Geologic Setting
The Carpathians define a convex to the east arc that spans northeastern Austria through western Ukraine and tightens into a 150 degree bend in central Romania. The Carpathians link into a convex to the west, 180 degree, southern Balkan arc, and together the two arcuate segments of the Alpine chain define a Z-shaped system of coupled arcs. The Balkan arc links the Carpathians into the southerly, east-west trending Balkan Mountains, which terminate at the eastern margin of the Black Sea (Fig. 2). Deformation within the Carpathian-Balkan belt, like the Alpine system as a whole, is long lived, polyphase, and complex, spanning from at least the Mid-Cretaceous to recent times (e.g. Burchfiel, 1980, Royden and Burchfiel, 1986). Early (Middle Cretaceous) deformation in the Carpathian – Balkan system involved long distance nappe transport directed towards the Eurasian foreland, which, in present day coordinates, flanks the eastern side of the orogen. Nappe stacks were subsequently folded, and axial traces of this Late Cretaceous fold system run parallel to the modern arcuate trend of the orogen Carpathian – Balkan chain (Burtman, 1986 and references therein). Structural vergence in the belt is outward from the core of the northern Carpathian bend and inwards toward the core of the southern Balkan bend, consistently oriented towards the orogenic foreland and perpendicular to structural strike (Burtman, 1986; Csontos and Vörös, 2004). Cretaceous nappe emplacement has been interpreted as marking the closure of the Tethys in this region of the Alpine system (e.g. Burtamn, 1986). Alternatively, collision and accretion of the magmatic arc bearing upper plate to the southern Eurasian continental margin may not have terminated until Eocene times (Nemcock, 1998). It is generally agreed that subduction polarity was away from the Eurasian margin and toward the accreting arc, with oceanic closure involving slab roll-back toward the Eurasian margin (e.g. Burchfiel, 1980). What has been interpreted as the remnant-subducted slab has been imaged beneath the easternmost reaches of the Carpathian bend (Nemcock et al., 1998). The western flanks of the orogen are characterized by accretionary sequences (including ophiolitic assemblages) that form a continuous band around the Z-shaped Carpathian – Balkan chain (Fig. 2) and have been previously interpreted as oceanic suture (Csontos and Vörös, 2004).
Figure 2.
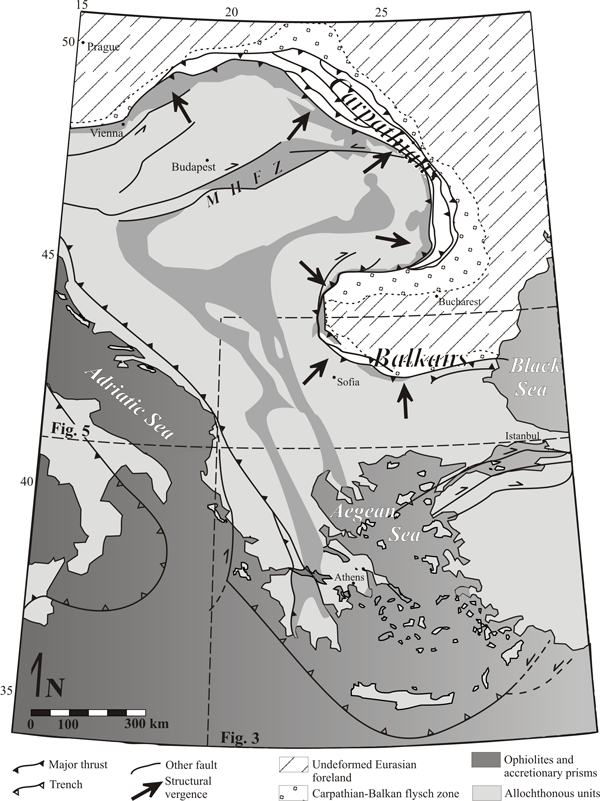
Curvature in the Carpathian – Balkan orogen and its geographic relation to the Aegean Sea region. MHFZ – Mid Hungarian fault zone. Structural vergence after Csontos and Vörös (2004). Simplified geology in the Carpathian – Balkan belt modeled after Burchfiel (1980), Horvath (1993), and Tischler et al. (2005). Extent of Balkan flysch zone after Burtman (1986), extent of ophiolites and accretionary prisms north of the fortieth parallel after Tischler et al. (2008), and simplified from van Hinsbergen et al. (2005) for Greece. Extent of ophiolitic terranes in the Anatolian Structural vergence in the belt is plate not displayed.
The Eastern Mediterranean Inclination Anomaly (EMIA)
Paleomagnetic inclination data obtained from Late Eocenee to Miocene rocks within the greater north Aegean region reveal consistently shallow inclinations with respect to a stable Europe, indicating that the region has undergone geologically recent northward displacement. We review paleomagnetic data initially compiled by Beck et al. (2000). Paleomagnetic inclinations for the Eastern Mediterranean region are measured relative to expected values as calculated based on the European apparent polar wander path (APW) of Besse and Courtillot (1991) by Beck et al. (2000). The difference between observed and calculated values yields the amount of inclination shallowing (ΔI; listed in Table 1 and displayed relatively in Fig. 3.)
All paleomagnetic results compiled herein were obtained from igneous rocks, thus avoiding likely inaccuracies associated with retrieval of inclination data from sedimentary rocks (e.g. Krijgsman, W., and Tauxe, 2004). Following the reasoning of Beck and Schermer (1994), the EMIA must be attributable to either (1) errors in the applied APW path of Besse and Courtillot (1991), (2) irregularities within dipole of the Tertiary geomagnetic field of the Aegean region, (3) consistent procedural errors in sampling and/or laboratory analysis, or (4) northward directed crustal mobility of the Aegean region with respect to stable Europe. Beck and Schermer (1994) recalculated expected inclination for the Aegean region from all available APW paths and obtained results consistent with those calculated from the path of Besse and Courtillot (1991), concluding that the problem did not lie in the European APWP. Westphal (1993) suggested that the EMIA records a large (18°) and abrupt shift in the geomagnetic dipole. As noted by Beck and Schermer (1994), however, such a shift should be recorded in contemporaneous rocks worldwide, which is not the case. For example, no such shift is recorded in the well-constrained APW paths for North America. The extended time period and localized scale of the EMIA argue against its being attributable to geomagnetic irregularities such as dipole shifts or non-dipole components. Finally, the significant number of studies that record the anomaly negate the possibility of consistent procedural errors producing false results. We conclude that the EMIA provides a true record of northward translation of crust underlying the Eastern Mediterranean region.
Early Miocene volcanic rocks from the island of Lesbos (site 6) have been the subject of several paleomagnetic studies, all of which yielded consistent results. These rocks record an average ∆I value of -5.1, corresponding to ca. 500 km northward displacement (Beck et al., 2000). Lesbos volcanic rocks may, however, have erupted after the onset of northward translation and hence may not record its full extent; we accept 500 km as a minimum value for total possible northward displacement. A plot of ∆I versus average age can be used to (1) estimate the full extent of inclination shallowing and northward mobility and (2) constrain the timing of displacement (Fig. 4). Our line of best fit intersects the x-axis (i.e. modern latitude) at 3 Ma, corresponding with the age Volos volcanics (Site 1), the only locality for which inclination shallowing was not recorded. We therefore accept 3 Ma as an estimate for the end of northward displacement. Inclination shallowing in 30 to 35 Ma rocks is consistent with 1000 km of northward displacement. Assuming that this value of 1000 km represents the total amount of northward displacement of the Eastern Mediterranean region, and that translation began around 33 Ma and ended at 3 Ma, a moderate translation rate of just over 3 cm∙yr-1 is implied.
Figure 3.
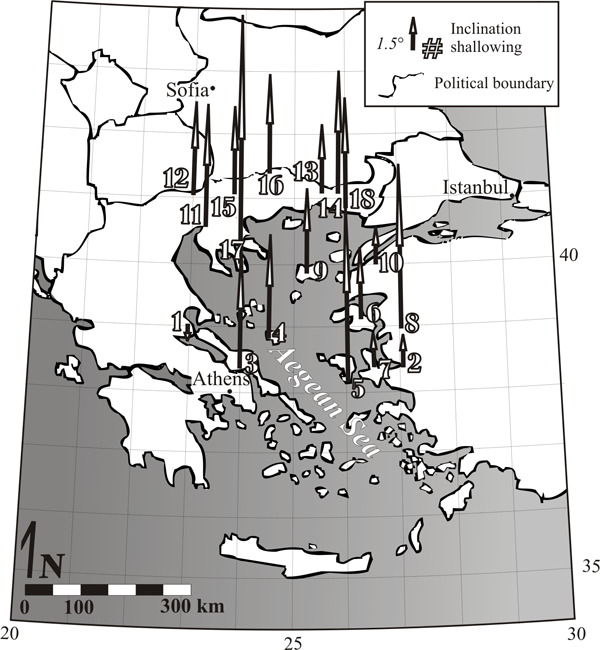
Scaled arrows represent degrees latitude of northward displacement within the Aegean region corresponding to calculated ΔI values, as listed in Table 1. Sites are numbered youngest (1) to oldest (18).
Figure 4.
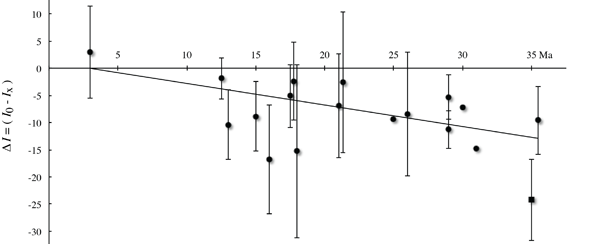
Plot of ∆I versus average age estimates for sampled sites corresponding to date presented in Table 1, modified from Beck et al. (2000). Error bars are 95% confidence values; incalculable where not shown. The solid line of best fit, from which we estimate the rate and end time of northward displacement, excludes the outlying Chalkadiki plutonics (Site 17; plotted as a square).
The Carpathian – Balkan belt: paleomagnetic rotations and the orocline hypothesis
Interpretation of the arcuate, Z-shaped geometry of the Carpathian – Balkan chain as a product of oroclinal bending of a formerly linear orogen is suggested by the perpendicularity of structural vergence to local strike. In other words, structural vergence varies as a function of strike. Primary bend models that suggest terrane accretion driven by roll-back into a pre-existing embayment predict syn-accretionary strike-slip motion along its outer flanks, for which there is no supporting evidence. In addition, regional palinspastic restoration of crustal shortening within the Carpathian – Balkan fold and thrust belt is only possible after restoration of the orogen to a linear geometry. Not doing so implies an excess of line length around the Carpathian bend, requiring all nappes to restore to a common origin, and a line length deficit around the Balkan bend, requiring thrust nappes to have been originally discontinuous. The continuity of accreted terranes around both bends further supports a secondary, oroclinal origin for the bends. Models of terrane accretion to an arcuate European continental margin predict significant differences in length between the shorter accreting arcs and the much longer continental margin. There is, however, no indication of syn-accretionary strike parallel extension within the terranes, including ophiolites, which are continuous around the Carpathian – Balkan chain. The most commonly accepted test for the oroclinal nature of arcuate orogens involves plotting paleomagnetic declination against structural strike in order to determine if changes in structural strike resulted from rotations relative to the magnetic pole (Eldredge et al. 1985). Application of the test requires declination data of well constrained age from around the entire arcuate orogenic belt. Paleomagnetic declination data for the Carpathian-Balkan region are of limited quantity and poor quality (Table 2). Data are: commonly from rocks with broad age limits; supplied with inadequate coordinate data are; characterized by error values larger than reported rotations; and/or lacking discussion of standard methods and tests used to control paleomagnetic data accuracy, e.g. the fold test. As a result, paleomagnetic data from the Carpathian – Balkan belt are not abundant or well constrained enough for application of the orocline test. There are, however, sufficient data to enable us to test for, and constrain the timing of, rotations of the Carpathian chain. In our compilation of available paleomagnetic data, we rejected data characterized by the problems outlined above, and excluded data points for which reference poles were not available. We are left with a data set comparable to those previously compiled by Dupont-Nivet et al. (2005) and (van Hinsbergen, 2008) (Fig. 5).
Figure 5.
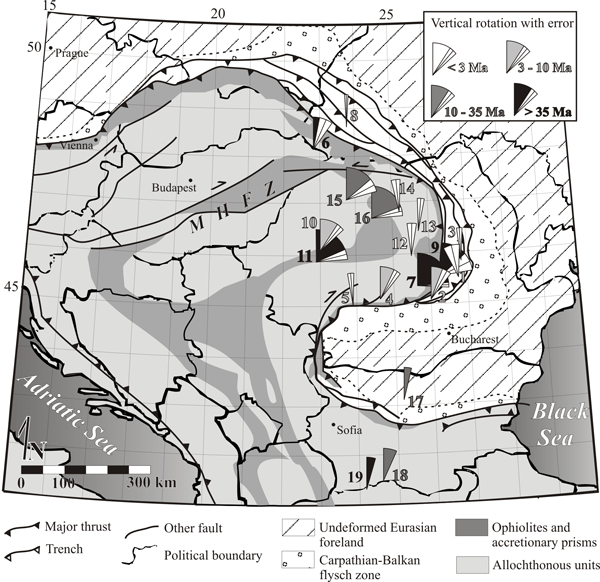
Vertical rotation data from the compilations of Dupont-Nivet et al. (2005) and van Hinsbergen (2008) show a general pattern of increasing rotation with increasing age. Site numbers correspond to those listed in Table 2. Note (1) sites with small rotations and small errors (5, 8, 12-14) and (2) error range is not plotted where not included or accurately inferable from data source (sites 17-19).
The pattern that emerges in the Carpathians is consistent with the arcuate geometry of the chain being the result of significant rotations about a vertical axis (some > 90°). In addition, the magnitudes of rotation increase with increasing magnetization age; 35+ Ma magnetizations yield the largest rotations while younger rocks yield smaller rotations. Sampling is biased toward the southern limb of the bend, for which exclusively clockwise rotations are recorded, consistent with the expected sense of rotation should the arcuate nature of the Carpathians have resulted from buckling of a previously linear orogen. In the Balkan region, data are sparse and assessments of data error are lacking. Reported rotations are small, variable, and do not provide a test of the origin of the Balkan segment of the orogen.
A Geometric model for eastern Mediterranean crustal displacement and oroclinal buckling in the Carpathian – Balkan belt
We have established that (1) the arcuate Carpathian – Balkan segment of the Alpine orogenic belt likely originated as a linear orogen which was subsequently buckled to yield a Z-shaped geometry oroclinal pair, (2) orocline formation probably occurred after 35 Ma, and (3) the EMIA is attributable to a minimum 500, but more likely 1000 km of northward translation of the Eastern Mediterranean region between 33 and 3 Ma. Hence, oroclinal bending of the Carpathian – Balkan orogen was coeval with northward translation of the eastern Mediterranean, suggesting that the two may be related. Here we provide a simple geometric test of a model in which buckling of the Carpathian – Balkan chain is explained as a result of northward translation of the Eastern Mediterranean region.
Figure 6.
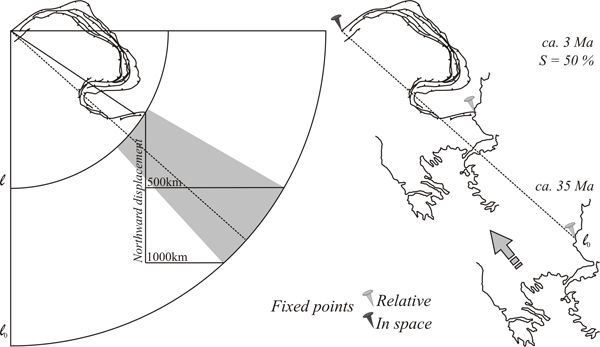
Geometric model showing 50% shortening of the Carpathian-Balkan orogen with a fixed northwest corner reveals the necessity for a component of latitudinal displacement of the Aegean. Length of originally linear orogen (l0) = 2100 km; current length of orogen (l) measured linearly from northeast to southwest endpoint = 1050 km. The shaded region represents possible pathways of a point marking the southeastern end of the orogen starting along an arc of radius l0 between 500 km and 1000 km vertical distance from its current location. The dashed line illustrates one potential geometry for the originally linear orogen.
We assume (1) an originally linear Carpathian – Balkan orogen with a northeastern end point fixed to autochthonous Europe just east of Vienna, Austria, and a southeastern endpoint fixed to the Eastern Mediterranean crustal block where the Balkans meet the western limit of the Black Sea, and (2) that a rigidly behaving Eastern Mediterranean crustal block originated between 500 and 1000 km south of its present-day location. The strike-parallel line length of the Carpathian – Balkan chain, measured from pinning point to pinning point (l0) is 2100 km, contrasting with the 1050 km linear distance between the two points measured today (lf); 50% shortening is therefore required in order to explain the bends as a product of an originally linear orogen. Our model demonstrates that 500 to 1000 km northward displacement of the eastern Mediterranean can be accommodated by 50% shortening of an originally Carpathian – Balkan orogen if (1) the orogen had an initial northwest-southeast strike (310-330°) and (2) northward displacement of the eastern Mediterranean was coupled with a westward component of latitudinal displacement (Fig. 6).
Net displacement of the eastern Mediterranean is equivalent to the 1050 km line length difference between the initial linear and final buckled Carpathian – Balkan belt. Assuming the same 30 My time window for displacement, a recalculated required rate of translation remains a reasonable 3.5 cm∙yr-1. The perfect linearity of an unbuckled Carpathian – Balkan orogen depicted in this idealized model presents an end-member extreme. A gentle curvature inherent in the belt may have facilitated oroclinal buckling and enabled its achievement through a lesser extent of total displacement within the orogen and of the impeding Eastern Mediterranean region. Younging of the youngest deformation toward the SE in the Outer Carpathians (e.g Sperner et al., 2002; Zattin et al., 2011) may indicate that oroclinal buckling originated to the northwest and progressed to the southeast. As with most documented oroclines, there is little evidence for regional strain associated with buckling within the Carpathian – Balkan system, suggesting that buckling must have been accommodated along existing planes of weakness (Johnston, 2001, Weil et al., 2000, Weil & Sussman, 2004, Shaw et al., 2012).
Figure 7.
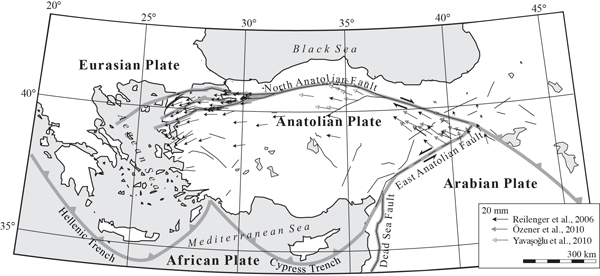
Tectonic setting for the Turkey and surrounding regions. GPS vector data along the North Anatolian fault zone show current westward motion (with a component of anticlockwise rotation) of the Anatolian plate. Thin black lines are active faults; thick grey lines are plate boundaries. Redrawn from Tartar et al. (2012) figures 1 and 2.
A latitude-parallel component of eastern Mediterranean displacement would not be visible in the paleomagnetic record, but is consistent with observed and ongoing westward displacement of the Anatolian block (Turkey). Westward directed tectonic escape of the Anatolian block out of the Arabian – Eurasian collision zone likely began in the mid-Miocene (Atzemoglou et al., 1994), and continues into the present day at an average rate of 2 cm∙yr-1 (Fig. 7) (Tartar et al., 2012). Our model thus suggests that the Z-shaped geometry of the Carpathian – Balkan belt is explained as a result of westward tectonic escape of the Aegean – Anatolian region out of the Arabian – Eurasian collision zone coupled with a component of northward translation. Palinspastic restoration of the Carpathian – Balkan oroclines therefore restores Anatolia 500 to 1000 km to the east, and confirms that westward tectonic escape of Anatolia has been ongoing since at least the mid-Miocene, as suggested by Atzemoglou (1994). As the Arabian – Eurasian collision and related tectonic escape is ongoing, further study of the Carpathian – Balkan belt in the context of an oroclinal model may aid in determining how such large-scale features are accommodated within the surrounding lithosphere and at depth.