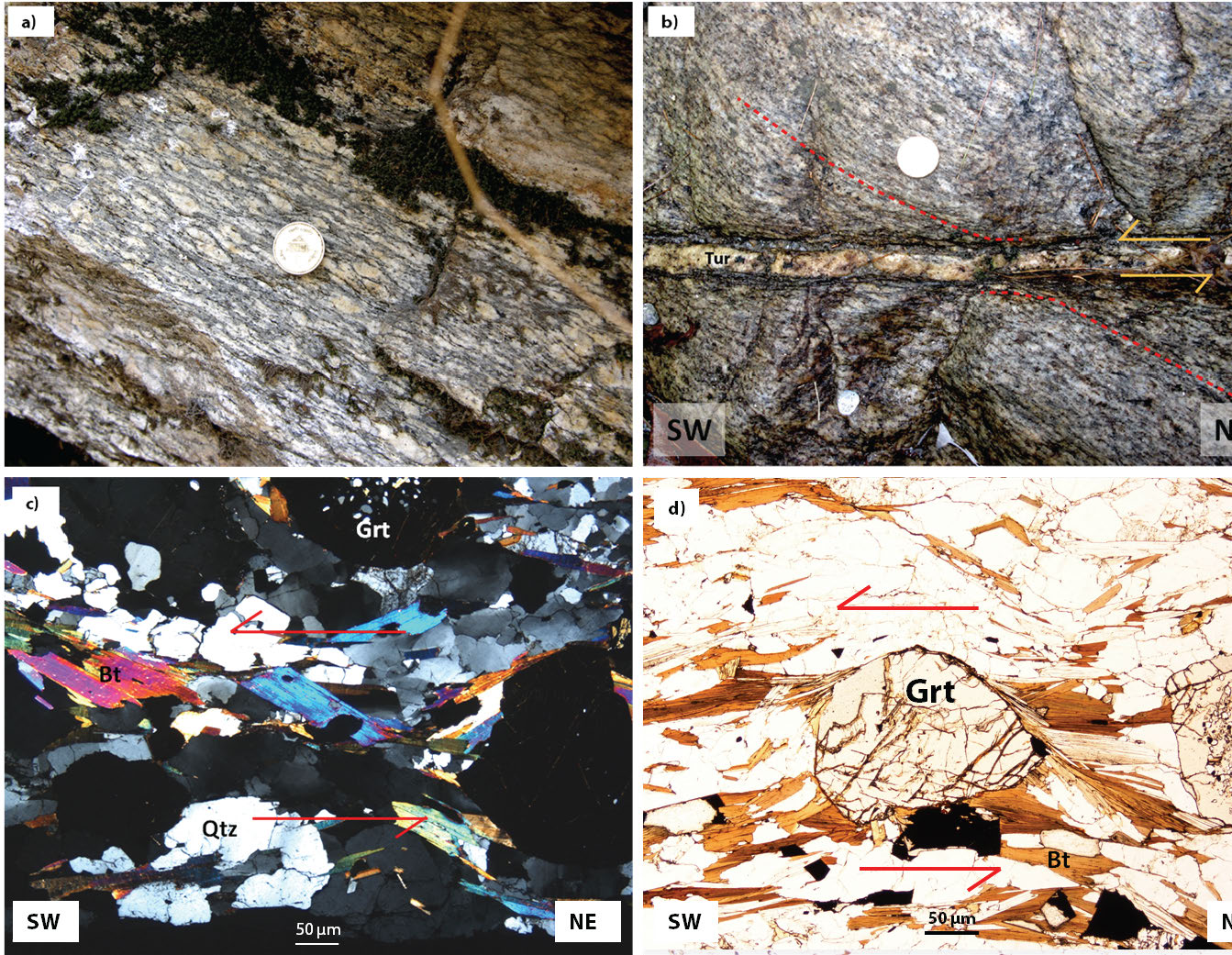
Outcrop photo (a-b) and microstructure (c-d) of the mylonitic orthogneiss within the high-strain zone with a top-to-the SW sense of shear. A: Outcrop photo of the augen gneiss of unit 3. B: Shear band affected the orthogneiss near Kalopani, the sense of shear is top-to the South-west. The core of the shear band is composed by a Tur leucogranite. The sheared foliation S2 is indicated (red). C: microstructure of the Grt paragneiss (2 nicol), mylonitic fabric and the mica-fish object indicating SW-verging simple shear of the Kalopani shear zone. Lobate contact within quartz ribbon indicating partial recrystallization. Rotated garnet with inclusion trails of quartz (S1 relict). D: Rotated garnet from a KSZ paragneiss (1 nicol)
Based on 40Ar/39Ar geochronology Vannay and Hodges (1996) interpreted movement across the shear zone to be older than 15-13 Ma. Godin et al. (2001) placed further constrains on the shearing to be between 15 Ma and 22.5 Ma. New data in progress obtained by U-Th-Pb in situ isotopic analyses on monazite reveal that shearing could have initiated along the KSZ earlier than 15-22.5 Ma, potentially before 28 Ma (Carosi et al., 2013b).
Figure 23. Kalopani shear zone microphotos
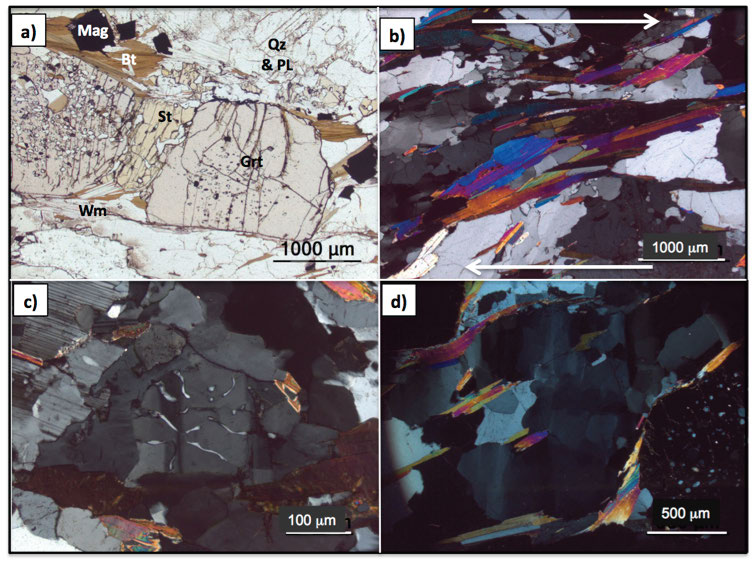
a) Garnet and staurolite porhyroclasts in the sheared paragneiss; b) mica fish pointing to a top-to-the South West sense of shear: othognriss; c) myrmekitic feldspar in the sheared orthogneiss; d) chess-board extinction in quartz testyfing simultaneous basal and prismatic slip under high-T conditions: orthogneiss
Stop 8
Locality: Lete river valley (N28° 37’40,9”- E83° 34’56,3”)
Theme: GHS; contact between Unit 2 and Unit 3.
We move to the south from the village of Kalopani along the main road to Lete village. After walking nearly 500 m we take a little track in the forest on the west side of the road and we follow it until we find the Lete river (Fig. 19. We follow up the river and at an altitude of nearly 2700 m the river splits in two parts; we follow the northern branch. From this point is possible to observe mega-scale F2 Nilgiri anticline and refolded F1 fang nappe to the east as explained in the line drawing of Fig. 24.
Figure 24. Photograph of the west face of Mt. Nilgiri and Mt. Annapurna
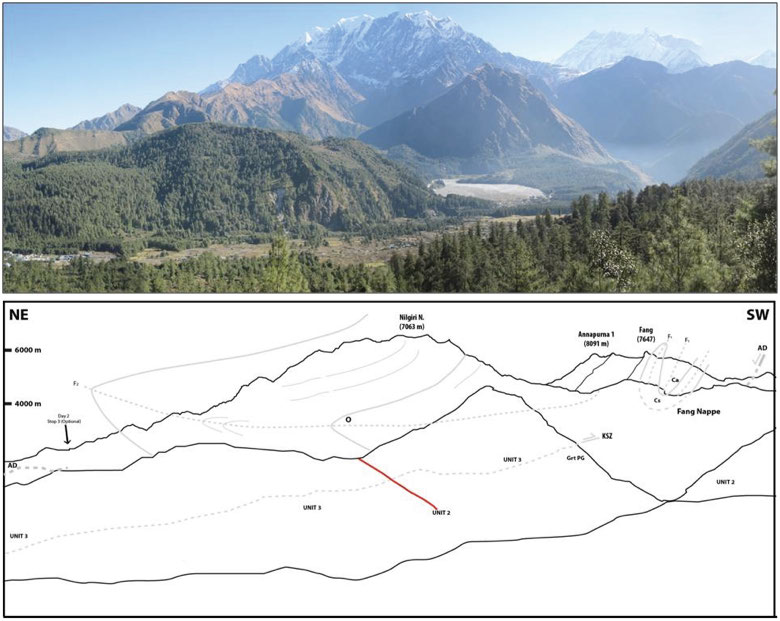
Photograph of the west face of Mt. Nilgiri and Mt. Annapurna as seen from position P1 in fig. GHS-1. The line drawing shows the projected contacts of the Paleozoic units that outline the F2 Nilgiri anticline and the folded F1 Fang nappe. The red line rapresent the normal fault system associated with the Takkhola graben (Hurtado et al., 2001). AD, Annapurna detachment, KSZ, Kalopani shear zone, CA, Cambrian-Ordovician, CS, Cambrian-Ordovician Santuary Formation, O, Ordovician, S, Silurian
On the north side (left bank) of the river we cross the orthogneiss of Unit 3, and on the southern side (right bank) green calc-silicate of the Unit 2 is well exposed. The calc-silicate is characterized by a planar penetrative foliation dipping towards the north - north-east.
The contact between the units is parallel to the main foliation that is locally related to large- to small-scale irregular isoclinals folds.
Unit 2 is mainly represented by calc-silicates gneiss and marbles with the mineral assemblage made by Qz + Pl + Kfs + Bt + Ep + Cpx + Hbl + Cal + Scp + Grt + Ms + Ttn and others accessories (mineral abbreviations after Whitney and Evans, 2010), indicating an upper amphibolite facies metamorphic condition (Miyashiro, 1994; Vannay and Hodges, 1996). Gneiss shows a granoblastic-polygonal texture, suggesting a post-kinematic annealing of the fabric due to GBAR (grain boundary area reduction; Passchier and Trouw, 2005) in amphibolites facies conditions with local fluids redistribution on grain boundary as suggested by coronitic brown amphibole overgrowths on Cpx on cooling. Locally up to 20% (by volume) of leucogranitic bodies (Larson and Godin, 2009) are present (Fig. 25, interpreted as sheared sills and/or transposed dykes. Constraints on metamorphic condition of Unit 2 and on its timing in central Nepal are reported in Kohn and Corrie (2011). These Authors coupled titanite U-Pb geochronology with Zr-in titanite thermometer, revealing how this calcsilicate rocks have experienced a very high temperature (> 700 °C up to 775°C) metamorphism for a long protracted period of time, more than 10 Ma (c. 37 to 24 Ma), before cooling starts around 20 Ma. Similar T values are also recorded by titanites in the calcsilicate of Kali Gandaki transect (Iaccarino S., unpublished data).
Figure 25. Middle GHS rocks from unit 2 and unit 3 exposed in the Lete khola valley
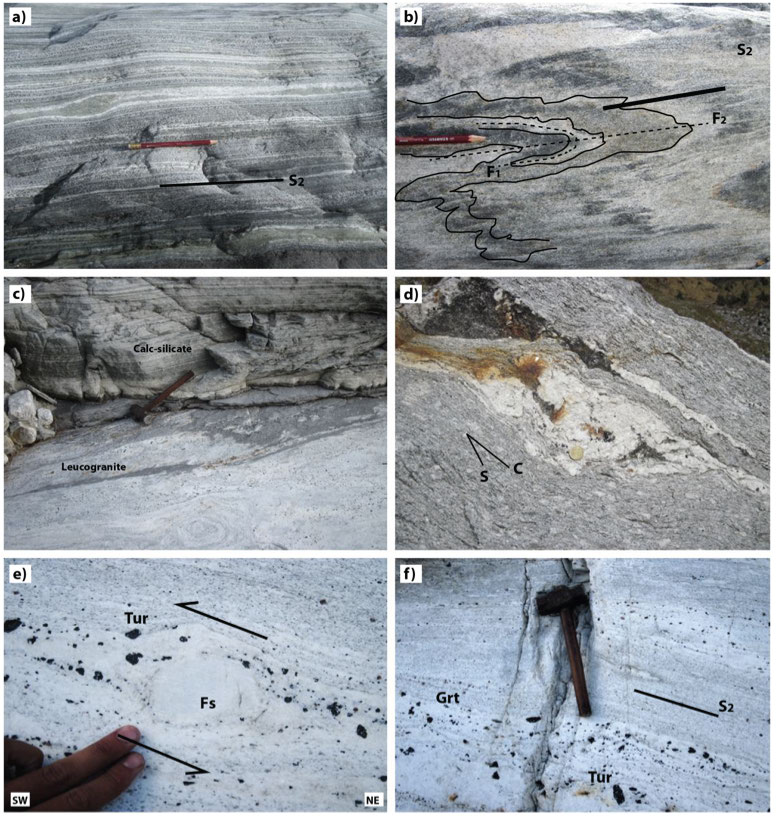
A: Penetrative S2 foliation in the Calc-silicates. B: view of the F1 axial plane folded by the irregular isoclinals folding of F2, and S2 Axial plane foliation in the calc-silicates. C: Contact between leucogranite and calc-silicate. D: Tourmaline crystals in in situ melt from augen gneiss of unit 3 C-S plain in the mylonitic foliation. E: Centimeter δ-type feldspar porphyroclast indicating top-to-the NE sense of shear in the leucogranite. F: Rotated porphyroclasts of tourmaline and garnet in leucogranite
Proceeding upward in the valley, close to the river at an altitude of nearly 3000 m (N 28° 37’40,9”-E 83° 34’56,3”), we observe a transposed contact between calc-silicate gneiss and a plurimetric tourmaline-garnet leucogranite; interpreted to be units 2 and 3 respectively. In the calc-silicate gneiss the S1 foliation is not exposed at the meso-scale, and F1 axial planes are folded by F2 (Fig. 24 a-b). D2 structures mainly consist of isoclinal folds, from centimeter to metre-size, transposing the S1 foliation into a penetrative S2 axial plane foliation, that varies from spaced to continuous. We observe rotated porphyroclasts of feldspar showing a top-to-the NE sense of shear related to the deformation phase D2. Centimeter to pluri-centimeter crystals of tourmaline and garnet are rotated in deformed leucogranite sills (Fig. 25 e-f).
Stop 9.
Locality: South of Ghasa village (N 28° 35’ 23’’ E 83° 38’ 47’’)
Theme: GHS; calc-silicates of Unit 2
Choose a stop near to, but after, the suspension bridge south of Ghasa village to examine the calc-silicates of Unit 2 and cm-size diopside crystals (Fig. 26a). In thin section we can observe coronitic amphibole overgrowths on Cpx (Fig. 26b).
Figure 26. Gneiss in Unit 2
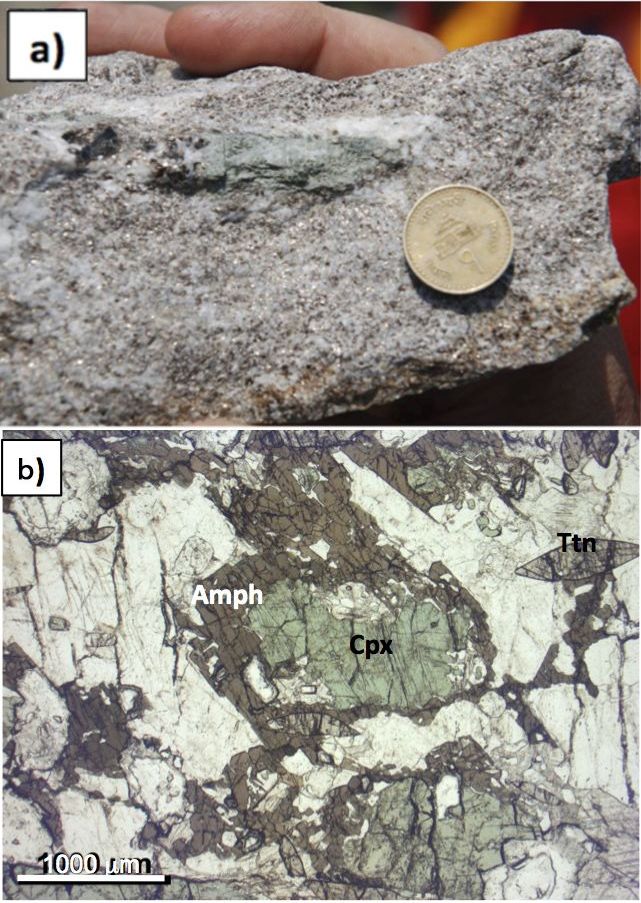
a) Cm-size diopside crystal (green) in biotite-gneiss; b) Microphoto showing amphibole coronae on clinopyroxene in a titanite bearing calcsilicate gneiss
Stop 10.
Locality: N 28° 34’ 13’’; E 83° 38’ 20’’, 1787 m a.s.l.
Theme: GHS; biotite- muscovite -kyanite-garnet bearing schist and gneiss of Unit I
This stop roughly corresponds to the stop G42 of the Field Guide book by Upreti and Yoshida (2005).
Following down the road in between Rupse-Chhahara and Titar villages, fresh gneiss and micaschist crop out along the road cut for several kilometers.
The outcrop exposes biotite-muscovite-kyanite-garnet bearing micaschist and gneiss, interlayered dm-thick amphibolite layers containing centimetric garnets (Fig. 27a). The kyanite and garnet in the micaschist and gneiss is cm-size. Kyanite is particularly abundant in quartz veins and quartz-feldpsar leucocratic layers parallel to the main foliation along the road cut where this aluminosilicate can reach several cm in size (Fig., 28 b, d).
The mineral assemblage of the gneisses is: Qz + Pl + Bt + Grt + Ky + Ms ± Sil, with locally retrograde Chl. Accessory minerals are Mnz, Ap, Zrn, Tur, Rt, Ilm, Py and rare Xtm.
Figure 27. Biotite- muscovite -kyanite-garnet bearing schist and gneiss
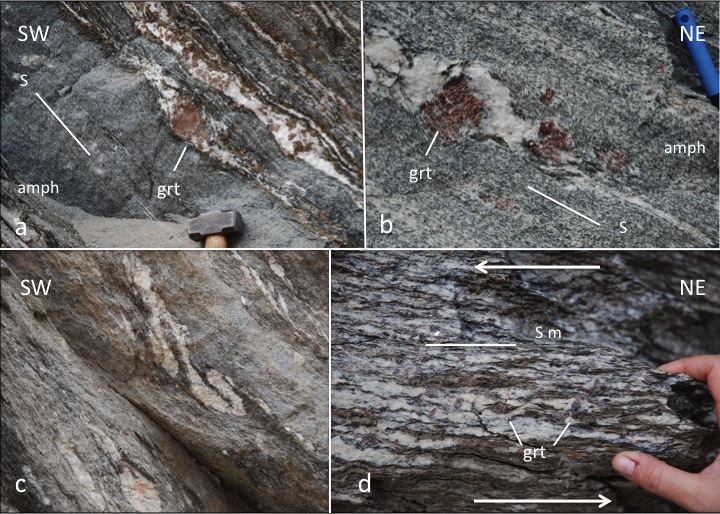
a) Amphibolite with cm-size garnet bearing layers; b) detail of the foliation with mm-size green amphiboles and deformed garnet and deformed veins with cm-size garnets; c) stretched and isoclinally folded vein along the main foliation; d) biotite and sillimanite bearing mylonitic foliation with asymmetric pressure shadow around garnet with a top-to-the SW sense of shear
Figure 28. Biotite- muscovite -kyanite-garnet bearing schist and gneiss
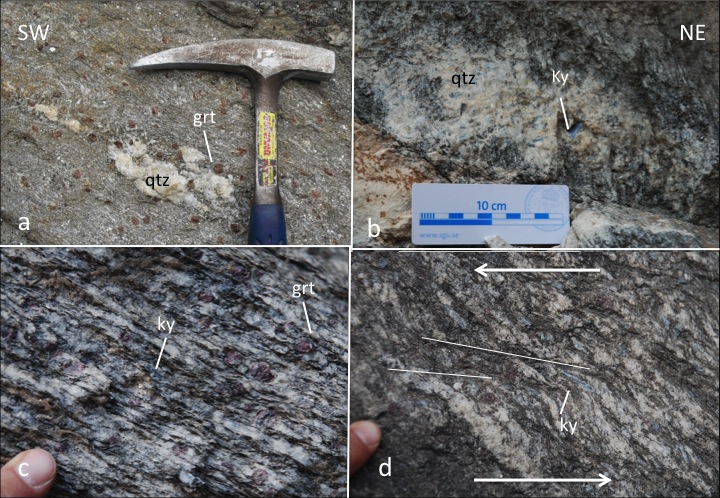
a) Garnet bearing micaschist; b) cm-size kyanite crystals in leucocratic vein; c) kyanite-garnet paragneiss; d) shear bands with a top-to-the SW sense of shear linked to the MCT shearing affecting kyanite and garnet
The main foliation is marked by aligned biotite, white mica, quartz, feldspar and sillimanite and dips moderately to the NE (Figs. 27, 28). It wraps around garnet and kyanite porphyroclasts. Quartz veins and leucosomes are deformed in tight asymmetric folds, with stretched limbs, verging to the SW (Fig. 27 b, c).
Kinematic indicators include shear bands, C-S fabrics and sigma type-asymmetric tails around garnet porphyroclasts that indicate a top-to-the SW sense of shear (Figs. 27, 28) in agreement to the shearing linked to the MCT few hundreds meters structurally below (Fig. 29a, b).
Needles of fibrolitic sillimanite occur rarely within the fractured kyanite, sometimes at the rims of biotite and muscovite in contact with garnet and at the boundary of plagioclase. This is a microstructural evidence of P-T-path of the rock crossing the stability field of sillimanite after the main assemblage Ky-Bt-Ms-Grt-melt equilibrated (mineral abbreviation according to Whitney and Evans, 2010). The mineral assemblage Qtz + Pl + Bt + Ms ± Grt ± Ky (+ former melt, see below) characterizes the prograde metamorphism (Vannay and Hodges, 1996) whereas the growth of sillimanite occurred later during decompression. A late stage of greenschist facies retrogression is sporadically testifyed by growth of late Chl on Bt and along cracks of Grt.
Figure 29. Main Central Thrust near Dana village
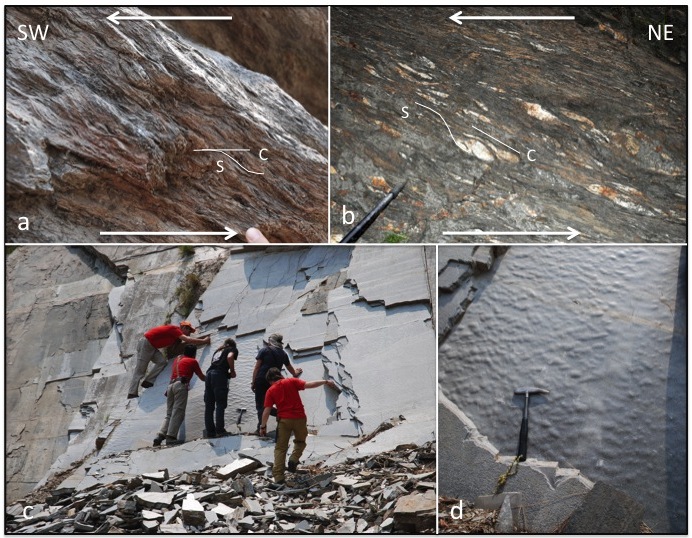
Location according to Colchen et al. (1986). a) Phyllites with C-S fabric showing a top-to-the SW sense of shear; b) sigmoidal quartz veins and C-S fabric showing a top-to-the SW sense of shear; c) Quartzite layer dipping to the north just below the kyanite-garnet bearing gneiss and micaschist; d) zoomed-in photo of the foliation surface of the quartzite in c).
It is worth of note that the “peak” P-T estimates of ~ 0.8-1.0 GPa and 600-650°C reported by Vannay and Hodges (1996) on these rocks, based on rim geo-thermobarometry, reflect probably a late re-equlibration as also suggested by recent data based on P-T pseudosection calculation and Zr-in rutile thermometer (Iaccarino, unpublished) as well as the findings of nanotonalites inclusion in garnet (see below).
Both quartz and plagioclase grains show microstructures indicating recovery and dynamic recrystallization processes. Quartz grains have lobate grain boundaries, internal subgrains that are locally formed a chessboard pattern. Plagioclase feldspar also has lobate grain boundaries, deformation twins and a distinct lack of internal microfractures. Kyanite is often twinned and sometimes shows undulose extinction. Pinning and windows microstructures (Passchier and Trouw, 2005) are observed in quartz indicating Grain Boundary Migration recrystallization at relatively high temperature of deformation.
Garnet grains are zoned with a core and inner rim grown during prograde metamoprhism whereas the outer rim is interpreted to have grown during retrograde P-T conditions as indicated by compositional X-ray maps (Ca, Mg, Mn and Fe) (Carosi et al., in press).
It is worth noting that Ca-rich melts (nanotonalites inclusions) have been found in cm-size garnets from this outcrop indicating partial melting in the kyanite stability field (Carosi et al., in press). In situ U-Th-Pb geochronology on monazite in the same microstructural position as the nanotonalites (further confirmed by microchemical zoning in garnet) provide information of the timing of their entrapment at ~ 36-41 Ma during early Eo-Himalayan metamorphism (Carosi et al., in press). Older Eo-Himalayan ages, up to ~ 35 Ma, have been reported also by Godin et al. (2001) in monazite of Unit 3 associated to the generation of kyanite-bearing leucosomes.
The composition of Ca-rich melts is in agreement with the occurrence of similar melts found in the Namche Barwa syntaxis and in southern Tibetan gneiss domes (King et al., 2011; Zeng et al., 2012) produced by muscovite-absent melting under high pressure fluid present conditions. This first stage of melting was followed by the widespread occurrence of younger melts producing the well known high Himalayan Miocene leucogranites during the overall decompression of the GHS. Monazite ages, related to nanogranites in garnets, at 36-41 Ma in Kali Gandaki valley and at 31-28 Ma in Central and Eastern Nepal point to a quite larger time span for the occurrence of melting during prograde metamorphism in the GHS.
Stop 11
Locality : Northern end of Dana village (N 28° 31’ 27.7’’ E 83° 39’ 31,3’’)
Theme : Main Central Thrust
Going down after some turns of the road we reach Dana village and nearly 500 m before the village we can observe the sharp contact between kyanite and garnet micaschist and gneiss of the GHS with the quartzites of the LHS. This contact has been considered as the classical MCT (Fig. 29a, b) by Le Fort (1975); Vannay and Hodges (1996); Godin et al. (2001); Godin (2003); Upreti and Yoshida (2005). A medium to dark grey weathering quartzite mylonite with biotite partings crops out a little distance south of this location (Fig 29c, d). It records top to the south sense shear strain and has an fabric opening angle that is consistent with deformation temperatures of ~640 ± 50 ˚C (Larson and Godin, 2009). As mentioned previously both the definition and the position of the MCT has been rigorously debated across the Himalayan and other authors, following the definition of Searle et al. (2008), have located the structure farther to the south, near the village of Mahabar (Larson and Godin, 2009; Searle 2010). There, the structure is sometimes referred to as the MCT II or lower MCT, whereas the MCT near Dana regarded as the MCT I or upper MCT.
Stop 12
Locality: Duwari Khola, Duwarikhola village, This stop corresponds to the stop G-47 of the Field Guide book by Upreti and Yoshida (2005); N 28˚ 31’ 41.05’’, E 083˚ 39’ 04.94’’
Theme: Phyllonite (lower part) of the MCT zone
The area between the MCT and the Duwari Khola to the south is includes variable phyllonitic and mylonitic, dominantly meta-pelitic rocks of the LHS. Black phyllonitic meta-pelitic rocks are particularly well exposed along the Duwari Khola. The rocks here comprise muscovite, graphite, and biotite and include with light colored disaggregated anatexite(?) pods of quartz + feldspar. The rocks in this exposure have been subjected to top to the south shear deformation. This is recorded as well-developed C/S/C’ fabrics (Fig. 30).
Figure 30. C-S fabric in phyllites
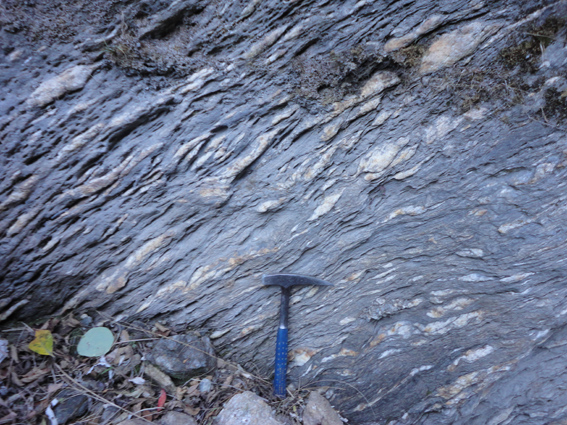
C-S fabric in phyllites with abundant deformed quartz veins. Top-to-the SW sense of shear
Stop 13
Locality: Near the bridge on the west bank of the Kali Gandaki River near Gharkholagau; 28˚ 29’ 27.4’’N, 083˚ 38’ 57.7’’E
Theme: Fagfog Formation and gabbro amphibolite
After passing south, through the village of Tatopani (the name of which means ‘hot water’ as it is located near famous riverside hot springs) quartzite of the Fagfog Formation and intercalated gabbro amphibolite crop out along the road section near to the confluence of Kali Gandaki River and Ghar Khola. Both are characterized by and foliation that dips to the north-northeast. The white to grayish colored quartzite of Fagfog Formation (Fig. 31) consists of coarse-to medium grained recrystallized quartz with fine muscovite partings and thin intercalations of phyllite. A specimen collected from this location yielded a quartz c-axis lattice preferred orientation fabric consistent with deformation at ~ 500 ± 50 ˚C (Larson and Godin, 2009). The amphibolite at this locality consists dominantly of medium-to-coarse grained plagioclase, epidote, and hornblende with minor quartz, K-feldspar and biotite. It is interpreted to have a gabbroic protolith
Stop 14
Locality: Confluence of the Beg Khola and Kali Gandaki river; 28˚ 25’ 56.08’’N, 083˚ 35’ 59.36’’E
Theme: Kuncha Formation phyllite and the lower MCT (MCT II)
The Kuncha Formation at this location consists of fine-grained gray phyllite with local intercalated metasandstone lenses. Disaggregated quartz veins are also common at this locality. The rocks here record evidence of pervasive, high shear strain. C/S/C’ fabrics are well developed throughout the outcrop (Fig. 32) and all indicate a top to the south sense of shear. (It is recommended that the outcrop is viewed along the Beg Khola; approximately perpendicular to the tectonic transport direction.) Larson and Godin (2009), using the definition of the Main Central thrust suggested by Searle et al. (2008) that the structure be mapped at the base of Cenozoic metamorphism, cooling, and pervasive ductile deformation, mapped these rocks as part of the Main Central thrust. All rocks structurally higher are pervasively deformed at relatively high temperatures (see quartz deformation temperatures above), while from this location southward the road section towards Beni Bazaar (800 m) is dominated by relatively less strained low-grade phyllitic rocks of the Kuncha Formation.
Figure 32. Deformation in phyllites
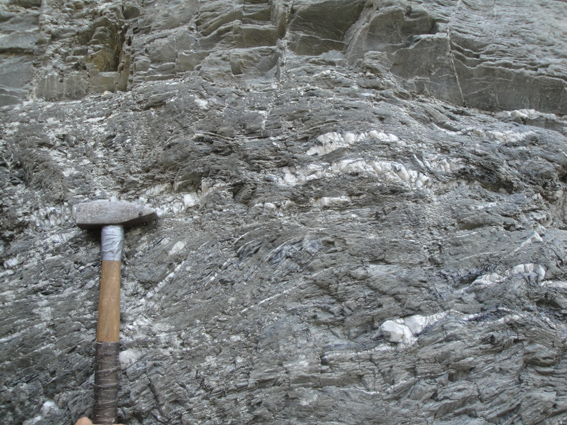
Deformation in phyllites showing sigmoidal foliation, C/S/C’ fabrics, and asymmetric, folded quartz-veins. All indicate a top-to-the SW sense of shear.